59 Ultrasound Essay Topic Ideas & Examples
🏆 best ultrasound topic ideas & essay examples, ✅ good essay topics on ultrasound, 📑 interesting topics to write about ultrasound.
- Use of Ultrasound-Guidance for Arterial Puncture All the anthropometric and demographic variables were recorded, as well as the main diagnosis of admission, comorbidities, the placement of the central venous catheter, and the course of the procedure.
- Benefits of 3D Ultrasound to Pregnant Mothers This is coherent to the 3D planar imaging are improved technology previously applied in the 2D ultrasound technology. As an extrapolation from 3D technology, 3D ultrasound is applied as a medical diagnostic technique that utilizes […]
- The Biological Effects of Ultrasound The paper also evaluates the physical mechanisms for the biological effects of ultrasound and the effects of ultrasound on living tissues in vivo and vitriol.
- The Recent Advances in Real Time Imaging in Ultrasound In point of fact, Medical imaging provides the most perfect task of diagnostic to Ultrasound, whereas, the main usage of therapeutic Ultrasound is to treat the numerous types of diseases and disorders in human beings.
- Comparison of MRI and Ultrasound for Determining Abnormalities in Preterm Infants Medical Imaging helps in detecting and diagnosing diseases at its earliest and treatable stage and helps in determining most appropriate and effective care for the patient.”Medical imaging provides a picture of the inside of the […]
- Ultrasound Techniques Applied to Body Fat Measurement in Male and Female The main objective of this paper is to evaluate the accuracy of body fat by using portable ultra sound device which results are reliable and authentic. The ultra sound technique is widely used to measure […]
- Benefits of 3D/4D Ultrasound in Prenatal Care The information that is obtained from this exam assists the health care providers in counseling parents on the development of the fetus especially in the nature of anomalies, prognosis, and the postnatal consideration of the […]
- Biologic Effects of Ultrasound in Healthcare Setting The instrument performing the emission of the sound waves and the recording of their bouncing back is referred to as the transducer and the medical practitioner generally gently presses the transducer against the skin of […]
- Ultrasound Scanning: Diagnosing Health Conditions The calculation is based on the time taken by the wave to return by measuring the distance, mass, nature, and stability of the object hit.
- Mammography vs. Ultrasound for Breast Tissue Analysis Mammography screening is one of the most recognized options for analyzing breast tissue in adult women. In contrast, the accuracy of this procedure allows it to be an alternative for women who cannot undergo mammography […]
- Ultrasound Physics and Instrumentation The camera is often not in harmony with the perception of the depth of a human vision. The level of such an acoustic signal distortion within a tissue is dependent on the emitted pulse’s amplitude […]
- Low-Back Pain and Ultrasound Therapy In the meantime, their opponents highlight that the beneficial aspects of the treatment course outweigh the risks related to the use of ultrasound equipment.
- Ultrasound in Treatment and Side-Effect Reduction Within the framework of the research project conducted by Ebadi et al, the research problem consisted in the fact that the effects of continuous ultrasound were underresearched.
- Ultrasound in Achilles Tendinitis Diagnosis In this research, the case study approach is applicable due to the fact that various patients suffering from tendon Achilles problem will be used as a basis for gauging the effectiveness of the method of […]
- Ultrasound Technology in Podiatry Surgery First, it is important to briefly outline the peculiarities of the RCT to understand the researchers’ point. They will be able to use the technology in numerous settings.
- Abdominal Ultrasound and Diagnoses The examiner explains to the patient how the procedure will be performed and how much time is necessary to finish the examination.
- Ultrasound and Color Doppler-Guided Surgery The purpose of the study is to examine the opinions of the trainees attending a training course concerning the use of technology.
- Contrast-Enhanced Ultrasound in Focal Liver Lesions In addition, inaccessibility to the eighth of the liver is a major setback in detecting lesions in the segment. With the advent of Doppler ultrasound, more insight in the diagnosis of liver lesions has been […]
- Ultrasound in Chemistry: Sonochemistry
- Intravascular Ultrasound: Current Role and Future Perspectives
- The Difference Between an Echocardiogram and an Ultrasound of the Heart
- Cooperative Control With Ultrasound Guidance for Radiation Therapy
- Optically Generated Ultrasound: A New Paradigm for Intracoronary Imaging
- Nondestructive Testing: Principle of Flaw Detection With Ultrasound
- Closed-Loop Transcranial Ultrasound Stimulation for Real-Time Non-invasive Neuromodulation
- Consumer Application of Ultrasound: A Television Remote
- Roles of Low-Intensity Ultrasound in Differentiating Cell Death
- High-Intensity Focused Ultrasound Development: Destroying the Target Tissue
- Using Ultrasound to Enhance the Mechanical and Physical Properties of Metals
- The Security Implications of the Machine-Learning Supply Chain: Professionalism in the Ultrasound Department
- Ultrasound Neuromodulation: Mechanisms and the Potential of Multimodal Stimulation for Neuronal Function Assessment
- How Ultrasound Can Produce Sonoluminescence
- Preparation for an Ultrasound of a Gallbladder and a Pelvic
- Endorectal and Endoanal Ultrasound Technique
- Transvaginal Ultrasound: Is It Painful, Purpose, and Results
- Endoscopic Ultrasound in Diagnosing Cancer: One of the Most Common Imaging Procedures
- Ultrasound Skin Imaging in Dermatology, Aesthetic Medicine, and Cosmetology
- Ultrasound Physical Medical Treatment in Healing Following an Acute Injury or a Chronic Condition
- How Do Ultrasound Scans Work
- Americas Ultrasound Systems Market: From USD 7.9 Billion to USD 10.23 Billion
- How Ultrasound Imaging Helps Us Understand Speech and Accent Variation
- Cerebral Ultrasound Time-Harmonic Elastography: Softening of the Human Brain Due to Dehydration
- Wireless Communication in “Audio Beacons” Using Ultrasound
- Low-Intensity Focused Ultrasound for Posttraumatic Stress Disorder
- Thyroid Ultrasound: Purpose, Procedure, Benefits
- Blood Pressure Modulation With Low-Intensity Focused Ultrasound
- Accuracy of Ultrasounds in Diagnosing Birth Defects
- Functional Ultrasound During Awake Brain Surgery
- Live Animal Ultrasound Explained by Dr. Allen Williams
- Ultrasound Waves in Acoustic Microscopy
- Chemical and Physical Effects of Ultrasound: Sonoluminescence and Materials
- Focused Ultrasound for Noninvasive, Focal Pharmacologic Neurointervention
- Lung Ultrasound Findings in COVID-19 Pneumonia
- High-Power Ultrasound in Dry Corn Milling Plants
- History of Ultrasound of Physics and the Properties of the Transducer
- Relationship Between Ultrasound Viewing and Proceeding to Abortion
- Transrectal Ultrasound of the Prostate With a Biopsy
- Iron-Based Catalysts Used in Water Treatment Assisted by Ultrasound
- Epigenetics Essay Titles
- Hypertension Topics
- Biomedicine Essay Topics
- Deontology Questions
- Breast Cancer Ideas
- Health Insurance Research Topics
- Evidence-Based Practice Titles
- Healthcare Questions
- Chicago (A-D)
- Chicago (N-B)
IvyPanda. (2023, January 24). 59 Ultrasound Essay Topic Ideas & Examples. https://ivypanda.com/essays/topic/ultrasound-essay-topics/
"59 Ultrasound Essay Topic Ideas & Examples." IvyPanda , 24 Jan. 2023, ivypanda.com/essays/topic/ultrasound-essay-topics/.
IvyPanda . (2023) '59 Ultrasound Essay Topic Ideas & Examples'. 24 January.
IvyPanda . 2023. "59 Ultrasound Essay Topic Ideas & Examples." January 24, 2023. https://ivypanda.com/essays/topic/ultrasound-essay-topics/.
1. IvyPanda . "59 Ultrasound Essay Topic Ideas & Examples." January 24, 2023. https://ivypanda.com/essays/topic/ultrasound-essay-topics/.
Bibliography
IvyPanda . "59 Ultrasound Essay Topic Ideas & Examples." January 24, 2023. https://ivypanda.com/essays/topic/ultrasound-essay-topics/.
Thank you for visiting nature.com. You are using a browser version with limited support for CSS. To obtain the best experience, we recommend you use a more up to date browser (or turn off compatibility mode in Internet Explorer). In the meantime, to ensure continued support, we are displaying the site without styles and JavaScript.
- View all journals
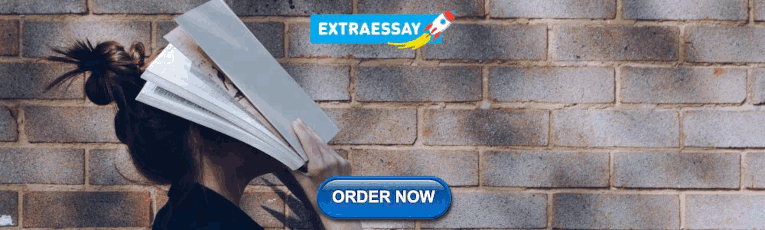
Ultrasonography articles from across Nature Portfolio
Ultrasonography is an imaging technique that uses the reflections of high-frequency sound waves to create an image of a structure located within the body.
