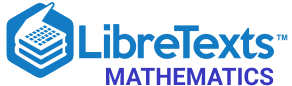
- school Campus Bookshelves
- menu_book Bookshelves
- perm_media Learning Objects
- login Login
- how_to_reg Request Instructor Account
- hub Instructor Commons
- Download Page (PDF)
- Download Full Book (PDF)
- Periodic Table
- Physics Constants
- Scientific Calculator
- Reference & Cite
- Tools expand_more
- Readability
selected template will load here
This action is not available.
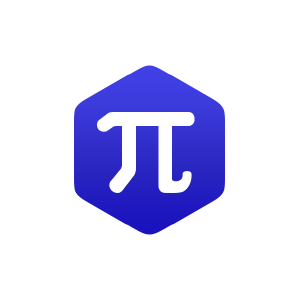
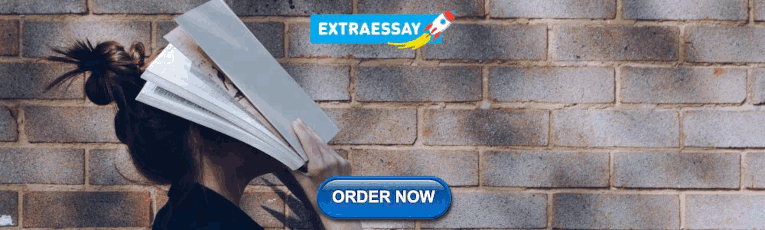
6.2.3: Using Metric Conversions to Solve Problems
- Last updated
- Save as PDF
- Page ID 62187

- The NROC Project
Learning Objectives
- Solve application problems involving metric units of length, mass, and volume.
Introduction
Learning how to solve real-world problems using metric conversions is as important as learning how to do the conversions themselves. Mathematicians, scientists, nurses, and even athletes are often confronted with situations where they are presented with information using metric measurements, and must then make informed decisions based on that data.
To solve these problems effectively, you need to understand the context of a problem, perform conversions, and then check the reasonableness of your answer. Do all three of these steps and you will succeed in whatever measurement system you find yourself using.
Understanding Context and Performing Conversions
The first step in solving any real-world problem is to understand its context. This will help you figure out what kinds of solutions are reasonable (and the problem itself may give you clues about what types of conversions are necessary). Here is an example.
In the Summer Olympic Games, athletes compete in races of the following lengths: 100 meters, 200 meters, 400 meters, 800 meters, 1500 meters, 5000 meters and 10,000 meters. If a runner were to run in all these races, how many kilometers would he run?
The runner would run 18 kilometers.
This may not be likely to happen (a runner would have to be quite an athlete to compete in all of these races) but it is an interesting question to consider. The problem required you to find the total distance that the runner would run (in kilometers). The example showed how to add the distances, in meters, and then convert that number to kilometers.
An example with a different context, but still requiring conversions, is shown below.
One bottle holds 295 deciliters while another one holds 28,000 milliliters. What is the difference in capacity between the two bottles?
There is a difference in capacity of 1.5 liters between the two bottles.
This problem asked for the difference between two quantities. The easiest way to find this is to convert one quantity so that both quantities are measured in the same unit, and then subtract one from the other.
One boxer weighs in at 85 kilograms. He is 80 dekagrams heavier than his opponent. How much does his opponent weigh?
- \(\ 5 \text { kilograms }\)
- \(\ 84.2 \text { kilograms }\)
- \(\ 84.92 \text { kilograms }\)
- \(\ 85.8 \text { kilograms }\)
- Incorrect. Look at the unit labels. The boxer is 80 dekagrams heavier, not 80 kilograms heavier. The correct answer is 84.2 kilograms.
- Correct. \(\ 80 \text { dekagrams }=0.8 \text { kilograms }\), and \(\ 85-0.8=84.2\).
- Incorrect. This would have been true if the difference in weight was 8 dekagrams, not 80 dekagrams. The correct answer is 84.2 kilograms.
- Incorrect. The first boxer is 80 dekagrams heavier , not lighter than his opponent. This question asks for the opponent’s weight. The correct answer is 84.2 kilograms.
Checking your Conversions
Sometimes it is a good idea to check your conversions using a second method. This usually helps you catch any errors that you may make, such as using the wrong unit fractions or moving the decimal point the wrong way.
A two-liter bottle contains 87 centiliters of oil and 4.1 deciliters of water. How much more liquid is needed to fill the bottle?
The amount of liquid needed to fill the bottle is 0.72 liter.
Having come up with the answer, you could also check your conversions using the quicker “move the decimal” method, shown below.
The amount of liquid needed to fill the bottle is 0.72 liters.
The initial answer checks out. 0.72 liter of liquid is needed to fill the bottle. Checking one conversion with another method is a good practice for catching any errors in scale.
Understanding the context of real-life application problems is important. Look for words within the problem that help you identify what operations are needed, and then apply the correct unit conversions. Checking your final answer by using another conversion method (such as the “move the decimal” method, if you have used the factor label method to solve the problem) can cut down on errors in your calculations.
Measurement Worksheets
Welcome to the measurement worksheets page at Math-Drills.com where you can measure up, measure down or measure all around! This page includes Measurement worksheets for length, area, angles, volume, capacity, mass, time and temperature in Metric, U.S. and Imperial units.
Measurement concepts and skills give students the ability to perform tasks related to everyday life. Length, area, volume, capacity, mass, time and temperature are measurement concepts that people are exposed to everyday. Students begin using non-standard units such as their own height and progress to using standard measurement units. Being able to recognize and use for comparison, common measurement units such as the metre or foot, allows students to use their estimation skills to help them solve problems in measurement. Measurement tools enable students to learn hands-on and develop a deeper understanding of measurement concepts.
Most Popular Measurement Worksheets this Week
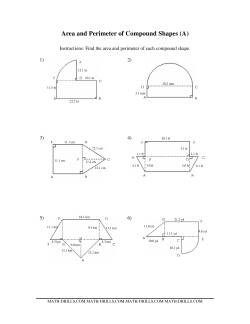
Reading and Converting Temperature Worksheets
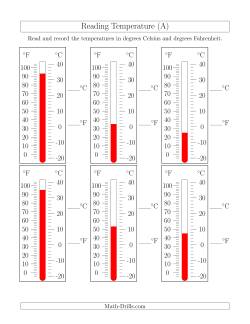
Reading temperatures from a thermometer is one of those everyday skills that is transferable to other situations. Sure, students could just ask their smart device about the temperature, but that only tells them the temperature at the nearest weather station, not where they're standing. Wouldn't it be more exciting to look at a thermometer in their own yard? And what if they are working at a solar power farm (or elsewhere) in the future and have other meters and gauges to read? Their skill in reading thermometers will help introduce them to negative numbers and be useful for reading other types of meters and gauges in the future.
- Reading Temperatures From Thermometers Reading Temperatures from a Thermometer Reading Temperatures from Circular Thermometers (Fahrenheit Dominant) Reading Temperatures from Circular Thermometers (Celsius Dominant)
Millions of people live near the U.S./Canada border, so it is inevitable that once in a while, those people are going to hear or see the temperature in a less familiar unit. Perhaps traveling to another country has one baffled about the forecast. Having a knowledge of some simple equivalents (like the freezing point is 0 degrees Celsius and 32 degrees Fahrenheit) and the ability to convert between C and F will not only help in determining the temperature in a familiar form, but will also help students build math skills that are useful in other situations. The temperature conversion guide gives a summary of the formulas used and some whole number equivalents to learn. There are several practice sheets for converting from °C to °F, from °F to °C, and a couple with both conversions mixed up.
- Temperature Conversion Guide Temperature Conversion Guide
- Converting Temperatures Between °C and °F Converting °C to °F (no negatives) Converting °C to °F (with negatives) Converting °F to °C (no negatives) Converting °F to °C (with negatives) Converting Between °F and °C (no negatives) Converting Between °F and °C (with negatives)
Measuring and Converting Within Measurement Systems
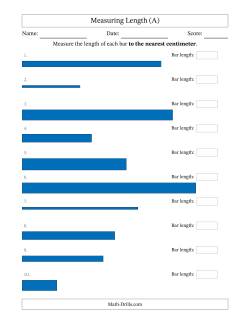
Measuring length is so much more interesting if you can send students out with rulers and have them measure items in their environment. What is the width of the textbook? the classroom? the school? Have you ever met a student who didn't enjoy using a measuring wheel (you know the one that clicks every time you've traveled a yard or a meter)? How do you know they've measured things correctly though? Well, you might need something like we've provided below. You can also compare students' measurements of the same objects to see if they got the same measurement. Let's say, you had 20 students measure the height of the doorway. You should get 20 very similar answers (unless they are the sharing type then you'll get exactly the same answers) and any different answers can be quickly identified. IMPORTANT: When printing, select "Actual Size" or your measurements may be off.
- Measuring Lengths of Bars in Centimeters and Millimeters Measuring Length in Centimeters ✎ Measuring Length in Half Centimeters ✎ Measuring Length in Millimeters ✎
- Measuring Lengths of Bars in Inches Measuring Length in Half Inches ✎ Measuring Length in Quarter Inches ✎ Measuring Length in Eighth Inches ✎ Measuring Length in Sixteenth Inches ✎
Just like regrouping when adding, sometimes it is useful to regroup when measuring. If you have too many feet, it might be easier to express your number in yards or miles, for example.
- Converting Between U.S. Length Measurements Worksheets Converting Between Inches, Feet and Yards Converting Between Feet, Yards and Miles Converting Inches, Feet, Yards and Miles
Which unit to use with mass depends a lot on the quantity and what you want to communicate. Whatever your reason for converting mass measurements, these worksheets are likely able to help you take a weight off your shoulders.
- Converting Between U.S. Mass Measurements Worksheets Converting Between Ounces and Pounds Converting Between Ounces and Pounds with Fractions Converting Between Pounds and Tons Converting Between Pounds and Tons with Fractions Converting Ounces/Pounds or Pounds/Tons Converting Ounces/Pounds or Pounds/Tons with Fractions
The liquid measurement worksheets include gills because this is the key unit that results in more fluid ounces in an Imperial gallon than in a U.S. gallon. You can learn more about gills in our liquid measurement conversion guide.
- U.S. Liquid Measurements Conversion Guide Liquid Measurements Conversion Guide (U.S.)
- Converting Between U.S. Liquid Measurements Converting Liquid Measurements (U.S.) Converting Liquid Measurements (No Gills) (U.S.) Converting Between Pints, Quarts and Gallons (U.S.)
Even though Imperial and U.S. Customary units may sound the same, they aren't always the same amount. For example, there are 3.785 litres in a U.S. gallon and 4.546 litres in an Imperial gallon. Sometimes there are also different definitions for units like the gill used in liquid measurements. In the U.S., there are 4 fluid ounces in a gill and in the Imperial System, there are 5 fluid ounces in a gill.
- Imperial (U.K.) Liquid Measurements Conversion Guide Liquid Measurements Conversion Guide (Imperial)
- Converting Between Imperial (U.K.) Liquid Measurements Converting Liquid Measurements (Imperial) Converting Liquid Measurements (No Gills) (Imperial)
Converting between Metric units is really an exercise in multiplying and dividing by powers of ten. Each of the converting worksheets in this section includes a "Conversion Line" that includes the prefixes, symbols and powers. It can be used to figure out how many "steps" are required to convert from one unit to another and what operation must be used. For example, when converting from millimeters to kilometers, students would determine that it takes six steps to the left in the direction of the division sign to get from milli to kilo on the conversion line. Depending on which method they are taught, this could involve dividing by 10 six times, dividing by 10 6 or "moving the decimal" six places to the left. For squared units, each step counts as 100 or 10 2 and for cubic units, each step counts as 1000 or 10 3 . For more details, please see the Metric system conversion guide.
- Converting Within the Metric System Resources Metric System Conversion Guide (U.S. Version) Metric System Conversion Lines ✎
- Converting Between Metric Length Measurements Worksheets Converting Between Millimeters and Centimeters ✎ Converting Between Centimeters and Meters ✎ Converting Between Millimeters and Meters ✎ Converting Between Millimeters, Centimeters and Meters ✎ Converting Between Meters and Kilometers ✎ Converting Between Millimeters, Centimeters, Meters, and Kilometers ✎ Converting Between Nanometers, Micrometers, Millimeters and Centimeters ✎
- Converting Between Metric Mass Measurements Worksheets Converting Between Milligrams and Grams ✎ Converting Between Grams and Kilograms ✎ Converting Between Milligrams, Grams and Kilograms ✎ Converting Between Nanograms, Micrograms, Milligrams and Grams ✎
- Converting Between Metric Volume Measurements Worksheets Converting Between Milliliters and Liters ✎ Converting Between Microliters, Milliliters, Centiliters and Liters ✎ Converting Between Milliliters, Centiliters, Liters and Kiloliters ✎ Converting Between Milliliters, Liters, and Kiloliters ✎ Converting Between Liters, Kiloliters, Megaliters and Gigaliters ✎
- Converting Between Common Metric Measurements (Mixed) Converting Between Common Metric Length, Mass and Volume Units ✎
- Converting Between Metric Area Measurements Worksheets Converting Between Square Millimeters and Square Centimeters ✎ Converting Between Square Centimeters and Square Meters ✎ Converting Between Square Millimeters, Square Centimeters and Square Meters ✎ Converting Between Square Meters, Square Hectometers and Square Kilometers ✎
The Metric or SI system uses thin spaces for thousands separators and spells metres and litres with -re rather than -er. This section is mainly for students in English Canada, however, anyone who uses spaces for thousands separators might like these worksheets. This section is very similar to the previous section except for the differences in number formats and spelling.