Related Subjects
- Echocardiography
Latest Research and Reviews
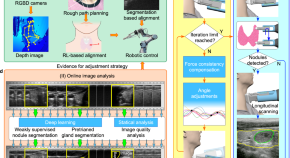
A fully autonomous robotic ultrasound system for thyroid scanning
Current thyroid ultrasounds rely heavily on the experience and skills of the sonographer and of the radiologist, and the process is physically and cognitively exhausting. Here, the authors show a fully autonomous robotic ultrasound system, which is able to scan thyroid regions without human assistance and identify malignant nodules.
- Jingwei Liu
- Peter Xiaoping Liu
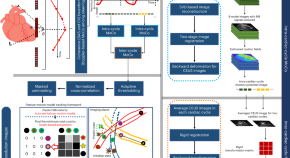
Transthoracic ultrasound localization microscopy of myocardial vasculature in patients
Transthoracic ultrasound localization microscopy enables super-resolution imaging of myocardial microvasculature and haemodynamics in patients with impaired myocardial function using data acquired within a breath hold.
- Meng-Xing Tang
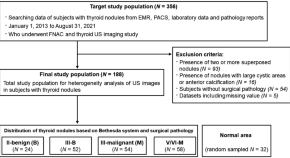
Differential diagnosis of thyroid nodules using heterogeneity quantification software on ultrasound images: correlation with the Bethesda system and surgical pathology
- Young Jae Ryu
- Jin Woong Kim
- Tae-Hoon Kim
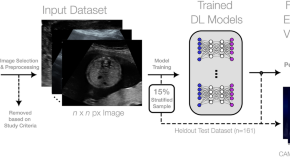
Deep learning prediction of renal anomalies for prenatal ultrasound diagnosis
- Olivier X. Miguel
- Emily Kaczmarek
- Mark C. Walker
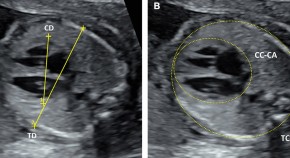
Comparing three cardiothoracic ratio measurement techniques and creating multivariable scoring system to predict Bart’s hydrops fetalis at 17–22 weeks’ gestation
- Sanitra Anuwutnavin
- Patsawee Rangseechamrat
- Sommai Viboonchard
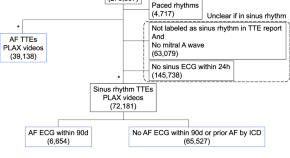
Deep learning evaluation of echocardiograms to identify occult atrial fibrillation
- Nathan R. Stein
- David Ouyang
News and Comment
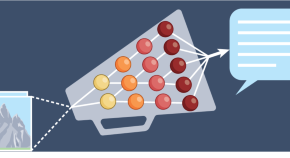
Adapting vision–language AI models to cardiology tasks
Vision–language models can be trained to read cardiac ultrasound images with implications for improving clinical workflows, but additional development and validation will be required before such models can replace humans.
- Rima Arnaout
Factors identified that predict resolution of subclinical synovitis
New findings provide insight into the natural history of subclinical synovitis, a reported predictor of the development of rheumatoid arthritis, and identify various factors associated with its reversal.
- Jessica McHugh
AI outperforms sonographers at diagnosing cardiac function on echocardiography
An artificial intelligence-guided workflow for initial evaluation of left ventricular ejection fraction in echocardiography is non-inferior to initial assessment by a sonographer, according to findings from a blinded, randomized, non-inferiority clinical trial.
- Irene Fernández-Ruiz
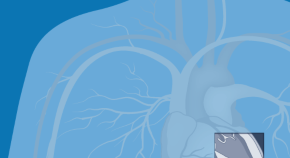
A wearable ultrasonic device to image cardiac function
Researchers have engineered a wearable device that adheres to the skin and uses ultrasound imaging and a deep learning model to produce a dynamic, real-time assessment of cardiac function.
- Gregory B. Lim
Secukinumab reduces synovitis in PsA
- Sarah Onuora

Deep learning for detecting congenital heart disease in the fetus
New advances in machine learning could facilitate and reduce disparities in the prenatal diagnosis of congenital health disease, the most common and lethal birth defect.
- Shaine A. Morris
- Keila N. Lopez
Quick links
- Explore articles by subject
- Guide to authors
- Editorial policies


An official website of the United States government
The .gov means it’s official. Federal government websites often end in .gov or .mil. Before sharing sensitive information, make sure you’re on a federal government site.
The site is secure. The https:// ensures that you are connecting to the official website and that any information you provide is encrypted and transmitted securely.
- Publications
- Account settings
Preview improvements coming to the PMC website in October 2024. Learn More or Try it out now .
- Advanced Search
- Journal List
- Ultrasonography
- v.37(1); 2018 Jan
Ultrasonography: current status, challenges, and future directions
Since Ultrasonography was launched as an international peer-reviewed academic journal dedicated to medical ultrasound (US) in January 2014, it has been an honor and privilege for me to serve as the journal’s Honorary Editor [ 1 ]. The purpose of this editorial update is to provide readers with some recent information and to share my views on the journal and its future directions.
During the last 4 years, Ultrasonography has published a total of 160 peer-reviewed articles. In 2017, the Ultrasonography home page had over 85,000 hits per month, with over 2,300 downloads of manuscripts from 115 different countries per month. In 2016, Ultrasonography demonstrated an increase in the number of citations by authors throughout the world, and the estimated impact factor of Ultrasonography reached 2.075 [ 2 ], as it has continued to attract more and more international readers. Consequently, Ultrasonography became indexed by the Web of Science’s new Emerging Source Citation Index (ESCI) in November 2016 [ 2 ] and by SCOPUS in September 2017, marking it as one of the leading global journals in the field of medical US.
The aim of Ultrasonography is to provide up-to-date information on new diagnostic concepts and technical developments, as well as to publish well-designed reviews of contemporary issues in patient care in the field of medical US. As the Honorary Editor of Ultrasonography , I am aware of the editor’s tremendous responsibility in guiding the publication to fulfill the aims of Ultrasonography and of how important the roles of the authors, reviewers, and readers are for a journal to be successful.
Those of us who are involved in the journal’s publication have the great responsibility to provide an effective means to disseminate the original work of the authors as well as to provide timely, high-quality research to readers. We will continue to promote and publish high-quality peer-reviewed research. We also plan to invite internationally renowned scholars to submit reviews of the latest research and introductions to innovative new technologies to enhance the quality of the content and to attract a larger international readership. Original articles that advance the science of US will remain the centerpiece of the journal, while Pictorial Essays, Technical Notes, and Perspectives will continue to be published. Sonologists and US-related specialists are encouraged to submit their most promising research results to Ultrasonography . We will continue to provide high-quality editing and publishing services without cost, and there will be no fees for submissions or for the publication process.
A quality publication must have capable, fair, and considerate reviewers, as well as outstanding authors and manuscripts. The adoption of a double-blind peer review process and an open-access policy will enable Ultrasonography to fulfill its obligation to publication ethics; each article must also comply with our editorial standards for quality and ethics. We have thus established an Editorial Board composed of internationally renowned scholars and scientists to meet global standards for the process of peer-reviewing submitted manuscripts. We are grateful to the many reviewers and editorial members who volunteer their time and effort to ensure the quality of the journal’s content. The speed of publication is also important for the journal’s success. We have established a state-of-the-art electronic manuscript submission, review, and electronic publishing system, and we make our best effort to complete the review process as soon as possible, ideally within 4 weeks of receiving a manuscript submission.
Going forward, I believe Ultrasonography will be a useful and valuable international journal covering recent advances in US for every US-related specialist. I look forward to receiving a wide range of submissions and contributions from basic and clinical researchers throughout the world.
- Frontiers in Acoustics
- Ultrasound Technologies
- Research Topics
Insights in Ultrasound Technologies: 2024
Total Downloads
Total Views and Downloads
About this Research Topic
We are now entering the third decade of the 21st Century, and, especially in the last years, the achievements made by scientists have been exceptional, leading to major advancements in the fast-growing field of acoustics. This editorial initiative of particular relevance, led by Professor Yun Jing, Specialty Chief Editor of the Ultrasound Technologies section, is focused on new insights, novel developments, current challenges, latest discoveries, recent advances and future perspectives in the field of ultrasound technologies. The Research Topic solicits brief, forward-looking contributions that describe the state of the art, recent developments and major accomplishments achieved and that need to occur to move the field forward. Authors are encouraged to identify the greatest challenges in the sub-disciplines, and how to address those challenges. The goal of this special edition Research Topic is to shed light on the progress made during the past decade within the broad and multidisciplinary field of ultrasound technologies and on any perceived future challenges associated with this to provide a thorough overview of the status of the field. This article collection will inspire, inform, and provide direction and guidance to researchers in the field.
Keywords : medical ultrasound imaging, therapeutic ultrasound, non-destructive evaluation, nonlinear ultrasonics, physics of ultrasound, underwater ultrasound, ultrasonic transducers
Important Note : All contributions to this Research Topic must be within the scope of the section and journal to which they are submitted, as defined in their mission statements. Frontiers reserves the right to guide an out-of-scope manuscript to a more suitable section or journal at any stage of peer review.
Topic Editors
Topic coordinators, submission deadlines.
Manuscript |
Participating Journals
Manuscripts can be submitted to this Research Topic via the following journals:
total views
- Demographics
No records found
total views article views downloads topic views
Top countries
Top referring sites, about frontiers research topics.
With their unique mixes of varied contributions from Original Research to Review Articles, Research Topics unify the most influential researchers, the latest key findings and historical advances in a hot research area! Find out more on how to host your own Frontiers Research Topic or contribute to one as an author.
Open Access is an initiative that aims to make scientific research freely available to all. To date our community has made over 100 million downloads. It’s based on principles of collaboration, unobstructed discovery, and, most importantly, scientific progression. As PhD students, we found it difficult to access the research we needed, so we decided to create a new Open Access publisher that levels the playing field for scientists across the world. How? By making research easy to access, and puts the academic needs of the researchers before the business interests of publishers.
We are a community of more than 103,000 authors and editors from 3,291 institutions spanning 160 countries, including Nobel Prize winners and some of the world’s most-cited researchers. Publishing on IntechOpen allows authors to earn citations and find new collaborators, meaning more people see your work not only from your own field of study, but from other related fields too.
Brief introduction to this section that descibes Open Access especially from an IntechOpen perspective
Want to get in touch? Contact our London head office or media team here
Our team is growing all the time, so we’re always on the lookout for smart people who want to help us reshape the world of scientific publishing.
Home > Books > Medical Imaging
Ultrasound Imaging - Current Topics

Book metrics overview
4,212 Chapter Downloads
Impact of this book and its chapters
Total Chapter Downloads on intechopen.com
Total Chapter Citations
Academic Editor
Gregory University , Nigeria
Published 11 May 2022
Doi 10.5772/intechopen.95178
ISBN 978-1-78985-186-1
Print ISBN 978-1-78984-877-9
eBook (PDF) ISBN 978-1-78985-331-5
Copyright year 2022
Number of pages 154
Ultrasound Imaging - Current Topics presents complex and current topics in ultrasound imaging in a simplified format. It is easy to read and exemplifies the range of experiences of each contributing author. Chapters address such topics as anatomy and dimensional variations, pediatric gastrointestinal emergencies, musculoskeletal and nerve imaging as well as molecular sonography. The book is...
Ultrasound Imaging - Current Topics presents complex and current topics in ultrasound imaging in a simplified format. It is easy to read and exemplifies the range of experiences of each contributing author. Chapters address such topics as anatomy and dimensional variations, pediatric gastrointestinal emergencies, musculoskeletal and nerve imaging as well as molecular sonography. The book is a useful resource for researchers, students, clinicians, and sonographers looking for additional information on ultrasound imaging beyond the basics.
By submitting the form you agree to IntechOpen using your personal information in order to fulfil your library recommendation. In line with our privacy policy we won’t share your details with any third parties and will discard any personal information provided immediately after the recommended institution details are received. For further information on how we protect and process your personal information, please refer to our privacy policy .
Cite this book
There are two ways to cite this book:
Edited Volume and chapters are indexed in
Table of contents.
By Solomon Demissie, Mulatie Atalay and Yonas Derso
By Ercan Ayaz
By Haithem Zaafouri, Meryam Mesbahi, Nizar Khedhiri, Wassim Riahi, Mouna Cherif, Dhafer Haddad and Anis Ben Maamer
By Felix Okechukwu Erondu
By Stefan Cristian Dinescu, Razvan Adrian Ionescu, Horatiu Valeriu Popoviciu, Claudiu Avram and Florentin Ananu Vreju
By María Eugenia Aponte-Rueda and María Isabel de Abreu
By Jong Hwa Lee, Jae Uk Lee and Seung Wan Yoo
By J.M. López Álvarez, O. Pérez Quevedo, S. Alonso-Graña López-Manteola, J. Naya Esteban, J.F. Loro Ferrer and D.L. Lorenzo Villegas
By Arthur Fleischer and Sai Chennupati
IMPACT OF THIS BOOK AND ITS CHAPTERS
4,212 Total Chapter Downloads
1 Crossref Citations
2 Dimensions Citations
Order a print copy of this book
Available on

Delivered by
£119 (ex. VAT)*
Hardcover | Printed Full Colour
FREE SHIPPING WORLDWIDE
* Residents of European Union countries need to add a Book Value-Added Tax Rate based on their country of residence. Institutions and companies, registered as VAT taxable entities in their own EU member state, will not pay VAT by providing IntechOpen with their VAT registration number. This is made possible by the EU reverse charge method.
As an IntechOpen contributor, you can buy this book for an Exclusive Author price with discounts from 30% to 50% on retail price.
Log in to your Author Panel to purchase a book at the discounted price.
For any assistance during ordering process, contact us at [email protected]
Related books
Medical imaging.
Edited by Felix Okechukwu Erondu
Medical Imaging in Clinical Practice
Medical and biological image analysis.
Edited by Robert Koprowski
Edited by Yongxia Zhou
Ultrasound Elastography
Edited by Monica Lupsor Platon
New Advances in Magnetic Resonance Imaging
Edited by Denis Larrivee
Frontiers in Neuroimaging
Edited by Xianli Lv
Optical Coherence Tomography
Edited by Giuseppe Lo Giudice
Updates in Endoscopy
Edited by Somchai Amornyotin
Elastography
Edited by Dana Stoian
Call for authors
Submit your work to intechopen.


Head Start Your Radiology Residency [Online] ↗️
- Radiology Thesis – More than 400 Research Topics (2022)!
Please login to bookmark
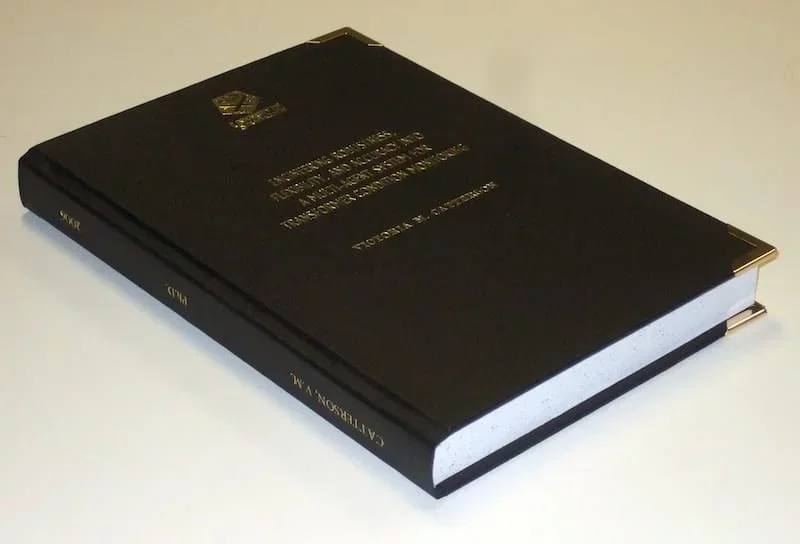
Introduction
A thesis or dissertation, as some people would like to call it, is an integral part of the Radiology curriculum, be it MD, DNB, or DMRD. We have tried to aggregate radiology thesis topics from various sources for reference.
Not everyone is interested in research, and writing a Radiology thesis can be daunting. But there is no escape from preparing, so it is better that you accept this bitter truth and start working on it instead of cribbing about it (like other things in life. #PhilosophyGyan!)
Start working on your thesis as early as possible and finish your thesis well before your exams, so you do not have that stress at the back of your mind. Also, your thesis may need multiple revisions, so be prepared and allocate time accordingly.
Tips for Choosing Radiology Thesis and Research Topics
Keep it simple silly (kiss).
Retrospective > Prospective
Retrospective studies are better than prospective ones, as you already have the data you need when choosing to do a retrospective study. Prospective studies are better quality, but as a resident, you may not have time (, energy and enthusiasm) to complete these.
Choose a simple topic that answers a single/few questions
Original research is challenging, especially if you do not have prior experience. I would suggest you choose a topic that answers a single or few questions. Most topics that I have listed are along those lines. Alternatively, you can choose a broad topic such as “Role of MRI in evaluation of perianal fistulas.”
You can choose a novel topic if you are genuinely interested in research AND have a good mentor who will guide you. Once you have done that, make sure that you publish your study once you are done with it.
Get it done ASAP.
In most cases, it makes sense to stick to a thesis topic that will not take much time. That does not mean you should ignore your thesis and ‘Ctrl C + Ctrl V’ from a friend from another university. Thesis writing is your first step toward research methodology so do it as sincerely as possible. Do not procrastinate in preparing the thesis. As soon as you have been allotted a guide, start researching topics and writing a review of the literature.
At the same time, do not invest a lot of time in writing/collecting data for your thesis. You should not be busy finishing your thesis a few months before the exam. Some people could not appear for the exam because they could not submit their thesis in time. So DO NOT TAKE thesis lightly.
Do NOT Copy-Paste
Reiterating once again, do not simply choose someone else’s thesis topic. Find out what are kind of cases that your Hospital caters to. It is better to do a good thesis on a common topic than a crappy one on a rare one.
Books to help you write a Radiology Thesis
Event country/university has a different format for thesis; hence these book recommendations may not work for everyone.