- Metric System Conversion Guide (SI Format: Spelling and Space-Separated Thousands) Metric System Conversion Guide (SI Version)
- Converting Between Metric Length Measurements Worksheets (SI Format: Space-Separated Thousands) Converting Between Millimetres and Centimetres (SI number format) ✎ Converting Between Centimetres and Metres (SI number format) ✎ Converting Between Millimetres and Metres (SI number format) ✎ Converting Between Millimetres, Centimetres and Metres (SI number format) ✎ Converting Between Metres and Kilometres (SI number format) ✎ Converting Between Millimetres, Centimetres, Metres, and Kilometres (SI number format) ✎ Converting Between Nanometres, Micrometres, Millimetres and Centimetres (SI number format) ✎
- Converting Between Metric Mass Measurements Worksheets (SI Format: Space-Separated Thousands) Converting Between Milligrams and Grams (SI number format) ✎ Converting Between Grams and Kilograms (SI number format) ✎ Converting Between Milligrams, Grams and Kilograms (SI number format) ✎ Converting Between Nanograms, Micrograms, Milligrams and Grams (SI number format) ✎
- Converting Between Metric Volume Measurements Worksheets (SI Format: Space-Separated Thousands) Converting Between Millilitres and Litres (SI number format) ✎ Converting Between Microlitres, Millilitres, Centilitres and Litres (SI number format) ✎ Converting Between Millilitres, Centilitres, Litres and Kilolitres (SI number format) ✎ Converting Between Millilitres, Litres, and Kilolitres (SI number format) ✎ Converting Between Litres, Kilolitres, Megalitres and Gigalitres (SI number format) ✎
- Converting Between Common Metric Measurements (Mixed) Worksheets (SI Format: Space-Separated Thousands) Converting Between Common Metric Length, Mass and Volume Units (SI number format) ✎
- Converting Between Metric Area Measurements Worksheets (SI Format: Space-Separated Thousands) Converting Between Square Millimetres and Square Centimetres (SI number format) ✎ Converting Between Square Centimetres and Square Metres (SI number format) ✎ Converting Between Square Millimetres, Square Centimetres and Square Metres (SI number format) ✎ Converting Between Square Metres, Square Hectometres and Square Kilometres (SI number format) ✎
Similar to the previous two sections; however, these worksheets use dots for thousands separators and commas for decimals. This is often found in languages other than English. Metres and litres are spelled with -re as you would find in many countries outside of the U.S.
- Metric System Conversion Guide (European Format: Period-Separated Thousands and Comma Decimals) Metric System Conversion Guide (European Format: Period-Separated Thousands and Comma Decimals)
- Converting Between Metric Length Measurements Worksheets (European Format: Period-Separated Thousands and Comma Decimals) Converting Between Millimetres and Centimetres (Euro number format) ✎ Converting Between Centimetres and Metres (Euro number format) ✎ Converting Between Millimetres and Metres (Euro number format) ✎ Converting Between Millimetres, Centimetres and Metres (Euro number format) ✎ Converting Between Metres and Kilometres (Euro number format) ✎ Converting Between Millimetres, Centimetres, Metres, and Kilometres (Euro number format) ✎ Converting Between Nanometres, Micrometres, Millimetres and Centimetres (Euro number format) ✎
- Converting Between Metric Mass Measurements Worksheets (European Format: Period-Separated Thousands and Comma Decimals) Converting Between Milligrams and Grams (Euro number format) ✎ Converting Between Grams and Kilograms (Euro number format) ✎ Converting Between Milligrams, Grams and Kilograms (Euro number format) ✎ Converting Between Nanograms, Micrograms, Milligrams and Grams (Euro number format) ✎
- Converting Between Metric Volume Measurements Worksheets (European Format: Period-Separated Thousands and Comma Decimals) Converting Between Millilitres and Litres (Euro number format) ✎ Converting Between Microlitres, Millilitres, Centilitres and Litres (Euro number format) ✎ Converting Between Millilitres, Centilitres, Litres and Kilolitres (Euro number format) ✎
- Converting Between Common Metric Measurements (Mixed) Worksheets (European Format: Period-Separated Thousands and Comma Decimals) Converting Between Common Metric Length, Mass and Volume Units (Euro number format) ✎
- Converting Between Metric Area Measurements Worksheets (European Format: Period-Separated Thousands and Comma Decimals) Converting Between Square Millimetres and Square Centimetres (Euro number format) ✎ Converting Between Square Centimetres and Square Metres (Euro number format) ✎ Converting Between Square Millimetres, Square Centimetres and Square Metres (Euro number format) ✎ Converting Between Square Metres, Square Hectometres and Square Kilometres (Euro number format) ✎
Converting Between Measurement Systems Worksheets
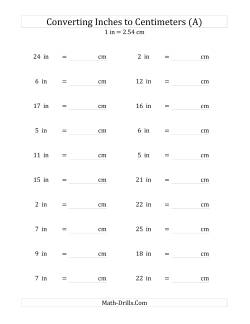
Converting between Metric and U.S. customary units can be accomplished in a number of ways and usually takes a little knowledge of fractions and/or decimals. Most commonly, students will use a formula to convert and round the values. You may like our converting inches and centimeters with rulers worksheets for students who have difficulty with manipulating the numbers and formulas and need an easier method.
- Converting Between Inches and Centimeters Converting Inches to cm (whole inches) Converting Inches to cm (to 1/2 inches) Converting Inches to cm (to 1/4 inches) Converting Inches to cm (to 1/8 inches)
- Converting Between Inches and Centimeters With a Ruler Convert Inches to Centimeters with a Ruler Convert Centimeters to Inches with a Ruler Convert Between in/cm with a Ruler
- Converting Between U.S. and Metric Length Units Converting Between U.S. Inches and Centimeters Converting Between Meters and U.S. Feet and Yards Converting Between U.S. Miles and Kilometers Converting Between U.S. Feet and Kilometers and Meters and U.S. Miles Converting Between Metric and U.S. Length Units Converting Between Metric and U.S. Length Units including ft/km and m/mi
- Converting Between U.S. and Metric Mass Units Converting Between U.S. Ounces and Grams Converting Between U.S. Pounds and Kilograms Converting Between Metric and U.S. Mass Units
- Converting Between U.S. and Metric Volume Units Converting Between Milliliters and U.S. Fluid Ounces Converting Between Liters and U.S. Cups, Pints, Quarts and Gallons Converting Between Metric and U.S. Volume Units
- Converting Between Imperial (U.K.) and Metric Mass Units Converting Between Grams and Imperial Ounces Converting Between Kilograms and Imperial Pounds and Stone Converting Between Metric and Imperial Mass Units
- Converting Between Imperial (U.K.) and Metric Volume Units Converting Between Milliliters and Imperial Fluid Ounces Converting Between Liters and Imperial Cups, Pints, Quarts and Gallons Converting Between Metric and Imperial Volume Units
- Converting Worksheets For U.S. Nurses Converting mass measurements used in Nursing Converting volume measurements used in Nursing
Measuring Angles, Rectangles and Triangles
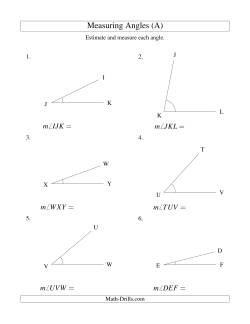
If they are available, full round protractors help students to recognize that measuring angles is the same as measuring sections of a circle. They also makes it much easier and precise to measure reflex angles.
- Measuring Angles Measuring Angles from 5° to 90° Measuring Angles from 5° to 175° Measuring Angles from 90° to 175° Measuring Angles from 185° to 355° Measuring Angles from 5° to 355°
Rectangles are fairly straight-forward polygons to measure since it is easy to find rectangles that use whole numbers. Rectangles are generally used when students first learn about perimeter and area and it is an opportune time to teach students that units are an essential part of any measurement. Without units, numbers are meaningless. Get your students into the habit of expressing all of their measurements with the correct units before they learn how to measure other polygons. Especially make sure they know that area is always expressed with squared units.
If a student is just starting to learn about perimeter and area, a few hands-on activities to learn the concepts is a good idea. Have them use square tiles to cover an area, have them paint a piece of paper and see how much paint is required. Create rectangles with straws and pipe cleaners and fill with square tiles to differentiate between perimeter and area. See if there are differently shaped rectangles that will hold the same number of square tiles.
- Perimeter and Area of Rectangles Worksheets Calculate the Area and Perimeter of Rectangles from Side Measurements (Smaller Whole Numbers) Calculate the Area and Perimeter of Rectangles from Side Measurements (Larger Whole Numbers) Calculate the Area and Perimeter of Rectangles from Side Measurements (Decimal Numbers) Area of Rectangles (grid form)
- Perimeter and Area of Rectangles Worksheets (Retro) (Retro) Rectangles (whole numbers; range 1-9) (Retro) Rectangles (whole numbers; range 5-20) (Retro) Rectangles (whole numbers; range 10-99) (Retro) Rectangles (1 decimal place; range 1-9) (Retro) Rectangles (1 decimal place; range 5-20) (Retro) Rectangles (1 decimal place; range 10-99)
- Calculating Other Rectangle Measurements Using Perimeter and Area Calculate the Side and Area Measurements of Rectangles from Perimeter and Side (Smaller Whole Numbers) Calculate the Side and Area Measurements of Rectangles from Perimeter and Side (Larger Whole Numbers) Calculate the Side and Area Measurements of Rectangles from Perimeter and Side (Decimal Numbers) Calculate the Side and Perimeter Measurements of Rectangles from Area and Side (Smaller Whole Numbers) Calculate the Side and Perimeter of Rectangles from Area and Side Measurements (Larger Whole Numbers) Calculate the Side and Perimeter of Rectangles from Area and Side Measurements (Decimal Numbers) Calculate the Side Measurements of Rectangles from Perimeter and Area (Smaller Whole Numbers) Calculate the Side Measurements of Rectangles from Perimeter and Area (Larger Whole Numbers) Calculate the Side Measurements of Rectangles from Perimeter and Area (Decimal Numbers) Calculate Various Rectangle Measurements (Smaller Whole Numbers) Calculate Various Rectangle Measurements (Larger Whole Numbers) Calculate Various Rectangle Measurements ( Decimal Numbers)
If you want students to understand the triangle area formula, you might want to study parallelograms and rectangles first. Once students get how area is calculated for rectangles and parallelograms, they simply need to cut parallelograms and rectangles in half diagonally to get related triangles. They should quickly see that the area of a triangle is simply half of the area of the related quadrilateral.
- Area and Perimeter of Triangles Worksheets Calculate the Area and Perimeter of Acute Triangles Calculate the Area and Perimeter of Acute Triangles (Rotated Triangles) Calculate the Area and Perimeter of Right Triangles Calculate the Area and Perimeter of Right Triangles (Rotated Triangles) Calculate the Area and Perimeter of Obtuse Triangles Calculate the Area and Perimeter of Obtuse Triangles (Rotated Triangles) Calculate the Area and Perimeter of Acute and Right Triangles Calculate the Area and Perimeter of Acute and Right Triangles (Rotated Triangles) Calculate the Area and Perimeter of Various Triangles Calculate the Area and Perimeter of Various Triangles (Rotated Triangles)
- Area and Perimeter of Triangles Worksheets (Retro) (Retro) Triangles (1 decimal place; range 1-5) (Retro) Triangles (1 decimal place; range 1-9) (Retro) Triangles (1 decimal place; range 5-20) (Retro) Triangles (1 decimal place; range 10-99)
- Calculating Other Triangle Measurements (Angles, Heights, Bases) Calculate Angles of a Triangle Given Other Angle(s) Calculate the Perimeter and Height Measurements of Triangles Calculate the Area and Height Measurements of Right Triangles Calculate the Base and Height Measurements of Triangles Calculate Various Measurements of Triangles Calculate the Perimeter and Area from Side Measurements of Triangles (Heron's Formula)
Measuring Other Polygons
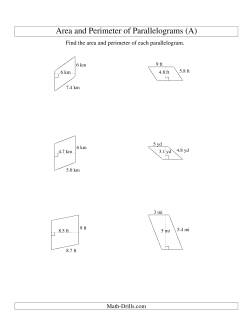
- Area and Perimeter of Parallelograms Worksheets Parallelograms (whole number base; range 1-9) Parallelograms (1 decimal place; range 1-5) Parallelograms (1 decimal place; range 1-9) Parallelograms (1 decimal place; range 5-20) Parallelograms (1 decimal place; range 10-99)
- Area and Perimeter of Trapezoids Worksheets Calculate the Area and Perimeter of Trapezoids (Smaller Numbers) Calculate the Area and Perimeter of Trapezoids (Larger Numbers) Calculate the Area and Perimeter of Trapezoids (Even Larger Numbers) Calculate the Area and Perimeter of Trapezoids (Larger Still Numbers) Calculate the Area and Perimeter of Isosceles Trapezoids Calculate the Area and Perimeter of Right Trapezoids Calculate the Area and Perimeter of Scalene Trapezoids
- Calculating Other Trapezoid Measurements Worksheets Calculate Bases and Areas of Trapezoids Calculate Bases and the Heights of Trapezoids Calculate Bases and Perimeters of Trapezoids Calculate Bases and Sides of Trapezoids Mixed Trapezoids Questions
- Area and Perimeter of Trapeziums Worksheets (U.K. Format: Name of Shape) Calculate the Area and Perimeter of Trapeziums (Smaller Numbers) Calculate the Area and Perimeter of Trapeziums (Larger Numbers) Calculate the Area and Perimeter of Trapeziums (Even Larger Numbers) Calculate the Area and Perimeter of Trapeziums (Larger Still Numbers) Calculate the Area and Perimeter of Isosceles Trapeziums Calculate the Area and Perimeter of Right Trapeziums Calculate the Area and Perimeter of Scalene Trapeziums
- Calculating Other Trapezium Measurements Worksheets (U.K. Format: Name of Shape) Calculate Bases and Areas of Trapeziums Calculate Bases and Heights of Trapeziums Calculate Bases and Perimeters of Trapeziums Calculate Bases and Sides of Trapeziums Mixed Trapeziums Questions
The shapes are mixed up on the worksheets in this section. These area and perimeter worksheets would be best suited to students who have mastered finding the areas of triangles, rectangles, parallelograms, and trapezoids. For students who need an extra challenge, give them the compound shapes worksheet, but make sure they know how to find the area and circumference of a circle first.