- Amazon Kindle Edition
- Gupta, Piyush (Author)
- English (Publication Language)
- 206 Pages - 10/12/2020 (Publication Date) - Jaypee Brothers Medical Publishers (P) Ltd. (Publisher)
In A Hurry? Download a PDF list of Radiology Research Topics!
Sign up below to get this PDF directly to your email address.
100% Privacy Guaranteed. Your information will not be shared. Unsubscribe anytime with a single click.
List of Radiology Research /Thesis / Dissertation Topics
- State of the art of MRI in the diagnosis of hepatic focal lesions
- Multimodality imaging evaluation of sacroiliitis in newly diagnosed patients of spondyloarthropathy
- Multidetector computed tomography in oesophageal varices
- Role of positron emission tomography with computed tomography in the diagnosis of cancer Thyroid
- Evaluation of focal breast lesions using ultrasound elastography
- Role of MRI diffusion tensor imaging in the assessment of traumatic spinal cord injuries
- Sonographic imaging in male infertility
- Comparison of color Doppler and digital subtraction angiography in occlusive arterial disease in patients with lower limb ischemia
- The role of CT urography in Haematuria
- Role of functional magnetic resonance imaging in making brain tumor surgery safer
- Prediction of pre-eclampsia and fetal growth restriction by uterine artery Doppler
- Role of grayscale and color Doppler ultrasonography in the evaluation of neonatal cholestasis
- Validity of MRI in the diagnosis of congenital anorectal anomalies
- Role of sonography in assessment of clubfoot
- Role of diffusion MRI in preoperative evaluation of brain neoplasms
- Imaging of upper airways for pre-anaesthetic evaluation purposes and for laryngeal afflictions.
- A study of multivessel (arterial and venous) Doppler velocimetry in intrauterine growth restriction
- Multiparametric 3tesla MRI of suspected prostatic malignancy.
- Role of Sonography in Characterization of Thyroid Nodules for differentiating benign from
- Role of advances magnetic resonance imaging sequences in multiple sclerosis
- Role of multidetector computed tomography in evaluation of jaw lesions
- Role of Ultrasound and MR Imaging in the Evaluation of Musculotendinous Pathologies of Shoulder Joint
- Role of perfusion computed tomography in the evaluation of cerebral blood flow, blood volume and vascular permeability of cerebral neoplasms
- MRI flow quantification in the assessment of the commonest csf flow abnormalities
- Role of diffusion-weighted MRI in evaluation of prostate lesions and its histopathological correlation
- CT enterography in evaluation of small bowel disorders
- Comparison of perfusion magnetic resonance imaging (PMRI), magnetic resonance spectroscopy (MRS) in and positron emission tomography-computed tomography (PET/CT) in post radiotherapy treated gliomas to detect recurrence
- Role of multidetector computed tomography in evaluation of paediatric retroperitoneal masses
- Role of Multidetector computed tomography in neck lesions
- Estimation of standard liver volume in Indian population
- Role of MRI in evaluation of spinal trauma
- Role of modified sonohysterography in female factor infertility: a pilot study.
- The role of pet-CT in the evaluation of hepatic tumors
- Role of 3D magnetic resonance imaging tractography in assessment of white matter tracts compromise in supratentorial tumors
- Role of dual phase multidetector computed tomography in gallbladder lesions
- Role of multidetector computed tomography in assessing anatomical variants of nasal cavity and paranasal sinuses in patients of chronic rhinosinusitis.
- magnetic resonance spectroscopy in multiple sclerosis
- Evaluation of thyroid nodules by ultrasound elastography using acoustic radiation force impulse (ARFI) imaging
- Role of Magnetic Resonance Imaging in Intractable Epilepsy
- Evaluation of suspected and known coronary artery disease by 128 slice multidetector CT.
- Role of regional diffusion tensor imaging in the evaluation of intracranial gliomas and its histopathological correlation
- Role of chest sonography in diagnosing pneumothorax
- Role of CT virtual cystoscopy in diagnosis of urinary bladder neoplasia
- Role of MRI in assessment of valvular heart diseases
- High resolution computed tomography of temporal bone in unsafe chronic suppurative otitis media
- Multidetector CT urography in the evaluation of hematuria
- Contrast-induced nephropathy in diagnostic imaging investigations with intravenous iodinated contrast media
- Comparison of dynamic susceptibility contrast-enhanced perfusion magnetic resonance imaging and single photon emission computed tomography in patients with little’s disease
- Role of Multidetector Computed Tomography in Bowel Lesions.
- Role of diagnostic imaging modalities in evaluation of post liver transplantation recipient complications.
- Role of multislice CT scan and barium swallow in the estimation of oesophageal tumour length
- Malignant Lesions-A Prospective Study.
- Value of ultrasonography in assessment of acute abdominal diseases in pediatric age group
- Role of three dimensional multidetector CT hysterosalpingography in female factor infertility
- Comparative evaluation of multi-detector computed tomography (MDCT) virtual tracheo-bronchoscopy and fiberoptic tracheo-bronchoscopy in airway diseases
- Role of Multidetector CT in the evaluation of small bowel obstruction
- Sonographic evaluation in adhesive capsulitis of shoulder
- Utility of MR Urography Versus Conventional Techniques in Obstructive Uropathy
- MRI of the postoperative knee
- Role of 64 slice-multi detector computed tomography in diagnosis of bowel and mesenteric injury in blunt abdominal trauma.
- Sonoelastography and triphasic computed tomography in the evaluation of focal liver lesions
- Evaluation of Role of Transperineal Ultrasound and Magnetic Resonance Imaging in Urinary Stress incontinence in Women
- Multidetector computed tomographic features of abdominal hernias
- Evaluation of lesions of major salivary glands using ultrasound elastography
- Transvaginal ultrasound and magnetic resonance imaging in female urinary incontinence
- MDCT colonography and double-contrast barium enema in evaluation of colonic lesions
- Role of MRI in diagnosis and staging of urinary bladder carcinoma
- Spectrum of imaging findings in children with febrile neutropenia.
- Spectrum of radiographic appearances in children with chest tuberculosis.
- Role of computerized tomography in evaluation of mediastinal masses in pediatric
- Diagnosing renal artery stenosis: Comparison of multimodality imaging in diabetic patients
- Role of multidetector CT virtual hysteroscopy in the detection of the uterine & tubal causes of female infertility
- Role of multislice computed tomography in evaluation of crohn’s disease
- CT quantification of parenchymal and airway parameters on 64 slice MDCT in patients of chronic obstructive pulmonary disease
- Comparative evaluation of MDCT and 3t MRI in radiographically detected jaw lesions.
- Evaluation of diagnostic accuracy of ultrasonography, colour Doppler sonography and low dose computed tomography in acute appendicitis
- Ultrasonography , magnetic resonance cholangio-pancreatography (MRCP) in assessment of pediatric biliary lesions
- Multidetector computed tomography in hepatobiliary lesions.
- Evaluation of peripheral nerve lesions with high resolution ultrasonography and colour Doppler
- Multidetector computed tomography in pancreatic lesions
- Multidetector Computed Tomography in Paediatric abdominal masses.
- Evaluation of focal liver lesions by colour Doppler and MDCT perfusion imaging
- Sonographic evaluation of clubfoot correction during Ponseti treatment
- Role of multidetector CT in characterization of renal masses
- Study to assess the role of Doppler ultrasound in evaluation of arteriovenous (av) hemodialysis fistula and the complications of hemodialysis vasular access
- Comparative study of multiphasic contrast-enhanced CT and contrast-enhanced MRI in the evaluation of hepatic mass lesions
- Sonographic spectrum of rheumatoid arthritis
- Diagnosis & staging of liver fibrosis by ultrasound elastography in patients with chronic liver diseases
- Role of multidetector computed tomography in assessment of jaw lesions.
- Role of high-resolution ultrasonography in the differentiation of benign and malignant thyroid lesions
- Radiological evaluation of aortic aneurysms in patients selected for endovascular repair
- Role of conventional MRI, and diffusion tensor imaging tractography in evaluation of congenital brain malformations
- To evaluate the status of coronary arteries in patients with non-valvular atrial fibrillation using 256 multirow detector CT scan
- A comparative study of ultrasonography and CT – arthrography in diagnosis of chronic ligamentous and meniscal injuries of knee
- Multi detector computed tomography evaluation in chronic obstructive pulmonary disease and correlation with severity of disease
- Diffusion weighted and dynamic contrast enhanced magnetic resonance imaging in chemoradiotherapeutic response evaluation in cervical cancer.
- High resolution sonography in the evaluation of non-traumatic painful wrist
- The role of trans-vaginal ultrasound versus magnetic resonance imaging in diagnosis & evaluation of cancer cervix
- Role of multidetector row computed tomography in assessment of maxillofacial trauma
- Imaging of vascular complication after liver transplantation.
- Role of magnetic resonance perfusion weighted imaging & spectroscopy for grading of glioma by correlating perfusion parameter of the lesion with the final histopathological grade
- Magnetic resonance evaluation of abdominal tuberculosis.
- Diagnostic usefulness of low dose spiral HRCT in diffuse lung diseases
- Role of dynamic contrast enhanced and diffusion weighted magnetic resonance imaging in evaluation of endometrial lesions
- Contrast enhanced digital mammography anddigital breast tomosynthesis in early diagnosis of breast lesion
- Evaluation of Portal Hypertension with Colour Doppler flow imaging and magnetic resonance imaging
- Evaluation of musculoskeletal lesions by magnetic resonance imaging
- Role of diffusion magnetic resonance imaging in assessment of neoplastic and inflammatory brain lesions
- Radiological spectrum of chest diseases in HIV infected children High resolution ultrasonography in neck masses in children
- with surgical findings
- Sonographic evaluation of peripheral nerves in type 2 diabetes mellitus.
- Role of perfusion computed tomography in the evaluation of neck masses and correlation
- Role of ultrasonography in the diagnosis of knee joint lesions
- Role of ultrasonography in evaluation of various causes of pelvic pain in first trimester of pregnancy.
- Role of Magnetic Resonance Angiography in the Evaluation of Diseases of Aorta and its Branches
- MDCT fistulography in evaluation of fistula in Ano
- Role of multislice CT in diagnosis of small intestine tumors
- Role of high resolution CT in differentiation between benign and malignant pulmonary nodules in children
- A study of multidetector computed tomography urography in urinary tract abnormalities
- Role of high resolution sonography in assessment of ulnar nerve in patients with leprosy.
- Pre-operative radiological evaluation of locally aggressive and malignant musculoskeletal tumours by computed tomography and magnetic resonance imaging.
- The role of ultrasound & MRI in acute pelvic inflammatory disease
- Ultrasonography compared to computed tomographic arthrography in the evaluation of shoulder pain
- Role of Multidetector Computed Tomography in patients with blunt abdominal trauma.
- The Role of Extended field-of-view Sonography and compound imaging in Evaluation of Breast Lesions
- Evaluation of focal pancreatic lesions by Multidetector CT and perfusion CT
- Evaluation of breast masses on sono-mammography and colour Doppler imaging
- Role of CT virtual laryngoscopy in evaluation of laryngeal masses
- Triple phase multi detector computed tomography in hepatic masses
- Role of transvaginal ultrasound in diagnosis and treatment of female infertility
- Role of ultrasound and color Doppler imaging in assessment of acute abdomen due to female genetal causes
- High resolution ultrasonography and color Doppler ultrasonography in scrotal lesion
- Evaluation of diagnostic accuracy of ultrasonography with colour Doppler vs low dose computed tomography in salivary gland disease
- Role of multidetector CT in diagnosis of salivary gland lesions
- Comparison of diagnostic efficacy of ultrasonography and magnetic resonance cholangiopancreatography in obstructive jaundice: A prospective study
- Evaluation of varicose veins-comparative assessment of low dose CT venogram with sonography: pilot study
- Role of mammotome in breast lesions
- The role of interventional imaging procedures in the treatment of selected gynecological disorders
- Role of transcranial ultrasound in diagnosis of neonatal brain insults
- Role of multidetector CT virtual laryngoscopy in evaluation of laryngeal mass lesions
- Evaluation of adnexal masses on sonomorphology and color Doppler imaginig
- Role of radiological imaging in diagnosis of endometrial carcinoma
- Comprehensive imaging of renal masses by magnetic resonance imaging
- The role of 3D & 4D ultrasonography in abnormalities of fetal abdomen
- Diffusion weighted magnetic resonance imaging in diagnosis and characterization of brain tumors in correlation with conventional MRI
- Role of diffusion weighted MRI imaging in evaluation of cancer prostate
- Role of multidetector CT in diagnosis of urinary bladder cancer
- Role of multidetector computed tomography in the evaluation of paediatric retroperitoneal masses.
- Comparative evaluation of gastric lesions by double contrast barium upper G.I. and multi detector computed tomography
- Evaluation of hepatic fibrosis in chronic liver disease using ultrasound elastography
- Role of MRI in assessment of hydrocephalus in pediatric patients
- The role of sonoelastography in characterization of breast lesions
- The influence of volumetric tumor doubling time on survival of patients with intracranial tumours
- Role of perfusion computed tomography in characterization of colonic lesions
- Role of proton MRI spectroscopy in the evaluation of temporal lobe epilepsy
- Role of Doppler ultrasound and multidetector CT angiography in evaluation of peripheral arterial diseases.
- Role of multidetector computed tomography in paranasal sinus pathologies
- Role of virtual endoscopy using MDCT in detection & evaluation of gastric pathologies
- High resolution 3 Tesla MRI in the evaluation of ankle and hindfoot pain.
- Transperineal ultrasonography in infants with anorectal malformation
- CT portography using MDCT versus color Doppler in detection of varices in cirrhotic patients
- Role of CT urography in the evaluation of a dilated ureter
- Characterization of pulmonary nodules by dynamic contrast-enhanced multidetector CT
- Comprehensive imaging of acute ischemic stroke on multidetector CT
- The role of fetal MRI in the diagnosis of intrauterine neurological congenital anomalies
- Role of Multidetector computed tomography in pediatric chest masses
- Multimodality imaging in the evaluation of palpable & non-palpable breast lesion.
- Sonographic Assessment Of Fetal Nasal Bone Length At 11-28 Gestational Weeks And Its Correlation With Fetal Outcome.
- Role Of Sonoelastography And Contrast-Enhanced Computed Tomography In Evaluation Of Lymph Node Metastasis In Head And Neck Cancers
- Role Of Renal Doppler And Shear Wave Elastography In Diabetic Nephropathy
- Evaluation Of Relationship Between Various Grades Of Fatty Liver And Shear Wave Elastography Values
- Evaluation and characterization of pelvic masses of gynecological origin by USG, color Doppler and MRI in females of reproductive age group
- Radiological evaluation of small bowel diseases using computed tomographic enterography
- Role of coronary CT angiography in patients of coronary artery disease
- Role of multimodality imaging in the evaluation of pediatric neck masses
- Role of CT in the evaluation of craniocerebral trauma
- Role of magnetic resonance imaging (MRI) in the evaluation of spinal dysraphism
- Comparative evaluation of triple phase CT and dynamic contrast-enhanced MRI in patients with liver cirrhosis
- Evaluation of the relationship between carotid intima-media thickness and coronary artery disease in patients evaluated by coronary angiography for suspected CAD
- Assessment of hepatic fat content in fatty liver disease by unenhanced computed tomography
- Correlation of vertebral marrow fat on spectroscopy and diffusion-weighted MRI imaging with bone mineral density in postmenopausal women.
- Comparative evaluation of CT coronary angiography with conventional catheter coronary angiography
- Ultrasound evaluation of kidney length & descending colon diameter in normal and intrauterine growth-restricted fetuses
- A prospective study of hepatic vein waveform and splenoportal index in liver cirrhosis: correlation with child Pugh’s classification and presence of esophageal varices.
- CT angiography to evaluate coronary artery by-pass graft patency in symptomatic patient’s functional assessment of myocardium by cardiac MRI in patients with myocardial infarction
- MRI evaluation of HIV positive patients with central nervous system manifestations
- MDCT evaluation of mediastinal and hilar masses
- Evaluation of rotator cuff & labro-ligamentous complex lesions by MRI & MRI arthrography of shoulder joint
- Role of imaging in the evaluation of soft tissue vascular malformation
- Role of MRI and ultrasonography in the evaluation of multifidus muscle pathology in chronic low back pain patients
- Role of ultrasound elastography in the differential diagnosis of breast lesions
- Role of magnetic resonance cholangiopancreatography in evaluating dilated common bile duct in patients with symptomatic gallstone disease.
- Comparative study of CT urography & hybrid CT urography in patients with haematuria.
- Role of MRI in the evaluation of anorectal malformations
- Comparison of ultrasound-Doppler and magnetic resonance imaging findings in rheumatoid arthritis of hand and wrist
- Role of Doppler sonography in the evaluation of renal artery stenosis in hypertensive patients undergoing coronary angiography for coronary artery disease.
- Comparison of radiography, computed tomography and magnetic resonance imaging in the detection of sacroiliitis in ankylosing spondylitis.
- Mr evaluation of painful hip
- Role of MRI imaging in pretherapeutic assessment of oral and oropharyngeal malignancy
- Evaluation of diffuse lung diseases by high resolution computed tomography of the chest
- Mr evaluation of brain parenchyma in patients with craniosynostosis.
- Diagnostic and prognostic value of cardiovascular magnetic resonance imaging in dilated cardiomyopathy
- Role of multiparametric magnetic resonance imaging in the detection of early carcinoma prostate
- Role of magnetic resonance imaging in white matter diseases
- Role of sonoelastography in assessing the response to neoadjuvant chemotherapy in patients with locally advanced breast cancer.
- Role of ultrasonography in the evaluation of carotid and femoral intima-media thickness in predialysis patients with chronic kidney disease
- Role of H1 MRI spectroscopy in focal bone lesions of peripheral skeleton choline detection by MRI spectroscopy in breast cancer and its correlation with biomarkers and histological grade.
- Ultrasound and MRI evaluation of axillary lymph node status in breast cancer.
- Role of sonography and magnetic resonance imaging in evaluating chronic lateral epicondylitis.
- Comparative of sonography including Doppler and sonoelastography in cervical lymphadenopathy.
- Evaluation of Umbilical Coiling Index as Predictor of Pregnancy Outcome.
- Computerized Tomographic Evaluation of Azygoesophageal Recess in Adults.