- Calculating The Area And Perimeter Of Various Shapes Various Shapes (1 decimal place; range 1-9) Various Shapes (1 decimal place; range 5-20) Various Shapes (1 decimal place; range 10-99) Area and Perimeter of Measured Compound Shapes
Measuring Circles
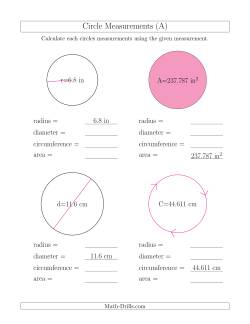
Radius, diameter, circumference and area are all related measurements; you only need one of them to find the remaining measurements. Diameter and radius are the simplest ones because the diameter of a circle is twice the radius and, conversely, the radius is half the diameter. To calculate between radius/diameter and circumference/area, you need to use π (pi). Depending on your accessibility to calculators or computers, you may use many digits of pi in the calculation or just a few. Often, people without calculators use an estimate of pi (3 or 3.14). Just for fun we made a worksheet with pi to 100,000 decimal places. The calculations on the worksheets below use a fairly precise version of pi; you may have to adjust the answers if you use more rounded versions of pi.
- Pi to 100K Decimal Places Reference Pi to 100K Decimal Places
- Circle Measurements Worksheets (Area, Circumference, Diameter, Radius) Calculate All Circle Measurements Calculate the Area & Circumference from Radius Calculate the Area & Circumference from Diameter Calculate the Area & Circumference Calculate the Radius & Diameter from Area Calculate the Radius & Diameter from Circumference Calculate the Radius & Diameter Calculate the Area & Circumference (old)
- Calculating Arc Lengths Calculating Arc Length from Circumference Calculating Arc Length from Radius Calculating Arc Length from Diameter Calculating Arc Length from Radius or Diameter Calculating Arc Length from Circumference, Radius or Diameter
- Calculating Arc Angles Calculating Arc Angle from Circumference Calculating Arc Angle from Radius Calculating Arc Angle from Diameter Calculating Arc Angle from Radius or Diameter Calculating Arc Angle from Circumference, Radius or Diameter
- Calculating Arc Lengths and Angles Calculating Arc Length or Angle from Circumference Calculating Arc Length or Angle from Radius Calculating Arc Length or Angle from Diameter Calculating Arc Length or Angle from Radius or Diameter Calculating Arc Length or Angle from Circumference, Radius or Diameter
Measuring Three-Dimensional Forms
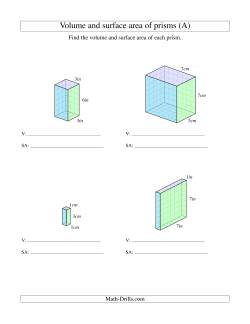
- Volume and Surface Area of Rectangular Prisms Worksheets Rectangular Prisms Volume and Surface Area ( Whole Numbers ) Rectangular Prisms Volume and Surface Area ( Decimal Numbers )
- Volume and Surface Area of Triangular Prisms Worksheets Triangular Prisms Volume and Surface Area ( Black and White ) Triangular Prisms Volume and Surface Area ( Color )
- Volume and Surface Area of Cylinders Worksheets Cylinders Volume and Surface Area Cylinders Volume and Surface Area ( Small Measurements )
- Volume and Surface Area of Other Prisms Worksheets Parallelogram Prisms Volume and Surface Area Trapezoid Prisms Volume and Surface Area Composite-Base Prisms Volume and Surface Area Mixed Right Prisms Volume and Surface Area
- Volume and Surface Area of Spheres Worksheets Volume and Surface Area of Spheres (1 Decimal Place) Volume and Surface Area of Spheres (2 Decimal Places) Volume and Surface Area of Spheres (Large Numbers)
- Volume and Surface Area of Cones Worksheets Volume and Surface Area of Cones (Whole Numbers) Volume and Surface Area of Cones (1 Decimal Place) Volume and Surface Area of Cones (2 Decimal Places) Volume and Surface Area of Cones (Large Numbers)
- Volume and Surface Area of Conical Frustums Worksheets Volume and Surface Area of Conincal Frustums (Whole Numbers) Volume and Surface Area of Conincal Frustums (1 Decimal Place) Volume and Surface Area of Conincal Frustums (2 Decimal Places) Volume and Surface Area of Conincal Frustums (Large Numbers)
Copyright © 2005-2024 Math-Drills.com You may use the math worksheets on this website according to our Terms of Use to help students learn math.
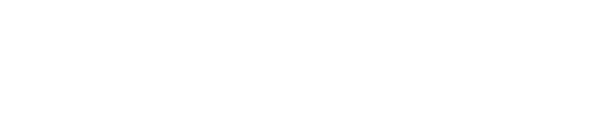
Or search by topic
Number and algebra
- The Number System and Place Value
- Calculations and Numerical Methods
- Fractions, Decimals, Percentages, Ratio and Proportion
- Properties of Numbers
- Patterns, Sequences and Structure
- Algebraic expressions, equations and formulae
- Coordinates, Functions and Graphs
Geometry and measure
- Angles, Polygons, and Geometrical Proof
- 3D Geometry, Shape and Space
- Measuring and calculating with units
- Transformations and constructions
- Pythagoras and Trigonometry
- Vectors and Matrices
Probability and statistics
- Handling, Processing and Representing Data
- Probability
Working mathematically
- Thinking mathematically
- Mathematical mindsets
- Cross-curricular contexts
- Physical and digital manipulatives
For younger learners
- Early Years Foundation Stage
Advanced mathematics
- Decision Mathematics and Combinatorics
- Advanced Probability and Statistics
Measurement
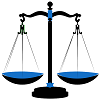
Estimating, Comparing, Measuring KS1
This collection of KS1 tasks combines the skills of estimating, comparing and measuring.
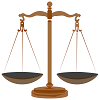
Estimating, Comparing, Measuring KS2
This collection of KS2 tasks combines the skills of estimating, comparing and measuring.
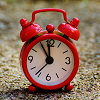
The KS1 activities in this collection are all related to time.
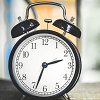
The KS2 activities in this collection are all related to time.
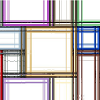
Area and Perimeter KS2
These resources introduce and explore the concepts of area and perimeter.
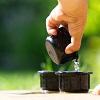
Volume and Capacity KS2
These resources introduce and explore the concepts of volume and capacity.
Find out how Siemens has benefited from our services
of the DAX 30 companies work with us
- Solutions Use cases Answer to your HR questions. smartData Market Intelligence Access to the world’s largest labor market database to tune your business and HR. smartPlan Future Workforce Planning Design your future workforce & uncover skills risks and gaps. smartPeople Skills Fulfillment Discover your internal skills and build a future-fit workforce.
- Podcasts & interviews
- ROI calculator
- HR Glossary
See why 100+ companies choose HRForecast.
- Book a demo
How to assess problem-solving skills
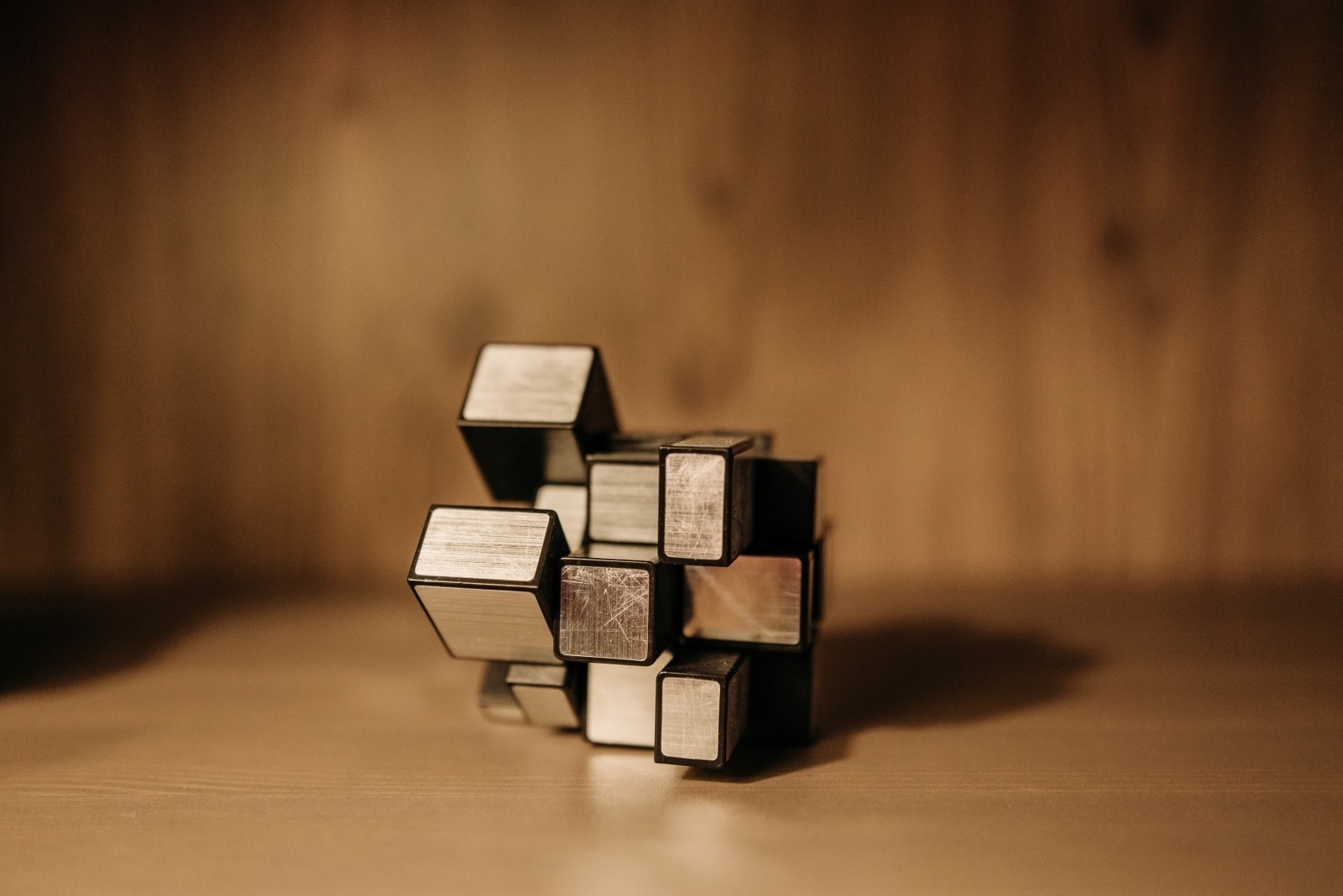
Human beings have been fascinated and motivated by problem-solving for as long as time. Let’s start with the classic ancient legend of Oedipus. The Sphinx aggressively addressed anyone who dared to enter Thebes by posing a riddle. If the traveler failed to answer the riddle correctly, the result was death. However, the Sphinx would be destroyed when the answer was finally correct.
Alas, along came Oedipus. He answered correctly. He unlocked this complex riddle and killed the Sphinx.
However, rationality was hardly defined at that time. Today, though, most people assume that it simply takes raw intelligence to be a great problem solver. However, it’s not the only crucial element.
Introduction to key problem-solving skills
You’ve surely noticed that many of the skills listed in the problem-solving process are repeated. This is because having these abilities and talents are so crucial to the entire course of getting a problem solved. Let’s look at some key problem-solving skills that are essential in the workplace.
Communication, listening, and customer service skills
In all the stages of problem-solving, you need to listen and engage to understand what the problem is and come to a conclusion as to what the solution may be. Another challenge is being able to communicate effectively so that people understand what you’re saying. It further rolls into interpersonal communication and customer service skills, which really are all about listening and responding appropriately.
Data analysis, research, and topic understanding skills
To produce the best solutions, employees must be able to understand the problem thoroughly. This is possible when the workforce studies the topic and the process correctly. In the workplace, this knowledge comes from years of relevant experience.
Dependability, believability, trustworthiness, and follow-through
To make change happen and take the following steps towards problem-solving, the qualities of dependability, trustworthiness, and diligence are a must. For example, if a person is known for not keeping their word, laziness, and committing blunders, that is not someone you’ll depend on when they provide you with a solution, will you?
Leadership, team-building, and decision-making
A true leader can learn and grow from the problems that arise in their jobs and utilize each challenge to hone their leadership skills. Problem-solving is an important skill for leaders who want to eliminate challenges that can otherwise hinder their people’s or their business’ growth. Let’s take a look at some statistics that prove just how important these skills are:
A Harvard Business Review study states that of all the skills that influence a leader’s success, problem-solving ranked third out of 16.
According to a survey by Goremotely.net, only 10% of CEOs are leaders who guide staff by example .
Another study at Havard Business Review found a direct link between teambuilding as a social activity and employee motivation.
Are you looking for a holistic way to develop leaders in your workplace?
Numerous skills and attributes define a successful one from a rookie when it comes to leaders. Our leadership development plan (with examples!) can help HR leaders identify potential leaders that are in sync with your company’s future goals.

Why is problem solving important in the workplace?
As a business leader, when too much of your time is spent managing escalations, the lack of problem-solving skills may hurt your business. While you may be hiring talented and capable employees and paying them well, it is only when you harness their full potential and translate that into business value that it is considered a successful hire.
The impact of continuing with poor problem-solving skills may show up in your organization as operational inefficiencies that may also manifest in product quality issues, defects, re-work and non-conformance to design specifications. When the product is defective, or the service is not up to the mark, it directly affects your customer’s experience and consequently reflects on the company’s profile.
At times, poor problem-solving skills could lead to missed market opportunities, slow time to market, customer dissatisfaction, regulatory compliance issues, and declining employee morale.
Problem-solving skills are important for individual business leaders as well. Suppose you’re busy responding to frequent incidents that have the same variables. In that case, this prevents you from focusing your time and effort on improving the future success of business outcomes.
Proven methods to assess and improve problem-solving skills
Pre-employment problem-solving skill assessment .
Recent research indicates that up to 85% of resumes contain misleading statements. Similarly, interviews are subjective and ultimately serve as poor predictors of job performance .