- Lumbar Facet Arthropathy in Low Backache.
- “Urethral Injuries After Pelvic Trauma: Evaluation with Uretrography
- Role Of Ct In Diagnosis Of Inflammatory Renal Diseases
- Role Of Ct Virtual Laryngoscopy In Evaluation Of Laryngeal Masses
- “Ct Portography Using Mdct Versus Color Doppler In Detection Of Varices In
- Cirrhotic Patients”
- Role Of Multidetector Ct In Characterization Of Renal Masses
- Role Of Ct Virtual Cystoscopy In Diagnosis Of Urinary Bladder Neoplasia
- Role Of Multislice Ct In Diagnosis Of Small Intestine Tumors
- “Mri Flow Quantification In The Assessment Of The Commonest CSF Flow Abnormalities”
- “The Role Of Fetal Mri In Diagnosis Of Intrauterine Neurological CongenitalAnomalies”
- Role Of Transcranial Ultrasound In Diagnosis Of Neonatal Brain Insults
- “The Role Of Interventional Imaging Procedures In The Treatment Of Selected Gynecological Disorders”
- Role Of Radiological Imaging In Diagnosis Of Endometrial Carcinoma
- “Role Of High-Resolution Ct In Differentiation Between Benign And Malignant Pulmonary Nodules In Children”
- Role Of Ultrasonography In The Diagnosis Of Knee Joint Lesions
- “Role Of Diagnostic Imaging Modalities In Evaluation Of Post Liver Transplantation Recipient Complications”
- “Diffusion-Weighted Magnetic Resonance Imaging In Diagnosis And
- Characterization Of Brain Tumors In Correlation With Conventional Mri”
- The Role Of PET-CT In The Evaluation Of Hepatic Tumors
- “Role Of Computerized Tomography In Evaluation Of Mediastinal Masses In Pediatric patients”
- “Trans Vaginal Ultrasound And Magnetic Resonance Imaging In Female Urinary Incontinence”
- Role Of Multidetector Ct In Diagnosis Of Urinary Bladder Cancer
- “Role Of Transvaginal Ultrasound In Diagnosis And Treatment Of Female Infertility”
- Role Of Diffusion-Weighted Mri Imaging In Evaluation Of Cancer Prostate
- “Role Of Positron Emission Tomography With Computed Tomography In Diagnosis Of Cancer Thyroid”
- The Role Of CT Urography In Case Of Haematuria
- “Value Of Ultrasonography In Assessment Of Acute Abdominal Diseases In Pediatric Age Group”
- “Role Of Functional Magnetic Resonance Imaging In Making Brain Tumor Surgery Safer”
- The Role Of Sonoelastography In Characterization Of Breast Lesions
- “Ultrasonography, Magnetic Resonance Cholangiopancreatography (MRCP) In Assessment Of Pediatric Biliary Lesions”
- “Role Of Ultrasound And Color Doppler Imaging In Assessment Of Acute Abdomen Due To Female Genital Causes”
- “Role Of Multidetector Ct Virtual Laryngoscopy In Evaluation Of Laryngeal Mass Lesions”
- MRI Of The Postoperative Knee
- Role Of Mri In Assessment Of Valvular Heart Diseases
- The Role Of 3D & 4D Ultrasonography In Abnormalities Of Fetal Abdomen
- State Of The Art Of Mri In Diagnosis Of Hepatic Focal Lesions
- Role Of Multidetector Ct In Diagnosis Of Salivary Gland Lesions
- “Role Of Virtual Endoscopy Using Mdct In Detection & Evaluation Of Gastric Pathologies”
- The Role Of Ultrasound & Mri In Acute Pelvic Inflammatory Disease
- “Diagnosis & Staging Of Liver Fibrosis By Ultraso Und Elastography In
- Patients With Chronic Liver Diseases”
- Role Of Mri In Evaluation Of Spinal Trauma
- Validity Of Mri In Diagnosis Of Congenital Anorectal Anomalies
- Imaging Of Vascular Complication After Liver Transplantation
- “Contrast-Enhanced Digital Mammography And Digital Breast Tomosynthesis In Early Diagnosis Of Breast Lesion”
- Role Of Mammotome In Breast Lesions
- “Role Of MRI Diffusion Tensor Imaging (DTI) In Assessment Of Traumatic Spinal Cord Injuries”
- “Prediction Of Pre-eclampsia And Fetal Growth Restriction By Uterine Artery Doppler”
- “Role Of Multidetector Row Computed Tomography In Assessment Of Maxillofacial Trauma”
- “Role Of Diffusion Magnetic Resonance Imaging In Assessment Of Neoplastic And Inflammatory Brain Lesions”
- Role Of Diffusion Mri In Preoperative Evaluation Of Brain Neoplasms
- “Role Of Multidetector Ct Virtual Hysteroscopy In The Detection Of The
- Uterine & Tubal Causes Of Female Infertility”
- Role Of Advances Magnetic Resonance Imaging Sequences In Multiple Sclerosis Magnetic Resonance Spectroscopy In Multiple Sclerosis
- “Role Of Conventional Mri, And Diffusion Tensor Imaging Tractography In Evaluation Of Congenital Brain Malformations”
- Role Of MRI In Evaluation Of Spinal Trauma
- Diagnostic Role Of Diffusion-weighted MR Imaging In Neck Masses
- “The Role Of Transvaginal Ultrasound Versus Magnetic Resonance Imaging In Diagnosis & Evaluation Of Cancer Cervix”
- “Role Of 3d Magnetic Resonance Imaging Tractography In Assessment Of White Matter Tracts Compromise In Supra Tentorial Tumors”
- Role Of Proton MR Spectroscopy In The Evaluation Of Temporal Lobe Epilepsy
- Role Of Multislice Computed Tomography In Evaluation Of Crohn’s Disease
- Role Of MRI In Assessment Of Hydrocephalus In Pediatric Patients
- The Role Of MRI In Diagnosis And Staging Of Urinary Bladder Carcinoma
- USG and MRI correlation of congenital CNS anomalies
- HRCT in interstitial lung disease
- X-Ray, CT and MRI correlation of bone tumors
- “Study on the diagnostic and prognostic utility of X-Rays for cases of pulmonary tuberculosis under RNTCP”
- “Role of magnetic resonance imaging in the characterization of female adnexal pathology”
- “CT angiography of carotid atherosclerosis and NECT brain in cerebral ischemia, a correlative analysis”
- Role of CT scan in the evaluation of paranasal sinus pathology
- USG and MRI correlation on shoulder joint pathology
- “Radiological evaluation of a patient presenting with extrapulmonary tuberculosis”
- CT and MRI correlation in focal liver lesions”
- Comparison of MDCT virtual cystoscopy with conventional cystoscopy in bladder tumors”
- “Bleeding vessels in life-threatening hemoptysis: Comparison of 64 detector row CT angiography with conventional angiography prior to endovascular management”
- “Role of transarterial chemoembolization in unresectable hepatocellular carcinoma”
- “Comparison of color flow duplex study with digital subtraction angiography in the evaluation of peripheral vascular disease”
- “A Study to assess the efficacy of magnetization transfer ratio in differentiating tuberculoma from neurocysticercosis”
- “MR evaluation of uterine mass lesions in correlation with transabdominal, transvaginal ultrasound using HPE as a gold standard”
- “The Role of power Doppler imaging with trans rectal ultrasonogram guided prostate biopsy in the detection of prostate cancer”
- “Lower limb arteries assessed with doppler angiography – A prospective comparative study with multidetector CT angiography”
- “Comparison of sildenafil with papaverine in penile doppler by assessing hemodynamic changes”
- “Evaluation of efficacy of sonosalphingogram for assessing tubal patency in infertile patients with hysterosalpingogram as the gold standard”
- Role of CT enteroclysis in the evaluation of small bowel diseases
- “MRI colonography versus conventional colonoscopy in the detection of colonic polyposis”
- “Magnetic Resonance Imaging of anteroposterior diameter of the midbrain – differentiation of progressive supranuclear palsy from Parkinson disease”
- “MRI Evaluation of anterior cruciate ligament tears with arthroscopic correlation”
- “The Clinicoradiological profile of cerebral venous sinus thrombosis with prognostic evaluation using MR sequences”
- “Role of MRI in the evaluation of pelvic floor integrity in stress incontinent patients” “Doppler ultrasound evaluation of hepatic venous waveform in portal hypertension before and after propranolol”
- “Role of transrectal sonography with colour doppler and MRI in evaluation of prostatic lesions with TRUS guided biopsy correlation”
- “Ultrasonographic evaluation of painful shoulders and correlation of rotator cuff pathologies and clinical examination”
- “Colour Doppler Evaluation of Common Adult Hepatic tumors More Than 2 Cm with HPE and CECT Correlation”
- “Clinical Relevance of MR Urethrography in Obliterative Posterior Urethral Stricture”
- “Prediction of Adverse Perinatal Outcome in Growth Restricted Fetuses with Antenatal Doppler Study”
- Radiological evaluation of spinal dysraphism using CT and MRI
- “Evaluation of temporal bone in cholesteatoma patients by high resolution computed tomography”
- “Radiological evaluation of primary brain tumours using computed tomography and magnetic resonance imaging”
- “Three dimensional colour doppler sonographic assessment of changes in volume and vascularity of fibroids – before and after uterine artery embolization”
- “In phase opposed phase imaging of bone marrow differentiating neoplastic lesions”
- “Role of dynamic MRI in replacing the isotope renogram in the functional evaluation of PUJ obstruction”
- Characterization of adrenal masses with contrast-enhanced CT – washout study
- A study on accuracy of magnetic resonance cholangiopancreatography
- “Evaluation of median nerve in carpal tunnel syndrome by high-frequency ultrasound & color doppler in comparison with nerve conduction studies”
- “Correlation of Agatston score in patients with obstructive and nonobstructive coronary artery disease following STEMI”
- “Doppler ultrasound assessment of tumor vascularity in locally advanced breast cancer at diagnosis and following primary systemic chemotherapy.”
- “Validation of two-dimensional perineal ultrasound and dynamic magnetic resonance imaging in pelvic floor dysfunction.”
- “Role of MR urethrography compared to conventional urethrography in the surgical management of obliterative urethral stricture.”
Search Diagnostic Imaging Research Topics
You can also search research-related resources and direct download PDFs for radiology articles on our custom radiology search engine .
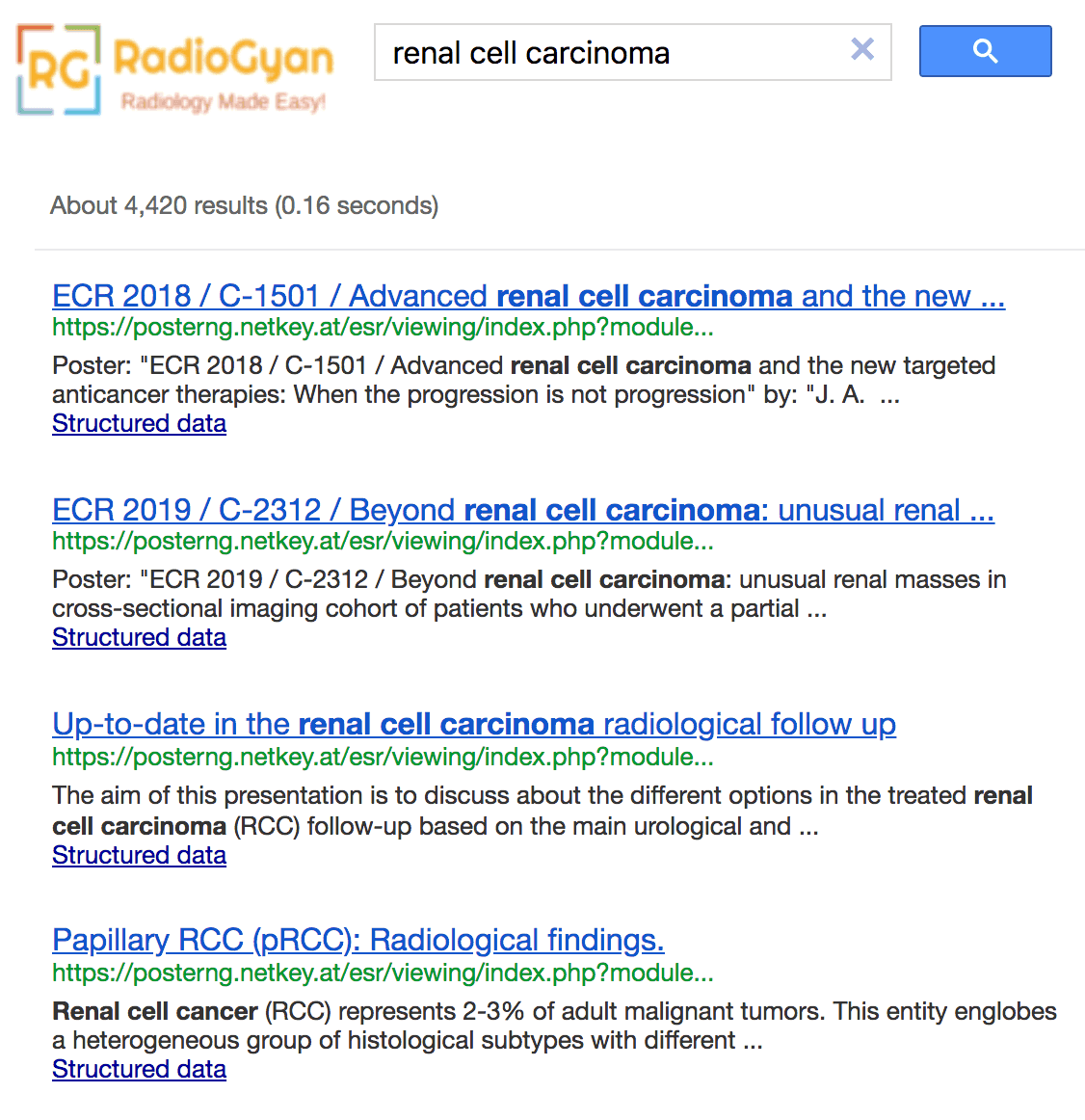
Free Resources for Preparing Radiology Thesis
- Radiology thesis topics- Benha University – Free to download thesis
- Radiology thesis topics – Faculty of Medical Science Delhi
- Radiology thesis topics – IPGMER
- Fetal Radiology thesis Protocols
- Radiology thesis and dissertation topics
- Radiographics
Proofreading Your Thesis:
Make sure you use Grammarly to correct your spelling , grammar , and plagiarism for your thesis. Grammarly has affordable paid subscriptions, windows/macOS apps, and FREE browser extensions. It is an excellent tool to avoid inadvertent spelling mistakes in your research projects. It has an extensive built-in vocabulary, but you should make an account and add your own medical glossary to it.

Guidelines for Writing a Radiology Thesis:
These are general guidelines and not about radiology specifically. You can share these with colleagues from other departments as well. Special thanks to Dr. Sanjay Yadav sir for these. This section is best seen on a desktop. Here are a couple of handy presentations to start writing a thesis:
Read the general guidelines for writing a thesis (the page will take some time to load- more than 70 pages!
A format for thesis protocol with a sample patient information sheet, sample patient consent form, sample application letter for thesis, and sample certificate.
Resources and References:
- Guidelines for thesis writing.
- Format for thesis protocol
- Thesis protocol writing guidelines DNB
- Informed consent form for Research studies from AIIMS
- Radiology Informed consent forms in local Indian languages.
- Sample Informed Consent form for Research in Hindi
- Guide to write a thesis by Dr. P R Sharma
- Guidelines for thesis writing by Dr. Pulin Gupta.
- Preparing MD/DNB thesis by A Indrayan
- Another good thesis reference protocol
Hopefully, this post will make the tedious task of writing a Radiology thesis a little bit easier for you. Best of luck with writing your thesis and your residency too!
More guides for residents :
- Guide for the MD/DMRD/DNB radiology exam!
- Guide for First-Year Radiology Residents
FRCR Exam: THE Most Comprehensive Guide (2022)!
- Radiology Practical Exams Questions compilation for MD/DNB/DMRD !
- Radiology Exam Resources (Oral Recalls, Instruments, etc )!
Tips and Tricks for DNB/MD Radiology Practical Exam
- FRCR 2B exam- Tips and Tricks !
- FRCR exam preparation – An alternative take!
- Why did I take up Radiology?
- Radiology Conferences – A comprehensive guide!
- ECR (European Congress Of Radiology)
- European Diploma in Radiology (EDiR) – The Complete Guide!
- Radiology NEET PG guide – How to select THE best college for post-graduation in Radiology (includes personal insights)!
- Interventional Radiology – All Your Questions Answered!
- What It Means To Be A Radiologist: A Guide For Medical Students!
- Radiology Mentors for Medical Students (Post NEET-PG)
- MD vs DNB Radiology: Which Path is Right for Your Career?
- DNB Radiology OSCE – Tips and Tricks
More radiology resources here: Radiology resources This page will be updated regularly. Kindly leave your feedback in the comments or send us a message here . Also, you can comment below regarding your department’s thesis topics.
Note: All topics have been compiled from available online resources. If anyone has an issue with any radiology thesis topics displayed here, you can message us here , and we can delete them. These are only sample guidelines. Thesis guidelines differ from institution to institution.
Image source: Thesis complete! (2018). Flickr. Retrieved 12 August 2018, from https://www.flickr.com/photos/cowlet/354911838 by Victoria Catterson
About The Author
Dr. amar udare, md, related posts ↓.

7 thoughts on “Radiology Thesis – More than 400 Research Topics (2022)!”
Amazing & The most helpful site for Radiology residents…
Thank you for your kind comments 🙂
Dr. I saw your Tips is very amazing and referable. But Dr. Can you help me with the thesis of Evaluation of Diagnostic accuracy of X-ray radiograph in knee joint lesion.
Wow! These are excellent stuff. You are indeed a teacher. God bless
Glad you liked these!
happy to see this
Glad I could help :).
Leave a Comment Cancel Reply
Your email address will not be published. Required fields are marked *
Get Radiology Updates to Your Inbox!
This site is for use by medical professionals. To continue, you must accept our use of cookies and the site's Terms of Use. Learn more Accept!
Wish to be a BETTER Radiologist? Join 14000 Radiology Colleagues !