To provide a reliable and objective means of gathering job-related information on candidates, you must validate and develop pre-employment problem-solving assessments. You can further use the data from pre-employment tests to make informed and defensible hiring decisions.
Depending on the job profile, below are examples of pre-employment problem-solving assessment tests:
Personality tests: The rise of personality testing in the 20th century was an endeavor to maximize employee potential. Personality tests help to identify workplace patterns, relevant characteristics, and traits, and to assess how people may respond to different situations.
Examples of personality tests include the Big five personality traits test and Mercer | Mettl’s Dark Personality Inventory .
Cognitive ability test: A pre-employment aptitude test assesses individuals’ abilities such as critical thinking, verbal reasoning, numerical ability, problem-solving, decision-making, etc., which are indicators of a person’s intelligence quotient (IQ). The test results provide data about on-the-job performance. It also assesses current and potential employees for different job levels.
Criteria Cognitive Aptitude test , McQuaig Mental Agility Test , and Hogan Business Reasoning Inventory are commonly used cognitive ability assessment tests.
Convergent and divergent thinking methods
American psychologist JP Guilford coined the terms “convergent thinking” and “divergent thinking” in the 1950s.
Convergent thinking involves starting with pieces of information and then converging around a solution. An example would be determining the correct answer to a multiple-choice question.
The nature of the question does not demand creativity but rather inherently encourages a person to consider the veracity of each answer provided before selecting the single correct one.
Divergent thinking, on the other hand, starts with a prompt that encourages people to think critically, diverging towards distinct answers. An example of divergent thinking would be asking open-ended questions.
Here’s an example of what convergent thinking and a divergent problem-solving model would look like.

The 5 whys method , developed by Sakichi Toyoda, is part of the Toyota production system. In this method, when you come across a problem, you analyze the root cause by asking “Why?” five times. By recognizing the countermeasure, you can prevent the problem from recurring. Here’s an example of the 5 whys method.

Source: Kanbanzie
This method is specifically useful when you have a recurring problem that reoccurs despite repeated actions to address it. It indicates that you are treating the symptoms of the problem and not the actual problem itself.
Starbursting
While brainstorming is about the team coming together to try to find answers, starbursting flips it over and asks everyone to think of questions instead. Here’s an example of the starbursting method.

The idea of this method is to go and expand from here, layering more and more questions until you’ve covered every eventuality of the problem.
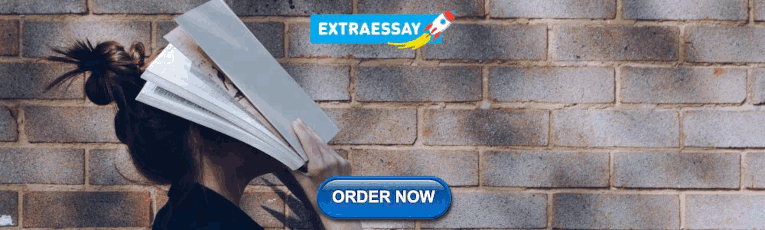
Use of data analysis to measure improvement in problem-solving skills for your organization
Problem-solving and data analytics are often used together. Supporting data is very handy whenever a particular problem occurs. By using data analytics, you can find the supporting data and analyze it to use for solving a specific problem.
However, we must emphasize that the data you’re using to solve the problem is accurate and complete. Otherwise, misleading data may take you off track of the problem at hand or even make it appear more complex than it is. Moreover, as you gain knowledge about the current problem, it further eases the way to solve it.
Let’s dig deeper into the top 3 reasons data analytics is important in problem-solving.
1. Uncover hidden details
Modern data analytics tools have numerous features that let you analyze the given data thoroughly and find hidden or repeating trends without needing any extra human effort. These automated tools are great at extracting the depths of data, going back way into the past.
2. Automated models
Automation is the future. Businesses don’t have enough time or the budget to encourage manual workforces to go through loads of data to solve business problems. Instead, the tools can collect, combine, clean, and transform the relevant data all by themselves and finally use it to predict the solutions.
3. Explore similar problems
When you use a data analytics approach to solve problems, you can collect all the data available and store it. It can assist you when you find yourself in similar problems, providing references for how such issues were tackled in the past.
If you’re looking for ways to help develop problem-solving skills in the workplace and want to build a team of employees who can solve their own problems, contact us to learn how we can help you achieve it.
Stay up to date with our newsletter
Every month, we’ll send you a curated newsletter with our updates and the latest industry news.
More stories we think you will like

The role of employee commitment: Why it matters and how to boost it

Human-Computer Interaction – What the Future Ep. 1
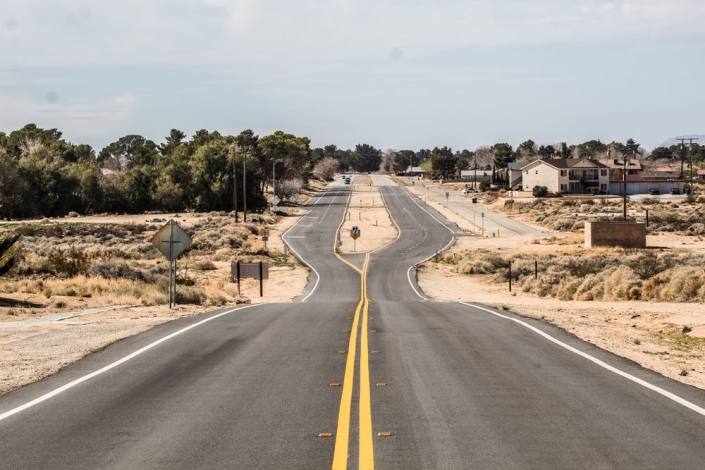
The right decision-making strategy for leaders: Do nothing or take action?
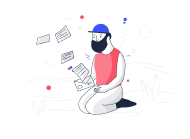
HRForecast newsletter
Get only relevant and insightful letters from us every month

Not a customer yet? Contact us

Career at HRForecast
Why hrforecast.
- Customer Stories
- Trust and Security
- Data Analytics Approach
- IT Skills Analytics
- smartPeople

2023 © Copyright - HRForecast | Imprint | Privacy policy | Terms and conditions (MSA)

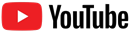
- TPC and eLearning
- What's NEW at TPC?
- Read Watch Interact
- Practice Review Test
- Teacher-Tools
- Subscription Selection
- Seat Calculator
- Ad Free Account
- Edit Profile Settings
- Classes (Version 2)
- Student Progress Edit
- Task Properties
- Export Student Progress
- Task, Activities, and Scores
- Metric Conversions Questions
- Metric System Questions
- Metric Estimation Questions
- Significant Digits Questions
- Proportional Reasoning
- Acceleration
- Distance-Displacement
- Dots and Graphs
- Graph That Motion
- Match That Graph
- Name That Motion
- Motion Diagrams
- Pos'n Time Graphs Numerical
- Pos'n Time Graphs Conceptual
- Up And Down - Questions
- Balanced vs. Unbalanced Forces
- Change of State
- Force and Motion
- Mass and Weight
- Match That Free-Body Diagram
- Net Force (and Acceleration) Ranking Tasks
- Newton's Second Law
- Normal Force Card Sort
- Recognizing Forces
- Air Resistance and Skydiving
- Solve It! with Newton's Second Law
- Which One Doesn't Belong?
- Component Addition Questions
- Head-to-Tail Vector Addition
- Projectile Mathematics
- Trajectory - Angle Launched Projectiles
- Trajectory - Horizontally Launched Projectiles
- Vector Addition
- Vector Direction
- Which One Doesn't Belong? Projectile Motion
- Forces in 2-Dimensions
- Being Impulsive About Momentum
- Explosions - Law Breakers
- Hit and Stick Collisions - Law Breakers
- Case Studies: Impulse and Force
- Impulse-Momentum Change Table
- Keeping Track of Momentum - Hit and Stick
- Keeping Track of Momentum - Hit and Bounce
- What's Up (and Down) with KE and PE?
- Energy Conservation Questions
- Energy Dissipation Questions
- Energy Ranking Tasks
- LOL Charts (a.k.a., Energy Bar Charts)
- Match That Bar Chart
- Words and Charts Questions
- Name That Energy
- Stepping Up with PE and KE Questions
- Case Studies - Circular Motion
- Circular Logic
- Forces and Free-Body Diagrams in Circular Motion
- Gravitational Field Strength
- Universal Gravitation
- Angular Position and Displacement
- Linear and Angular Velocity
- Angular Acceleration
- Rotational Inertia
- Balanced vs. Unbalanced Torques
- Getting a Handle on Torque
- Torque-ing About Rotation
- Properties of Matter
- Fluid Pressure
- Buoyant Force
- Sinking, Floating, and Hanging
- Pascal's Principle
- Flow Velocity
- Bernoulli's Principle
- Balloon Interactions
- Charge and Charging
- Charge Interactions
- Charging by Induction
- Conductors and Insulators
- Coulombs Law
- Electric Field
- Electric Field Intensity
- Polarization
- Case Studies: Electric Power
- Know Your Potential
- Light Bulb Anatomy
- I = ∆V/R Equations as a Guide to Thinking
- Parallel Circuits - ∆V = I•R Calculations
- Resistance Ranking Tasks
- Series Circuits - ∆V = I•R Calculations
- Series vs. Parallel Circuits
- Equivalent Resistance
- Period and Frequency of a Pendulum
- Pendulum Motion: Velocity and Force
- Energy of a Pendulum
- Period and Frequency of a Mass on a Spring
- Horizontal Springs: Velocity and Force
- Vertical Springs: Velocity and Force
- Energy of a Mass on a Spring
- Decibel Scale
- Frequency and Period
- Closed-End Air Columns
- Name That Harmonic: Strings
- Rocking the Boat
- Wave Basics
- Matching Pairs: Wave Characteristics
- Wave Interference
- Waves - Case Studies
- Color Addition and Subtraction
- Color Filters
- If This, Then That: Color Subtraction
- Light Intensity
- Color Pigments
- Converging Lenses
- Curved Mirror Images
- Law of Reflection
- Refraction and Lenses
- Total Internal Reflection
- Who Can See Who?
- Formulas and Atom Counting
- Atomic Models
- Bond Polarity
- Entropy Questions
- Cell Voltage Questions
- Heat of Formation Questions
- Reduction Potential Questions
- Oxidation States Questions
- Measuring the Quantity of Heat
- Hess's Law
- Oxidation-Reduction Questions
- Galvanic Cells Questions
- Thermal Stoichiometry
- Molecular Polarity
- Quantum Mechanics
- Balancing Chemical Equations
- Bronsted-Lowry Model of Acids and Bases
- Classification of Matter
- Collision Model of Reaction Rates
- Density Ranking Tasks
- Dissociation Reactions
- Complete Electron Configurations
- Elemental Measures
- Enthalpy Change Questions
- Equilibrium Concept
- Equilibrium Constant Expression
- Equilibrium Calculations - Questions
- Equilibrium ICE Table
- Intermolecular Forces Questions
- Ionic Bonding
- Lewis Electron Dot Structures
- Limiting Reactants
- Line Spectra Questions
- Mass Stoichiometry
- Measurement and Numbers
- Metals, Nonmetals, and Metalloids
- Metric Estimations
- Metric System
- Molarity Ranking Tasks
- Mole Conversions
- Name That Element
- Names to Formulas
- Names to Formulas 2
- Nuclear Decay
- Particles, Words, and Formulas
- Periodic Trends
- Precipitation Reactions and Net Ionic Equations
- Pressure Concepts
- Pressure-Temperature Gas Law
- Pressure-Volume Gas Law
- Chemical Reaction Types
- Significant Digits and Measurement
- States Of Matter Exercise
- Stoichiometry Law Breakers
- Stoichiometry - Math Relationships
- Subatomic Particles
- Spontaneity and Driving Forces
- Gibbs Free Energy
- Volume-Temperature Gas Law
- Acid-Base Properties
- Energy and Chemical Reactions
- Chemical and Physical Properties
- Valence Shell Electron Pair Repulsion Theory
- Writing Balanced Chemical Equations
- Mission CG1
- Mission CG10
- Mission CG2
- Mission CG3
- Mission CG4
- Mission CG5
- Mission CG6
- Mission CG7
- Mission CG8
- Mission CG9
- Mission EC1
- Mission EC10
- Mission EC11
- Mission EC12
- Mission EC2
- Mission EC3
- Mission EC4
- Mission EC5
- Mission EC6
- Mission EC7
- Mission EC8
- Mission EC9
- Mission RL1
- Mission RL2
- Mission RL3
- Mission RL4
- Mission RL5
- Mission RL6
- Mission KG7
- Mission RL8
- Mission KG9
- Mission RL10
- Mission RL11
- Mission RM1
- Mission RM2
- Mission RM3
- Mission RM4
- Mission RM5
- Mission RM6
- Mission RM8
- Mission RM10
- Mission LC1
- Mission RM11
- Mission LC2
- Mission LC3
- Mission LC4
- Mission LC5
- Mission LC6
- Mission LC8
- Mission SM1
- Mission SM2
- Mission SM3
- Mission SM4
- Mission SM5
- Mission SM6
- Mission SM8
- Mission SM10
- Mission KG10
- Mission SM11
- Mission KG2
- Mission KG3
- Mission KG4
- Mission KG5
- Mission KG6
- Mission KG8
- Mission KG11
- Mission F2D1
- Mission F2D2
- Mission F2D3
- Mission F2D4
- Mission F2D5
- Mission F2D6
- Mission KC1
- Mission KC2
- Mission KC3
- Mission KC4
- Mission KC5
- Mission KC6
- Mission KC7
- Mission KC8
- Mission AAA
- Mission SM9
- Mission LC7
- Mission LC9
- Mission NL1
- Mission NL2
- Mission NL3
- Mission NL4
- Mission NL5
- Mission NL6
- Mission NL7
- Mission NL8
- Mission NL9
- Mission NL10
- Mission NL11
- Mission NL12
- Mission MC1
- Mission MC10
- Mission MC2
- Mission MC3
- Mission MC4
- Mission MC5
- Mission MC6
- Mission MC7
- Mission MC8
- Mission MC9
- Mission RM7
- Mission RM9
- Mission RL7
- Mission RL9
- Mission SM7
- Mission SE1
- Mission SE10
- Mission SE11
- Mission SE12
- Mission SE2
- Mission SE3
- Mission SE4
- Mission SE5
- Mission SE6
- Mission SE7
- Mission SE8
- Mission SE9
- Mission VP1
- Mission VP10
- Mission VP2
- Mission VP3
- Mission VP4
- Mission VP5
- Mission VP6
- Mission VP7
- Mission VP8
- Mission VP9
- Mission WM1
- Mission WM2
- Mission WM3
- Mission WM4
- Mission WM5
- Mission WM6
- Mission WM7
- Mission WM8
- Mission WE1
- Mission WE10
- Mission WE2
- Mission WE3
- Mission WE4
- Mission WE5
- Mission WE6
- Mission WE7
- Mission WE8
- Mission WE9
- Vector Walk Interactive
- Name That Motion Interactive
- Kinematic Graphing 1 Concept Checker
- Kinematic Graphing 2 Concept Checker
- Graph That Motion Interactive
- Two Stage Rocket Interactive
- Rocket Sled Concept Checker
- Force Concept Checker
- Free-Body Diagrams Concept Checker
- Free-Body Diagrams The Sequel Concept Checker
- Skydiving Concept Checker
- Elevator Ride Concept Checker
- Vector Addition Concept Checker
- Vector Walk in Two Dimensions Interactive
- Name That Vector Interactive
- River Boat Simulator Concept Checker
- Projectile Simulator 2 Concept Checker
- Projectile Simulator 3 Concept Checker
- Hit the Target Interactive
- Turd the Target 1 Interactive
- Turd the Target 2 Interactive
- Balance It Interactive
- Go For The Gold Interactive
- Egg Drop Concept Checker
- Fish Catch Concept Checker
- Exploding Carts Concept Checker
- Collision Carts - Inelastic Collisions Concept Checker
- Its All Uphill Concept Checker
- Stopping Distance Concept Checker
- Chart That Motion Interactive
- Roller Coaster Model Concept Checker
- Uniform Circular Motion Concept Checker
- Horizontal Circle Simulation Concept Checker
- Vertical Circle Simulation Concept Checker
- Race Track Concept Checker
- Gravitational Fields Concept Checker
- Orbital Motion Concept Checker
- Angular Acceleration Concept Checker
- Balance Beam Concept Checker
- Torque Balancer Concept Checker
- Aluminum Can Polarization Concept Checker
- Charging Concept Checker
- Name That Charge Simulation
- Coulomb's Law Concept Checker
- Electric Field Lines Concept Checker
- Put the Charge in the Goal Concept Checker
- Circuit Builder Concept Checker (Series Circuits)
- Circuit Builder Concept Checker (Parallel Circuits)
- Circuit Builder Concept Checker (∆V-I-R)
- Circuit Builder Concept Checker (Voltage Drop)
- Equivalent Resistance Interactive
- Pendulum Motion Simulation Concept Checker
- Mass on a Spring Simulation Concept Checker
- Particle Wave Simulation Concept Checker
- Boundary Behavior Simulation Concept Checker
- Slinky Wave Simulator Concept Checker
- Simple Wave Simulator Concept Checker
- Wave Addition Simulation Concept Checker
- Standing Wave Maker Simulation Concept Checker
- Color Addition Concept Checker
- Painting With CMY Concept Checker
- Stage Lighting Concept Checker
- Filtering Away Concept Checker
- InterferencePatterns Concept Checker
- Young's Experiment Interactive
- Plane Mirror Images Interactive
- Who Can See Who Concept Checker
- Optics Bench (Mirrors) Concept Checker
- Name That Image (Mirrors) Interactive
- Refraction Concept Checker
- Total Internal Reflection Concept Checker
- Optics Bench (Lenses) Concept Checker
- Kinematics Preview
- Velocity Time Graphs Preview
- Moving Cart on an Inclined Plane Preview
- Stopping Distance Preview
- Cart, Bricks, and Bands Preview
- Fan Cart Study Preview
- Friction Preview
- Coffee Filter Lab Preview
- Friction, Speed, and Stopping Distance Preview
- Up and Down Preview
- Projectile Range Preview
- Ballistics Preview
- Juggling Preview
- Marshmallow Launcher Preview
- Air Bag Safety Preview
- Colliding Carts Preview
- Collisions Preview
- Engineering Safer Helmets Preview
- Push the Plow Preview
- Its All Uphill Preview
- Energy on an Incline Preview
- Modeling Roller Coasters Preview
- Hot Wheels Stopping Distance Preview
- Ball Bat Collision Preview
- Energy in Fields Preview
- Weightlessness Training Preview
- Roller Coaster Loops Preview
- Universal Gravitation Preview
- Keplers Laws Preview
- Kepler's Third Law Preview
- Charge Interactions Preview
- Sticky Tape Experiments Preview
- Wire Gauge Preview
- Voltage, Current, and Resistance Preview
- Light Bulb Resistance Preview
- Series and Parallel Circuits Preview
- Thermal Equilibrium Preview
- Linear Expansion Preview
- Heating Curves Preview
- Electricity and Magnetism - Part 1 Preview
- Electricity and Magnetism - Part 2 Preview
- Vibrating Mass on a Spring Preview
- Period of a Pendulum Preview
- Wave Speed Preview
- Slinky-Experiments Preview
- Standing Waves in a Rope Preview
- Sound as a Pressure Wave Preview
- DeciBel Scale Preview
- DeciBels, Phons, and Sones Preview
- Sound of Music Preview
- Shedding Light on Light Bulbs Preview
- Models of Light Preview
- Electromagnetic Radiation Preview
- Electromagnetic Spectrum Preview
- EM Wave Communication Preview
- Digitized Data Preview
- Light Intensity Preview
- Concave Mirrors Preview
- Object Image Relations Preview
- Snells Law Preview
- Reflection vs. Transmission Preview
- Magnification Lab Preview
- Reactivity Preview
- Ions and the Periodic Table Preview
- Periodic Trends Preview
- Intermolecular Forces Preview
- Melting Points and Boiling Points Preview
- Reaction Rates Preview
- Ammonia Factory Preview
- Stoichiometry Preview
- Nuclear Chemistry Preview
- Gaining Teacher Access
- Tasks and Classes
- Tasks - Classic
- Subscription
- Subscription Locator
- 1-D Kinematics
- Newton's Laws
- Vectors - Motion and Forces in Two Dimensions
- Momentum and Its Conservation
- Work and Energy
- Circular Motion and Satellite Motion
- Thermal Physics
- Static Electricity
- Electric Circuits
- Vibrations and Waves
- Sound Waves and Music
- Light and Color
- Reflection and Mirrors
- About the Physics Interactives
- Task Tracker
- Usage Policy
- Newtons Laws
- Vectors and Projectiles
- Forces in 2D
- Momentum and Collisions
- Circular and Satellite Motion
- Balance and Rotation
- Electromagnetism
- Waves and Sound
- Atomic Physics
- Forces in Two Dimensions
- Work, Energy, and Power
- Circular Motion and Gravitation
- Sound Waves
- 1-Dimensional Kinematics
- Circular, Satellite, and Rotational Motion
- Einstein's Theory of Special Relativity
- Waves, Sound and Light
- QuickTime Movies
- About the Concept Builders
- Pricing For Schools
- Directions for Version 2
- Measurement and Units
- Relationships and Graphs
- Rotation and Balance
- Vibrational Motion
- Reflection and Refraction
- Teacher Accounts
- Task Tracker Directions
- Kinematic Concepts
- Kinematic Graphing
- Wave Motion
- Sound and Music
- About CalcPad
- 1D Kinematics
- Vectors and Forces in 2D
- Simple Harmonic Motion
- Rotational Kinematics
- Rotation and Torque
- Rotational Dynamics
- Electric Fields, Potential, and Capacitance
- Transient RC Circuits
- Light Waves
- Units and Measurement
- Stoichiometry
- Molarity and Solutions
- Thermal Chemistry
- Acids and Bases
- Kinetics and Equilibrium
- Solution Equilibria
- Oxidation-Reduction
- Nuclear Chemistry
- Newton's Laws of Motion
- Work and Energy Packet
- Static Electricity Review
- NGSS Alignments
- 1D-Kinematics
- Projectiles
- Circular Motion
- Magnetism and Electromagnetism
- Graphing Practice
- About the ACT
- ACT Preparation
- For Teachers
- Other Resources
- Solutions Guide
- Solutions Guide Digital Download
- Motion in One Dimension
- Work, Energy and Power
- TaskTracker
- Other Tools
- Algebra Based Physics
- Frequently Asked Questions
- Purchasing the Download
- Purchasing the CD
- Purchasing the Digital Download
- About the NGSS Corner
- NGSS Search
- Force and Motion DCIs - High School
- Energy DCIs - High School
- Wave Applications DCIs - High School
- Force and Motion PEs - High School
- Energy PEs - High School
- Wave Applications PEs - High School
- Crosscutting Concepts
- The Practices
- Physics Topics
- NGSS Corner: Activity List
- NGSS Corner: Infographics
- About the Toolkits
- Position-Velocity-Acceleration
- Position-Time Graphs
- Velocity-Time Graphs
- Newton's First Law
- Newton's Second Law
- Newton's Third Law
- Terminal Velocity
- Projectile Motion
- Forces in 2 Dimensions
- Impulse and Momentum Change
- Momentum Conservation
- Work-Energy Fundamentals
- Work-Energy Relationship
- Roller Coaster Physics
- Satellite Motion
- Electric Fields
- Circuit Concepts
- Series Circuits
- Parallel Circuits
- Describing-Waves
- Wave Behavior Toolkit
- Standing Wave Patterns
- Resonating Air Columns
- Wave Model of Light
- Plane Mirrors
- Curved Mirrors
- Teacher Guide
- Using Lab Notebooks
- Current Electricity
- Light Waves and Color
- Reflection and Ray Model of Light
- Refraction and Ray Model of Light
- Classes (Legacy Version)
- Teacher Resources
- Subscriptions

- Newton's Laws
- Einstein's Theory of Special Relativity
- About Concept Checkers
- School Pricing
- Newton's Laws of Motion
- Newton's First Law
- Newton's Third Law
Chemistry: Units and Measurement
Accessibility Links
- Skip to content
- Skip to search IOPscience
- Skip to Journals list
- Accessibility help
- Accessibility Help
Click here to close this panel.

As a society-owned publisher with a legacy of serving scientific communities, we are committed to offering a home to all scientifically valid and rigorously reviewed research. In doing so, we aim to accelerate the dissemination of scientific knowledge and the advancement of scholarly communications to benefit all.
Journal of Physics Communications supports this mission and actively demonstrates our core values of inclusive publishing and trusted science . To find out more about these values and how they can help you publish your next paper with us, visit our journal scope .
Purpose-led Publishing is a coalition of three not-for-profit publishers in the field of physical sciences: AIP Publishing, the American Physical Society and IOP Publishing.
Together, as publishers that will always put purpose above profit, we have defined a set of industry standards that underpin high-quality, ethical scholarly communications.
We are proudly declaring that science is our only shareholder.
What does it take to solve the measurement problem?
Jonte R Hance 1 and Sabine Hossenfelder 2
Published 11 October 2022 • © 2022 The Author(s). Published by IOP Publishing Ltd Journal of Physics Communications , Volume 6 , Number 10 Citation Jonte R Hance and Sabine Hossenfelder 2022 J. Phys. Commun. 6 102001 DOI 10.1088/2399-6528/ac96cf
Article metrics
8270 Total downloads
Share this article
Author e-mails.
Author affiliations
1 Quantum Engineering Technology Laboratories, Department of Electrical and Electronic Engineering, University of Bristol, Woodland Road, Bristol, BS8 1US, United Kingdom
2 Frankfurt Institute for Advanced Studies, Ruth-Moufang-Str. 1, D-60438 Frankfurt am Main, Germany
Jonte R Hance https://orcid.org/0000-0001-8587-7618
Sabine Hossenfelder https://orcid.org/0000-0002-2515-3842
- Received 5 July 2022
- Revised 2 September 2022
- Accepted 30 September 2022
- Published 11 October 2022
Peer review information
Method : Single-anonymous Revisions: 1 Screened for originality? No
Buy this article in print
We summarise different aspects of the measurement problem in quantum mechanics. We argue that it is a real problem which requires a solution, and identify the properties a theory needs to solve the problem. We show that no current interpretation of quantum mechanics solves the problem, and that, being interpretations rather than extensions of quantum mechanics, they cannot solve it. Finally, we speculate what a solution of the measurement problem might be good for.
Export citation and abstract BibTeX RIS
Original content from this work may be used under the terms of the Creative Commons Attribution 4.0 licence . Any further distribution of this work must maintain attribution to the author(s) and the title of the work, journal citation and DOI.
1. Introduction
Quantum mechanics, in its standard formulation (often referred to as the Copenhagen Interpretation), has two different axioms for its time evolution. The one is the deterministic, linear Schrödinger equation, the other the non-deterministic, non-linear, and generically non-local collapse of the wave function. The latter is sometimes also referred to as the 'reduction' or 'update' of the wave function.
The collapse of the wave function must be mathematically applied in the event of a measurement, yet the theory leaves unspecified just what constitutes a measurement. While this problem is a century old, it is still hotly debated [ 1 ]. We will argue here that this is more than just unsatisfactory, but is a severe shortcoming that requires a solution. Previous accounts of some of the aspects discussed here can be found in [ 2 – 4 ].
Throughout this paper we use natural units ( ℏ = c = 1).
2. The axioms
Below, we will take an instrumental perspective on quantum mechanics. For the purposes of this paper, quantum mechanics is a mathematical machine. Into this machine we insert some known properties of a system that we have prepared in the laboratory. Then we do the maths, get out a prediction for measurement outcomes, and compare the prediction to the observation.
This brief summary doesn't do justice to all the subtleties of quantum mechanics. Among other things, it doesn't specify what the Hamiltonian operator is or how one gets the operators corresponding to the measurement observables. However, the question of what those operators look like will not concern us in the rest of this paper.
Of course, there are many different ways to approach quantum mechanics axiomatically. A notable attempt is for example that proposed by Hardy [ 5 ]. We will here use the above set of axioms because it is the way quantum mechanics is typically taught to students, and we believe that this familiarity will make our argument more accessible.
It is sometimes questioned whether the Collapse Postulate is actually necessary (e.g. in [ 6 ]). Without it, quantum mechanics would still correctly predict average values for large numbers of repetitions of the same experiment. This is the statistical interpretation suggested by Ballentine [ 7 ].
However, we do not merely observe averages of many experiments: we also observe the outcomes of individual experiments. And we know from observations that the outcome of an experiment is never a superposition of detector eigenstates, nor is it ever a mixed state (whatever that would look like)—a detector either detects a particle or it doesn't, but not both. As Maudlin put it [ 2 ], 'it is a plain physical fact that some individual cats are alive and some dead' (emphasis original). Without the Collapse Postulate, the mathematical machinery of quantum mechanics just does not describe this aspect of physical reality correctly.
This means quantum mechanics without the Collapse Postulate is not wrong, but it describes less of what we observe. The Collapse Postulate is hence useful, and part of the axioms because it increases the explanatory power of the theory. It cannot simply be discarded.
The necessity of the Collapse Postulate to describe observations is not a problem in itself, but it gives rise to the problems discussed below.
3. The problems
3.1. the heisenberg cut.
The most obvious problem with the axioms of quantum mechanics is the term 'measurement,' which remains undefined.
The need to refer to a measurement strikes one as suspect right away, because quantum mechanics is commonly believed to be a fundamental theory for the microscopic constituents of matter. But if quantum mechanics was fundamental, then the behavior of macroscopic objects (like measurement devices) should be derivable from it. The theory should explain what a measurement is, rather than require it in one of its axioms.
This problem has been known since the earliest days of quantum mechanics and is often referred to as the 'Heisenberg Cut', alluding to the question just where to make the 'cut' between the unitary Schrödinger evolution and the non-unitary measurement update [ 8 ].
One may object that it is a rather inconsequential problem, because in practice we know that, roughly speaking, measurements are caused by large things. This is how we have used the axioms of quantum mechanics so far, and it has worked reasonably well. Us not knowing just how large a device needs to be to induce a measurement hasn't really been an issue. However, the smaller the measurement devices we can manufacture become, the more pressing the question becomes.
That we cannot answer this question has practical consequences already. A few years ago, Frauchinger and Renner argued that quantum mechanics cannot consistently describe the use of itself [ 9 ]. But as was pointed out in [ 10 , 11 ], the origin of the inconsistency is that Frauchinger and Renner did not specify what a measurement device is. They treated a measurement merely as a sufficiently strong correlation, which leads to a basis ambiguity that allows mutually contradictory results. The problem was directly created by them not making a Heisenberg Cut.
This alleged inconsistency was later experimentally tested with a setup that, stunningly enough, used single photons as stand-ins for observers that supposedly make measurements [ 12 ]. Now, it may be a matter of debate just exactly where to apply the Heisenberg Cut, but Heisenberg would probably be surprised to learn that by 2018 physicists would have confused themselves so much over quantum mechanics that they came to believe single photons are observers. What the Frauchinger-Renner paradox therefore establishes is that quantum mechanics can result in inconsistent predictions so long as we do not add a definition for what a measurement device is to the axioms of quantum mechanics.
This problem could easily be remedied—after all, we would just need to write down a definition. However, the definition for a measurement of course should not just remove the risk of inconsistent predictions but also agree with observations, and this just returns us to the question of where to apply the cut.
It was argued in [ 10 , 11 ] that the Frauchinger-Renner paradox can be resolved by taking into account decoherence. But decoherence is still a unitary and linear process that is described by the Schrödinger equation. It can therefore not give rise to the measurement update, so this still has to be added to the axioms.
Decoherence can to some extent be used to identify the circumstances under which the measurement update should be applied, but this idea has its problems too. We will comment on this in more detail in section 5.4 . For now, let us just note that decoherence alone simply will not evolve a system into a single detector eigenstate, and hence does not agree with what we observe. Tracing out the environment gives us a mixed state, but that is still not what we observe, not to mention that taking this trace is not a physical process, and therefore doesn't change anything about the state of the system.
3.2. The classical limit
Before quantum mechanics, there was classical mechanics, and classical mechanics still describes most of our observations correctly. Unfortunately, quantum mechanics doesn't correctly reproduce it.
It has long been known that recovering the classical time-evolution for suitably defined expectation values in quantum mechanics works properly only for integrable systems [ 13 , 14 ]. For chaotic systems, on the other hand, the quantum–classical correspondence breaks down after a finite amount of time [ 15 , 16 ]. As pointed out by Zurek [ 17 ] (see also [ 18 ]), this time may be long, but not so long that we can't observe it. Zurek estimates that the chaotic motion of Hyperion (a moon of Saturn) would last less than 20 years if we used the Schrödinger evolution for its constituents. Alas, it has lasted hundreds of millions of years.
Again, decoherence allegedly solves the problem. If one includes the interaction of Hyperion with dust and photons in its environment, then one sees that the Moon becomes entangled with its environment much faster than its motion could significantly deviate from the classical limit.
However, we have to note again that tracing out the environment is not a physical process. Therefore, all entanglement gives us is a very big entangled state. What we would have to do to get a classical non-linear motion of a localised object is to actually include the Collapse Postulate into the dynamical law. This shouldn't be so surprising: the non-linearity has to come from somewhere.
To do this, we would have to know when and how the collapse happens, but we don't. Do the photons detect the Moon? Or does the Moon detect the photons? If there's neither photons nor dust, does the Moon detect itself? And if the state collapses, then just exactly when do we update what part of the Moon's wave function? These are not philosophical questions; these are questions about how to apply the axioms of our quantum machinery, and they are questions that we simply do not have an answer to.
Another way to look at this problem was summarised by Klein [ 19 ]: the ℏ → 0 limit of quantum mechanics just does not reproduce classical mechanics, unless one restricts oneself to special states (generalised coherent states) and specific types of potentials.
3.3. Locality and causality
The trouble with Hyperion brings us to the next problem. The collapse of the wave function in quantum mechanics is instantaneous; it happens at the same time everywhere in space. This 'spooky action at a distance' [ 20 ] understandably worried Einstein because it seems incompatible with the speed-of-light limit. We know now [ 21 ] that no information can be exchanged with the collapse of the wave function, but this doesn't explain how to apply the collapse postulate.
Consequently, people have debated for decades how to make the collapse compatible with relativistic invariance, and whether it requires backwards causation [ 22 – 24 ]. No resolution has been reached.
We acknowledge, however, that the non-locality of the collapse is not a problem for the instrumentalist because, in the Copenhagen Interpretation, collapse is not necessarily a physical process, and is not related to any observable. So, in just which reference frame it happens does not matter; there are no predictions tied to this frame anyway.
The reason we mention locality and causality is that these matter when we cross from special to general relativity, as we discuss next.
3.4. Conservation laws
In Einstein's field equations
Most important for our purposes is that the semi-classical approximation is generally believed to be at least approximately correct in the weak-field limit and if fluctuations of the stress-energy tensor are small [ 25 , 26 ].
But the expectation value in the stress-energy tensor generically has to be updated upon measurement with the collapse of the wave function. And since this update is non-local, it violates the (contracted) Bianchi-identities which the left side of Einstein's field equations do fulfil and that are usually associated with local stress-energy conservation [ 27 , 28 ].
Take for example a photon ( γ ) which passes through a beam splitter ( S ). Due to momentum conservation, this creates an entangled state
where p is the momentum and we will assume that it is really the mean value of a suitably localised wave-packet. The expectation value of this entangled state is a sum of localised momentum distributions going out in opposite directions from the beam splitter, while the mean momentum transferred to the beam splitter is zero.
According to the axioms of the quantum machine, when we measure the photon we have to update the wave function. In this moment, the momentum of one detector suddenly increases by p (− p ) and that of the beam splitter switches to − p ( p ). The momentum is conserved, but how did it get to the detector? Without general relativity there is no observable tied to this question, but in general relativity there is: even though it is too small to measure, something must have happened with the space-time curvature. But what?
Again this is a problem for which we simply do not have an answer. We do not have the mathematics to describe what happens with the gravitational field of a particle if its wave function collapses, not even approximately.
However, this shows an issue with approaches to the measurement problem which claim that wavefunction collapse is just a nonlocal process (e.g. Shimony's 'passion-at-a-distance' [ 29 ]): while they may work to some extent for non-relativistic many-particle quantum mechanics, they are difficult to reconcile with both relativistic quantum mechanics and gravity. And just letting go of relativistic covariance is not an option either since it is experimentally extremely well-confirmed [ 30 , 31 ]. That is to say, while it cannot strictly speaking be ruled out that a nonlocal approach can be made 'local enough' to agree with all available evidence, it seems like a stretch. The more pragmatic approach is to just look for an approach that is local and relativistically covariant to begin with.
4. What can we say about the solution?
4.1. solution requirements.
Requirement 1. Agree with all existing data.
Requirement 2. Reproduce quantum mechanics, including the Collapse Postulate (Axiom 4 ) and Born's Rule (Axiom 5 ), in a well-defined limit.
Requirement 3. Give an unambiguous answer to the question of what a measurement device is, at least in principle.
Requirement 4. Reproduce classical physics in a well-defined limit.
Requirement 5. Resolve the inconsistency between the non-local measurement collapse and local stress-energy conservation.
Requirement 1 must be fulfilled by any scientifically adequate theory and we just add it for completeness. Requirement 2 recognises that within its domain, standard quantum mechanics is incredibly well-supported by data and a new theory would not become accepted without reproducing the achievements of its predecessor. Requirement 3 is necessary to resolve the problem laid out in 3.1 , Requirement 4 the problem laid out in 3.2 , and Requirement 5 the problem discussed in 3.3 and 3.4 .
Requirements 2 and 4 have the same form—they both consist of ensuring the solution reproduces an established, well-proven theory in some well-defined limit. Their resolution might well be related; however, this does not have to be so, which is why we list them separately. Likewise, we would expect that requirements 2 and 4 can be used to show that requirement 1 is fulfilled. Again, however, this does not have to be the case—a limit might be well-defined and yet its result might just be in conflict with observations—so we list them separately.
We added the phrase 'at least in principle' in Requation ( 3 ) to make clear that no one expects it to be of much practical use to calculate from first principles what the arrangements of elementary particles in a detector are. To find out what a detector is, it is much more practical to just test whether it actually detects the thing we want to detect. However, even though it may be unpractical or even unfeasible to perform an exact calculation, we should be able to identify some general properties for what it takes for a collection of particles to act as a measurement device.
As mentioned previously, Requation ( 5 ) might be resolved by a theory of quantum gravity. However, the currently most well-developed approaches to quantum gravity do not address the measurement process. Similarly, these requirements could feasibly be met by a theory which isn't also a theory of quantum gravity. Therefore, we emphasise a theory of quantum gravity is neither necessary nor sufficient to solve the measurement problem.
4.2. Solution properties
Solution 1. The wave function itself evolves according to Axiom 3 and is updated according to Axiom 4 , but it is an incomplete description. The physical state of the system is described by something else that evolves locally though not necessarily deterministically.
Solution 2. The wave function is the complete description of the system, but does not evolve by Axiom 3 and Axiom 4 . It evolves instead according to a different, local evolution law, that is necessarily non-deterministic.
Solution 3. A combination of Solutions 1 and 2 .
Solution 1 is what is commonly called a 'hidden variables' theory. We will instead adopt the convention of [ 2 ] and refer to it as an 'additional variables' theory, to acknowledge that the variables may not, in fact, be hidden—the variables are merely not included in axioms 1 - 6 . It should be noted that according to this definition, details of the detector and environment (as they appear in the decoherence approach) count as additional variables. We will come back to this point later.
For the instrumentalist, all three solutions come down to local, deterministic evolution laws with additional variables. We here use the definition for deterministic from [ 32 ], which is that measurements have definite outcomes (probability 0 or 1). Using this terminology, the laws may be non-predictable, despite being deterministic.
This can be seen by noting that any local, non-deterministic evolution law can be rewritten into a local, deterministic evolution in a theory with additional variables: any time the evolution law is indeterministic (which could be continuously), we encode the possible time-evolutions with an additional variable.
To give a concrete albeit trivial example: we can make the Collapse Postulate deterministic by just using the eigenstate that is the outcome of the collapse as an additional variable. The collapse is then 'determined' by the 'additional variable'. Of course, this is somewhat pointless, because in quantum mechanics this additional variable is unpredictable (hence would well deserve the name 'hidden'), but it serves to show that additional variables could be used to describe the process.
The difference between the solutions is then merely what we call the 'wave function'. Do we reserve the term for that which evolves under Schrödinger equation (Solution 1 ) which has to come out of the mathematics at least in some limit (Requation ( 2 )), or are we okay with using the term for whatever it is that we use to describe the state of the system (Solutions 2 and 3 )? Do we require wave functions to be elements of a Hilbert-space, regardless of their evolution law? Do we want them to factorise for separable systems? Do we want them to be green, or married, or all of the above? In the end, this is just a matter of definition. The instrumentalist doesn't care and concludes that all possible solutions to the problem can be covered with a local and deterministic evolution in a theory with additional variables.
This, together with the undeniable resemblance between the von Neumann-Dirac equation and the Liouville equation, makes it plausible that quantum mechanics is indeed an ensemble description of an underlying statistical theory with the additional variables [ 33 ].
4.3. Solution parameterisation
That this new underlying theory must explain just where the Heisenberg Cut is (Requation ( 3 )) means that it has to bring in some new parameter to quantify how good the statistical approximation is. This parameter cannot be derived from quantum mechanics itself; it must be extracted from experiment.
It is clear that the new transition parameter cannot be something as simple as just the number of particles, not least because that quantity is not in general well-defined. (How many particles are inside an atom? How many particles does the vacuum contain?) Seeing the problems of the decoherence approach (see 5.4 ) it is also unlikely to be any measure of decoherence or entanglement, though the frequency or strength of interactions must play some role. Parameters based on the total mass or energy or gravitational self-energy have no a priori relation to generic measurements, and parameters based on the classical limit (possibly using the action as a quantifier) create a circular problem, because we would need them to know how the classical limit works to begin with.
However, we have reason to be optimistic that this problem is solvable because we have observational limits on the Heisenberg Cut both from above and below, and current technologies are pushing both these limits: by bringing larger objects into quantum states, while at the same time shrinking the size of detectors. It is only a matter of time until experiment will reach a regime in which deviations from quantum mechanics become noticeable. However, this process could be accelerated if we knew better what to look for, which is a task for theory development.
5. Solution attempts
5.1. second quantization.
The problems we discussed in the previous sections do not disappear with second quantization. All quantum field theories are built on the basic axioms of quantum mechanics which we listed in section 2 . In quantum field theory we merely have more complicated ways of describing interactions, and calculating the time-evolution of the system and observables related to it. If anything, it has been argued these problems become more complicated in quantum field theory [ 34 ].
5.2. Understanding quantum mechanics
Some readers may wonder if it is possible that these problems will one day be solved within the context of quantum mechanics itself. Maybe the problem with the classical limit is just that no one has found the right limit. Indeed, it is widely known that generalised coherent states make a promising basis for taking the classical limit [ 35 , 36 ]. But, in light of the problems pointed out by Klein [ 19 ], this would at least entail adding further axioms about what to do for obtaining the classical limit. Within the context of this present argument we would therefore have to consider it a new theory, because its set of axioms would not be equivalent to the one we listed earlier.
5.3. Wave functions as epistemic states
One common strategy to explain away the measurement problem is to argue that the wave function is not an ontic but an epistemic state, and that its collapse is not a physical process. The collapse, so the argument goes, is merely an update of our knowledge about the system, and its non-locality therefore should not worry us. After all, as Bell put it, when the Queen dies, Prince Charles will instantaneously become King, yet no information had to be sent non-locally for this update [ 37 ].
A common strategy to counter this argument is to point out that if the wave function is the complete description of the system, then there is nothing else the wave function could describe knowledge about [ 2 ]. Therefore, most wave function-epistemic views require the wavefunction to be an incomplete representation of the system.
While attempts have been made at formalising this view [ 38 , 39 ] and using it to come to no-go theorems on the wavefunction being in some way epistemic [ 40 – 42 ], these formalisations have fundamental issues [ 43 – 45 ]. Therefore, these no-go theorems contribute little to telling us whether the wavefunction can or cannot be epistemic.
In reality, the two sides of this argument haven't much advanced since the debate between Einstein and Bohr, and at this point it seems unlike they ever will. Let us therefore note that the problems listed in section 3 exist regardless of whether one believes the wave function is epistemic or ontic or how one wants to interpret the collapse. Quantum mechanics is unsatisfactory for the instrumentalist simply because we cannot answer questions about physical reality with it: just what properties does a measurement device need to have? Just what happens with space-time curvature when a photon passes through a beam splitter? Saying that the wave function is epistemic doesn't answer these questions.
5.4. Decoherence
The virtues of the decoherence program can be briefly summarised as follows. Given any system that includes a prepared state, detector, and environment, a detector is a subsystem that can keep a record of at least one aspect of another subsystem, which is the prepared state one wants to measure. To be able to keep a record of (some property of) the prepared state, the detector itself must have states that are stable under interaction with the rest of the system, which is the environment.
These stable detector states are often called 'pointer states' and we will denote them with ∣ I 〉. They keep a record of the prepared state's projection on the eigenstate corresponding to the pointer state, i.e., one gets a product state ∣ I 〉∣ O I 〉. Any superposition of pointer states would rapidly decohere under interaction of the environment, hence not keep a record of what we are interested in.
To describe the process of decoherence formally, one takes the density matrix of pointer states, prepared states, and environment. One estimates how quickly they become entangled and how much this affects the relative phases between the pointer states. This is the process of decoherence. It must be stressed that this process is fully described by the Schrödinger equation. After that, one traces out the environment, and obtains a mixed state whose probabilities are given by Born's rule.
What one learns from this is that a useful detector, loosely speaking, must be large enough so that superpositions of its pointer states rapidly decohere. Decoherence hence gives us a criterion for identifying detector pointer states by what Zurek termed 'einselection' by the environment [ 17 ].
But we do not observe mixed pointer states any more than we observe their superpositions. We only observe detectors in pointer states. In terms of the density matrix, we observe a matrix that has one entry equalling one, somewhere on the diagonal, and all other entries equal zero. The result of decoherence, however, is a density matrix with the Born probabilities on the diagonal.
Thus, while decoherence explains why we do not measure cats that are in a superposition of dead and alive, it does not explain why we do not measure cats that are 50% dead and 50% alive (a classical mixture) either [ 46 ]. To agree with observations, the wave function, or its density matrix, respectively, must therefore still be updated upon measurement.
Another way to see that decoherence does not solve the measurement problem is noting that it is based on counterfactual reasoning: the typical initial state of the system will, under the Schrödinger equation, evolve into a final state that is highly entangled with the environment, susceptible to decoherence, and hence not what we observe. According to the decoherence program, the state we observe is instead an (almost) decoherence-free subspace which is exactly what we generically do not expect. But the decoherence program gives us no clue as to how we get from evaluating the amount of decoherence in a state we do not observe to the not-decohering state we do observe.
This discrepancy raises the question of whether the notion of entropy we use in quantum mechanics, that increases under an evolution that we do not actually observe, can possibly be correct. Indeed, if the wave function describes an ensemble average, we do not expect a notion of entropy derived from it to be meaningful.
The decoherence program suffers from another problem, as pointed out by Kastner [ 47 ]. It requires one to specify a division between the observed system, the detector, and the environment already. Without that division, one does not know what the environment is that one should trace out. For this reason, decoherence does not allow us to define what a detector is. It merely quantifies certain properties that we know detectors do have.
We do not mean to deny the usefulness of studying and quantifying decoherence and entanglement. But they can ultimately not solve the problem of how to define a measurement, because these properties are basis-dependent. They will just reformulate the question into one about the choice of basis or the division into subsystems, respectively.
5.5. Many worlds
The many-worlds interpretation [ 48 , 49 ] and similar approaches are often claimed to be simpler than the Copenhagen Interpretation [ 50 ] because they do not require the Collapse Postulate. The fact that we only observe detector eigenstates is allegedly explained by the branching of the wave function and is supposedly a consequence of the Schrödinger equation alone.
Alas, this is just not the case. To make a prediction for a measurement outcome in a many-worlds approach, one has to replace the Collapse Postulate with sufficiently many assumptions that achieve the same. Those are normally stated as assumptions about what constitutes an observer or a detector or a branching event [ 51 ]; in any case, they are clearly not any simpler than the Collapse Postulate.
The easiest way to see that many worlds does not do away with the Collapse Postulate is to note that if it was possible to make predictions for our observations using the Schrödinger equation alone, then this would be possible in any interpretation of the mathematics. One therefore clearly needs the Collapse Postulate or at least equivalent assumptions in many worlds, regardless of how they are called or interpreted.
This is not to say that many world approaches are wrong. From the instrumentalist perspective, they are as good or as bad as the Copenhagen Interpretation. Anybody who doubts this statement is strongly encouraged to make a prediction with the many worlds interpretation and try to figure out how this differs from one made with the Copenhagen Interpretation.
5.6. Bohmian mechanics
Bohmian Mechanics [ 52 , 53 ] comes in two different versions, one in which the equilibrium hypothesis is counted as an axiom, and one in which it is not an axiom, but merely approximately fulfilled in the situations we typically observe in the laboratory.
Bohmian Mechanics with the equilibrium hypothesis is mathematically equivalent to the Copenhagen Interpretation in the sense that one can be derived from the other. They make exactly the same predictions. Bohmian Mechanics is usually formulated in position space, but one can easily extend this definition just by requiring it to respect invariance under basis transformations.
Since Bohmian Mechanics with the equilibrium hypothesis is equivalent to the Copenhagen Interpretation, it cannot solve the problems laid out in section 3 . It adds rather than removes non-locality, does not give us any clue about how to define a detector, and doesn't help us take a classical limit.
The reason Bohmian Mechanics is often said to solve the measurement problem is that the outcome of the time-evolution, interpreted suitably, is a detector eigenstate. In Bohmian Mechanics, one has a distribution of particles but interprets the actual ontic state of the system to be only one of them. Loosely speaking, Bohmian Mechanics combines the Schrödinger evolution and the Collapse Postulate to one local evolution for the particle and a non-local one for the guiding field. Since by assumption there is only one particle in the initial distribution, there is only one final outcome. 3
This solution however only works if one measures positions, so if one wants this solution to go through one has to argue that the only thing we ever measure are really positions of particles and everything else is derived from that. Given that the Collapse Postulate also brings the system into a detector eigenstate, one thus doesn't gain any advantage from switching to Bohmian Mechanics, one just gets this new headache. Further, since the ontology of Bohmian mechanics is itself non-local, it makes it even more difficult to conceive of a solution to the measurement problem.
Again, this is not to say that Bohmian Mechanics is wrong. Being equivalent to the Copenhagen Interpretation, it is isn't any better or worse: Nikolic proposed comparing Bohmian Mechanics to the Coulomb gauge of electrodynamics [ 54 ]. It seems non-local, and though it doesn't give rise to non-local observables, the explicit non-locality makes it difficult to generalise the formulation. It is quite possibly for this reason that quantum field theories based on Bohmian mechanics have been complete non-starters [ 55 , 56 ]. See also [ 57 ] for more about the difficulty of generalizing different interpretations of quantum mechanics to quantum field theory. Bohmian Mechanics may suffer from more severe problems than its failure to solve the measurement problem, see e.g. [ 58 – 60 ], but we will not discuss these here because it's not relevant for our purposes.
Bohmian Mechanics without the equilibrium hypothesis [ 61 , 62 ] is distinct from quantum mechanics, and to our best knowledge it has not helped solving the measurement problem. Since it has to reproduce the equilibrium hypothesis in the situations we typically encounter in the laboratory, it is also implausible that it would be of use.
5.7. Other interpretations
At this point, it should be clear that the problem can't be solved by reinterpreting the maths: we actually need new maths. If we cannot derive what a measurement device is, or what the source of gravity is in one interpretation, we can't do it in any interpretation. This means that QBism [ 63 – 65 ], the modal interpretation [ 66 ], the previously mentioned statistical interpretation [ 7 ], the transactional interpretation [ 67 , 68 ], Rovelli's relational interpretation [ 69 , 70 ], or Smolin's ensemble interpretation [ 71 ], or any other reinterpretation of the mathematics may in the best case make us feel better about quantum mechanics, but they can't actually solve the problems we laid out in section 3 . Those aren't just questions whose answers are difficult to calculate; they're questions whose answers can't be calculated in quantum mechanics—regardless of its interpretation.
We want to stress however that we certainly do not mean to say that it is useless to think about different interpretations of quantum mechanics. This is because some interpretations may make it easier to answer certain questions. A good example is the question of arrival time, a quantity that is notoriously difficult to calculate in the Copenhagen Interpretation, but that was recently successfully calculated using Bohmian Mechanics [ 72 ].
5.8. Collapse models
Collapse Models (be they gravitational, such as the Penrose-Diosi model, or spontaneous [ 73 ], such as the GRW [ 74 ] and CSL [ 75 ] models) have a chance to actually solve the problem, because they are not just reinterpreting the same mathematics. In accordance with what we discussed in 4.3 , they all bring in new parameters that quantify the deviation from standard quantum mechanics. However, the currently existing collapse models run into a well-known problem: Bell's theorem.
Remember that we can interpret any non-deterministic evolution as a deterministic evolution with additional hidden variables. This means, so long as collapse models fulfil the assumptions for Bell's theorem, they have to violate local causality (or they cannot reproduce observations). The currently used collapse models are therefore either still non-local [ 76 ], or the evolution law explicitly contains the basis that the evolution collapses into. In the latter case they either violate statistical independence, or one is forced to assume that measurements can only be made in one particular basis (usually the position basis).
6. The role of statistical independence
We have argued above that quantum mechanics suffers from several problems, and that any solution to the problem can be expressed as a local, deterministic theory with additional variables.
However, we can measure different observables of the same prepared state, and different observables correspond to different detector pointer states. If the evolution into the detector eigenstate is to be local, then the additional variables which determine the outcome must contain information about the pointer states from the outset. If the prepared state only gets this information by the time it arrives at the detector, then the collapse will generically have to be faster than light—this is exactly what happens in standard quantum mechanics.
If we want to avoid this, then the additional variables, commonly denoted λ , must be correlated with the measurement settings. This is known as a violation of statistical independence, or a violation of measurement independence. If ρ is the probability distribution of the hidden variables, and X are the detector settings (of possibly multiple detectors), then a violation of statistical independence means ρ ( λ ∣ X ) ≠ ρ ( λ ). Theories with this property have been dubbed superdeterministic [ 77 , 78 ].
To our best knowledge we do not presently have any theory that fulfills requirements 1–5 , but from our above arguments we do know that any such theory has to violate statistical independence. Seeing that most of what we argued above (except the reference to Frauchinger-Renner) could have been said 50 years ago, it is curious that progress on this has been so slow. We would like to offer some thoughts on why that may be.
One reason we don't yet have a solution to the problems of section 3 is almost certainly that so far it just wasn't necessary. Quantum mechanics in its present form has worked well, made a stunning amount of correct predictions, and for the most part saying that we know a detector when we see one is sufficient to make predictions with quantum mechanics' mathematical machine.
Let us not forget that it wasn't until 1982 [ 79 ] that violations of Bell's inequality were conclusively measured. Until about a decade ago, interpretations of quantum mechanics were discussed primarily by philosophers, simply because they weren't relevant for physicists. The re-approach between philosophy and physics in quantum foundations is a quite recent development, and it has been driven by technological advances.
Even now, the problems that we outlined in section 3 have grown of interest to the instrumentalist, but not yet for the experimentalist. This is about to change though. Soon, experiments will probe into the mesoscopic range, and investigate the question of just when a device ceases to be a useful detector. Further, tests of the weak-field limit of quantum gravity are on the way [ 80 , 81 ]—granted, the latter experiments weren't designed to test the Collapse Postulate, but they examine the parameter range where the questions raised above are also relevant.
Another likely reason why we haven't yet managed to solve the measurement problems is that the only viable option—violating statistical independence—was discarded 50 years ago on purely philosophical grounds. For peculiar reasons, statistical independence became referred to as the 'free will' or 'free choice' assumption, which has discouraged physicists from considering that this assumption may just not be fulfilled, and hence that a local description of the measurement process may still be possible. It has already been explained elsewhere [ 77 , 78 ] that this terminology is meaningless; whether statistical independence is violated or not bears no relevance for the existence of free will. The option of violating statistical independence was so strongly discouraged that as of today many physicists do not even know that Bell's theorem does not generally rule out local and deterministic completions with additional variables [ 82 ].
A third reason that probably added to the lack of interest in the option is that the additional variables are often interpreted as new degrees of freedom that reside inside elementary particles. This possibility is strongly constrained by experiments, and has only become more unappealing the more thoroughly we have tested the Standard Model of particle physics.
This, however, is based on a misunderstanding. The additional variables don't need to be new variables, and they don't need to reside on short distance scales; they merely need to be variables that don't appear in the standard axioms A1-A6. Further, as we mentioned above, the additional variables may merely be a stand-in for a non-deterministic (or non-computable) evolution law [ 83 ].
Most importantly though, there is no reason why the additional variables must be located inside particles or located anywhere for that matter. They could for example be the details of the detector or the environment or more generally variables that quantify large-scale properties or correlations. This means the solution we seek for may not to be found on the route of ontological reductionism that has preoccupied thinking in the foundations of physics for the past century (i.e., building bigger particle colliders won't solve this problem).
Indeed, as we have argued in [ 33 ], the additional variables in Bell's theorem are better interpreted as labels for trajectories (which also explains why they can alternatively be understood as encoding a non-deterministic evolution law). Whatever the reason for the slow progress, we think that the problems we have laid out here are eminently solvable with existing mathematics and will become accessible for experimental test in the near future.
7. What is it good for?
The brief answer to the question of what solving the measurement problem is good for (besides solving the problem, that is), is that we don't know. However, we can make some speculations.
For one thing, it might be that the underlying theory which solves the measurement problem turns out to be deterministic again, and explains the seeming indeterminism of quantum mechanics as being epistemic in origin. In this case, it stands to reason that the theory would allow us to overcome limits and bounds set by quantum mechanics on measurement accuracy with suitably configured experiments. This could turn out to be useful for many things, not least for quantum metrology and quantum computing.
However, it could turn out to go the other way. If deviations from the Schrödinger equation become important for, say, quantum computers beyond a certain number of qubits (as they are in Palmer's Invariant Set Theory [ 84 ]), then maybe large quantum computers will be impossible [ 85 , 86 ].
More generally, understanding in which cases a measurement process occurs and just what happens would almost certainly improve our ability to control quantum states.
That is to say, solving the measurement problem is not just a philosophical enterprise. Its solution will quite possibly have technological applications.
We have identified several different aspects of the measurement problem in quantum mechanics, and argued that to solve these problems we need a new theory. Deviations from quantum mechanics will likely become experimentally accessible in the near future. However, a theoretical understanding of the measurement process could greatly speed up the discovery. In a modest attempt to contribute to progress on the matter, we have listed five requirements that any satisfactory solution of the measurement problem must fulfil.
Acknowledgments
We thank Emily Adlam, Tim Palmer, Hrvoje Nikolic, and Ken Wharton for helpful discussions and feedback. JRH acknowledges support from the University of York's EPSRC DTP grant EP/R513386/1, and the EPSRC Quantum Communications Hub (funded by the EPSRC grants EP/M013472/1 and EP/T001011/1). SH acknowledges support by the Deutsche Forschungsgemeinschaft (DFG, German Research Foundation) under grant number HO 2601/8-1.
Data availability statement
No new data were created or analysed in this study.
Though this leaves one with the long-standing problem of the empty waves.
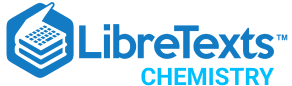
- school Campus Bookshelves
- menu_book Bookshelves
- perm_media Learning Objects
- login Login
- how_to_reg Request Instructor Account
- hub Instructor Commons
Margin Size
- Download Page (PDF)
- Download Full Book (PDF)
- Periodic Table
- Physics Constants
- Scientific Calculator
- Reference & Cite
- Tools expand_more
- Readability
selected template will load here
This action is not available.
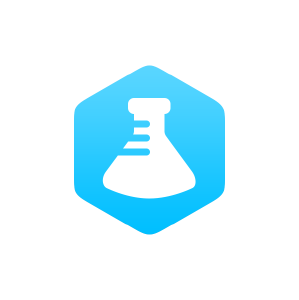
2: Measurement and Problem Solving
- Last updated
- Save as PDF
- Page ID 86009
Chemistry, like all sciences, is quantitative. It deals with quantities , things that have amounts and units. Dealing with quantities is very important in chemistry, as is relating quantities to each other. In this chapter, we will discuss how we deal with numbers and units, including how they are combined and manipulated.
- 2.1: Taking Measurements Chemists measure the properties of matter and express these measurements as quantities. A quantity is an amount of something and consists of a number and a unit. The number tells us how many (or how much), and the unit tells us what the scale of measurement is. For example, when a distance is reported as “5 kilometers,” we know that the quantity has been expressed in units of kilometers and that the number of kilometers is 5.
- 2.2: Scientific Notation - Writing Large and Small Numbers Chemists often work with numbers that are exceedingly large or small. For example, entering the mass in grams of a hydrogen atom into a calculator requires a display with at least 24 decimal places. A system called scientific notation avoids much of the tedium and awkwardness of manipulating numbers with large or small magnitudes.
- 2.3: Significant Figures - Writing Numbers to Reflect Precision Uncertainty exists in all measurements. The degree of uncertainty is affected in part by the quality of the measuring tool. Significant figures give an indication of the certainty of a measurement. Rules allow decisions to be made about how many digits to use in any given situation.
- 2.4: Significant Figures in Calculations To round a number, first decide how many significant figures the number should have. Once you know that, round to that many digits, starting from the left. If the number immediately to the right of the last significant digit is less than 5, it is dropped and the value of the last significant digit remains the same. If the number immediately to the right of the last significant digit is greater than or equal to 5, the last significant digit is increased by 1.
- 2.5: The Basic Units of Measurement Metric prefixes derive from Latin or Greek terms. The prefixes are used to make the units manageable. The SI system is based on multiples of ten. There are seven basic units in the SI system. Five of these units are commonly used in chemistry.
- 2.6: Problem Solving and Unit Conversions During your studies of chemistry (and physics also), you will note that mathematical equations are used in a number of different applications. Many of these equations have a number of different variables with which you will need to work. You should also note that these equations will often require you to use measurements with their units. Algebra skills become very important here!
- 2.7: Solving Multistep Conversion Problems Sometimes you will have to perform more than one conversion to obtain the desired unit.
- 2.8: Units Raised to a Power Conversion factors for area and volume can also be produced by the dimensional analysis method. Just remember that if a quantity is raised to a power of 10 both the number and the unit must be raised to the same power of 10.
- 2.9: Density Density is a physical property found by dividing the mass of an object by its volume. Regardless of the sample size, density is always constant.
- 2.E: Homework Chapter 2
- 2.E: Homework Chapter 2 Answers
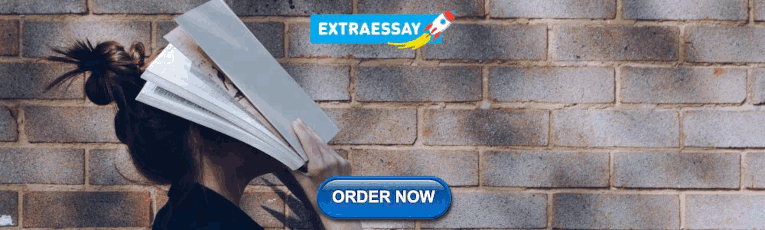
IMAGES
VIDEO
COMMENTS
This article for teachers suggests ways in which dinosaurs can be a great context for discussing measurement. Age 7 to 11 Problem Primary curriculum Secondary curriculum
Solution. To figure out how many kilometers he would run, you need to first add all of the lengths of the races together and then convert that measurement to kilometers. Use the factor label method and unit fractions to convert from meters to kilometers. Cancel, multiply, and solve. The runner would run 18 kilometers.
This page includes Measurement worksheets for length, area, angles, volume, capacity, mass, time and temperature in Metric, U.S. and Imperial units. Measurement concepts and skills give students the ability to perform tasks related to everyday life. Length, area, volume, capacity, mass, time and temperature are measurement concepts that people ...
Using place value to add 3-digit numbers: part 1. Using place value to add 3-digit numbers: part 2. Estimating when adding multi-digit numbers. Adding 3-digit numbers. Breaking apart 3-digit addition problems. Addition using groups of 10 and 100. Three digit addition word problems.
Converting units: centimeters to meters. (Opens a modal) Metric units of mass review (g and kg) (Opens a modal) Metric units of length review (mm, cm, m, & km) (Opens a modal) Metric units of volume review (L and mL) (Opens a modal) U.S. customary and metric units.
Measurement Problem-Solving. Measurement is the process of using a device or tool to find the dimensions, time, pressure, amount, weight or mass of an object. We use measurements to help us solve ...
Module 2: Unit conversions and problem solving with metric measurement: Quiz 2; Module 2: Unit conversions and problem solving with metric measurement: Unit test; About this unit "Module 2 focuses on length, mass, and capacity in the metric system where place value serves as a natural guide for moving between larger and smaller units." Eureka ...
2.E: Measurement and Problem Solving (Exercises) Exercises for Chapter 2 of Tro's Introductory Chemistry textmap. Chemistry, like all sciences, is quantitative. It concerns quantities, things that have amounts and units. Dealing with quantities and relating them to one another is very important in chemistry. In ….
Problem-solving Schools; About NRICH expand_more. About us; Impact stories; Support us; Our funders; Contact us; search; Site search search. Or search by topic. ... Measurement. Age 5 to 7. Estimating, Comparing, Measuring KS1. This collection of KS1 tasks combines the skills of estimating, comparing and measuring.
1.8: Solving Chemical Problems Measurements are made using a variety of units. It is often useful or necessary to convert a measured quantity from one unit into another. These conversions are accomplished using unit conversion factors, which are derived by simple applications of a mathematical approach called the factor-label method or ...
Cognitive ability test: A pre-employment aptitude test assesses individuals' abilities such as critical thinking, verbal reasoning, numerical ability, problem-solving, decision-making, etc., which are indicators of a person's intelligence quotient (IQ). The test results provide data about on-the-job performance.
A Maths article about solving problems involving height and length. ... The measurement for the height of the wall is in metres, but the measurements for each child's height is in centimetres. So ...
Measurements and Data. To solve length measurement problems, we need to have various factors in mind: the unit of measurement used, conversion exercises, and the understanding of how to use different measuring units. In this post, we'll present different exercise types that'll help us understand these concepts.
Solving Measurement Problems with Estimation. Notice that the line from Sally's to Eric's looks to be about 1/2 the length of the line from Sally's to Troy's house. This tells us that the distance ...
Module 2: Place value and problem solving with units of measure . Unit 3. Module 3: Multiplication and division with units of 0, 1, 6-9, and multiples of 10. Unit 4. Module 4: Multiplication and area. ... Module 7: Geometry and measurement word problems: Unit test; About this unit
Includes 8 problems. Problem Set UM2 - Unit Conversion 1. Use an understanding of metric prefixes to convert between metric units. Includes 7 problems, one of which involves temperature conversions. Problem Set UM3 - Unit Conversion 2. Perform 1-step or 2-step conversions to convert between metric and non-metric units. Includes 6 problems.
Use this resource to practice solving multi-step word problems involving measurement. This worksheet has two main scenarios, with each scenario having three questions. This works well when used as a math warm-up, a guided and independent practice, or as an exit ticket. Students need to be able to complete measurement conversions and multiply 2 ...
1 Define the problem. The first step in measuring your team's problem-solving skills is to clearly define the problem that needs to be solved. A well-defined problem should be specific, measurable ...
The brief answer to the question of what solving the measurement problem is good for (besides solving the problem, that is), is that we don't know. However, we can make some speculations. For one thing, it might be that the underlying theory which solves the measurement problem turns out to be deterministic again, and explains the seeming ...
Exercises for Chapter 2 of Tro's Introductory Chemistry textmap. 2: Measurement and Problem Solving is shared under a CC BY-NC-SA 4.0 license and was authored, remixed, and/or curated by LibreTexts. Chemistry, like all sciences, is quantitative. It deals with quantities, things that have amounts and units.
Solving measure problems at KS1. With these helpful worksheets, you can support your teaching of measure problems (KS1) with ease. Your students can learn how to solve addition and subtraction problems involving a range of different measurements, including: They're also useful for teaching your pupils measuring units such as metres, litres ...
When problem-solving progress as an executive is challenging to measure, using check metrics is crucial. Start by setting clear objectives and identifying key performance indicators (KPIs) related ...
1: Matter, Measurement, and Problem Solving is shared under a CC BY-NC-SA 4.0 license and was authored, remixed, and/or curated by LibreTexts. Back to top Licensing
OTP 107: Precision Optics & Mathematical Concepts (Covers basic algebraic operations, problem solving involving metric measurement, gears, pulleys, simple mechanism problems. Areas and volume calculations of geometric figures. Essentials of trigonometry for solving right and oblique triangles.) The program is optimized for students who want to ...
2.1: Taking Measurements. Chemists measure the properties of matter and express these measurements as quantities. A quantity is an amount of something and consists of a number and a unit. The number tells us how many (or how much), and the unit tells us what the scale of measurement is. For example, when a distance is reported as "5 ...