Enter your email address below to access HIGH YIELD radiology content, updates, and resources.
No spam, only VALUE! Unsubscribe anytime with a single click.
- Online Courses
- New England
- Grants, Abstracts, And Presentations
INFORMATION FOR
- Residents & Fellows
- Researchers
Publications
- Spatiotemporal Deep Learning-Based Cine Loop Quality Filter for Handheld Point-of-Care Echocardiography. Mukaddim RA, Mackay E, Gessert N, Erkamp R, Sethuraman S, Sutton J, Bharat S, Jutras M, Baloescu C, Moore CL, Raju B. IEEE Trans Ultrason Ferroelectr Freq Control. 2024 May 3; 2024 May 3. PMID: 38700961.
- Emergency Physician-performed Echocardiogram in Non-ST Elevation Acute Coronary Syndrome Patients Requiring Coronary Intervention. Xu Tan T, Wright D, Baloescu C, Lee S, Moore CL. West J Emerg Med. 2024 Jan. PMID: 38205979.
- Reply. Moore CL, Baskin A, Cheung D, Davis MA, Fertel BS, Larson DM, Lee RK, McCabe-Kline KB, Mills AM, Nicola GN, Nicola LP. J Am Coll Radiol. 2023 Dec; 2023 Jun 20. PMID: 37348746.
- Emergency Department Point-of-Care Echocardiography and Lung Ultrasound in Predicting COVID-19 Severity. Baloescu C, Weingart GE, Moore CL. J Ultrasound Med. 2023 Aug; 2023 Feb 25. PMID: 36840721.
- White Paper: Best Practices in the Communication and Management of Actionable Incidental Findings in Emergency Department Imaging. Moore CL, Baskin A, Chang AM, Cheung D, Davis MA, Fertel BS, Hans K, Kang SK, Larson DM, Lee RK, McCabe-Kline KB, Mills AM, Nicola GN, Nicola LP. J Am Coll Radiol. 2023 Apr; 2023 Mar 13. PMID: 36922265.
- Interobserver Agreement and Correlation of an Automated Algorithm for B-Line Identification and Quantification With Expert Sonologist Review in a Handheld Ultrasound Device. Moore CL, Wang J, Battisti AJ, Chen A, Fincke J, Wang A, Wagner M, Raju B, Baloescu C. J Ultrasound Med. 2022 Oct; 2021 Dec 28. PMID: 34964489.
- Novel Quality Measure Set: Closing the Completion Loop on Radiology Follow-up Recommendations for Noncritical Actionable Incidental Findings. Kadom N, Venkatesh AK, Shugarman SA, Burleson JH, Moore CL, Seidenwurm D. J Am Coll Radiol. 2022 Jul; 2022 May 21. PMID: 35606263.
- Closing the Compliance Loop on Follow-Up Imaging Recommendations: Comparing Radiologists' and Administrators' Attitudes. Kadom N, Fredericks N, Moore CL, Seidenwurm D, Shugarman S, Venkatesh A. Curr Probl Diagn Radiol. 2022 Jul-Aug; 2021 Aug 29. PMID: 34565635.
- Radiation Dose Reduction in Kidney Stone CT: A Randomized, Facility-Based Intervention. Moore CL, Bhargavan-Chatfield M, Shaw MM, Weisenthal K, Kalra MK. J Am Coll Radiol. 2021 Oct; 2021 Jun 9. PMID: 34115990.
- Incidental Findings: A Survey of Radiologists and Emergency Physicians. Moore CL, Kadom N, Seidenwurm D, Nicola G, Fredericks N, Shugarman S, Venkatesh A. J Am Coll Radiol. 2021 Jun; 2021 Jan 29. PMID: 33516766.
- Young Man With Shortness of Breath. Hodson DZ, Reinhardt SW, Moore CL. Ann Emerg Med. 2021 May. PMID: 33902827.
- Paramedic-performed Prehospital Point-of-care Ultrasound for Patients with Undifferentiated Dyspnea: A Pilot Study. Schoeneck JH, Coughlin RF, Baloescu C, Cone DC, Liu RB, Kalam S, Medoro AK, Medoro I, Joseph D, Burns K, Bohrer-Clancy JI, Moore CL. West J Emerg Med. 2021 Mar 24; 2021 Mar 24. PMID: 34125056.
- Automated Lung Ultrasound B-Line Assessment Using a Deep Learning Algorithm. Baloescu C, Toporek G, Kim S, McNamara K, Liu R, Shaw MM, McNamara RL, Raju BI, Moore CL. IEEE Trans Ultrason Ferroelectr Freq Control. 2020 Nov; 2020 Jun 15. PMID: 32746183.
- Ultrasound on the Frontlines of COVID-19: Report From an International Webinar. Liu RB, Tayal VS, Panebianco NL, Tung-Chen Y, Nagdev A, Shah S, Pivetta E, Henwood PC, Nelson MJ, Moore CL. Acad Emerg Med. 2020 Jun; 2020 Jun 9. PMID: 32348585.
- Imaging in Suspected Renal Colic: Systematic Review of the Literature and Multispecialty Consensus. Moore CL, Carpenter CR, Heilbrun ML, Klauer K, Krambeck AC, Moreno C, Remer EM, Scales C, Shaw MM, Sternberg KM. J Urol. 2019 Sep; 2019 Aug 8. PMID: 31412438.
- Imaging in Suspected Renal Colic: Systematic Review of the Literature and Multispecialty Consensus. Moore CL, Carpenter CR, Heilbrun ME, Klauer K, Krambeck AC, Moreno C, Remer EM, Scales C, Shaw MM, Sternberg KM. J Am Coll Radiol. 2019 Sep; 2019 Aug 8. PMID: 31402228.
- Imaging in Suspected Renal Colic: Systematic Review of the Literature and Multispecialty Consensus. Moore CL, Carpenter CR, Heilbrun ME, Klauer K, Krambeck A, Moreno C, Remer EM, Scales C, Shaw MM, Sternberg KM. Ann Emerg Med. 2019 Sep; 2019 Aug 8. PMID: 31402153.
- Point-of-Care Ultrasound: Does it Affect Scores on Standardized Assessment Tests Used Within the Preclinical Curriculum? Liu RB, Suwondo DN, Donroe JH, Encandela JA, Weisenthal KS, Moore CL. J Ultrasound Med. 2019 Feb; 2018 Jul 29. PMID: 30058124.
- Assessment of Myocardial Function and Injury by Echocardiography and Cardiac Biomarkers in African Children With Severe Plasmodium falciparum Malaria. Kotlyar S, Olupot-Olupot P, Nteziyaremye J, Akech SO, Uyoga S, Muhindo R, Moore CL, Maitland K. Pediatr Crit Care Med. 2018 Mar. PMID: 29206727.
- Predictors of Hospital Admission and Urological Intervention in Adult Emergency Department Patients with Computerized Tomography Confirmed Ureteral Stones. Daniels B, Schoenfeld E, Taylor A, Weisenthal K, Singh D, Moore CL. J Urol. 2017 Dec; 2017 Jun 23. PMID: 28652122.
- Right Upper Quadrant Pain: Ultrasound First! Revzin MV, Scoutt LM, Garner JG, Moore CL. J Ultrasound Med. 2017 Oct; 2017 Jun 6. PMID: 28586152.
- Emergency physician performed tricuspid annular plane systolic excursion in the evaluation of suspected pulmonary embolism. Daley J, Grotberg J, Pare J, Medoro A, Liu R, Hall MK, Taylor A, Moore CL. Am J Emerg Med. 2017 Jan; 2016 Oct 11. PMID: 27793505.
- Use of Point-of-Care Ultrasound in the Emergency Department: Insights From the 2012 Medicare National Payment Data Set. Hall MK, Hall J, Gross CP, Harish NJ, Liu R, Maroongroge S, Moore CL, Raio CC, Taylor RA. J Ultrasound Med. 2016 Nov; 2016 Oct 3. PMID: 27698180.
- Corrected flow time: a noninvasive ultrasound measure to detect preload reduction by nitroglycerin. Pare JR, Liu R, Moore CL, Safdar B. Am J Emerg Med. 2016 Sep; 2016 Jun 24. PMID: 27431739.
- STONE PLUS: Evaluation of Emergency Department Patients With Suspected Renal Colic, Using a Clinical Prediction Tool Combined With Point-of-Care Limited Ultrasonography. Daniels B, Gross CP, Molinaro A, Singh D, Luty S, Jessey R, Moore CL. Ann Emerg Med. 2016 Apr; 2015 Dec 31. PMID: 26747219.
- Emergency physician focused cardiac ultrasound improves diagnosis of ascending aortic dissection. Pare JR, Liu R, Moore CL, Sherban T, Kelleher MS Jr, Thomas S, Taylor RA. Am J Emerg Med. 2016 Mar; 2015 Dec 12. PMID: 26782795.
- External Validation of the STONE Score. Moore CL, Daniels B, Gross CP, Luty S, Singh D. Ann Emerg Med. 2016 Feb. PMID: 26801385.
- Defining Fluid Responsiveness by the Velocity-Time Integral Alone? Pare JR, Moore CL. J Ultrasound Med. 2016 Feb. PMID: 26795050.
- Does Ultrasound Improve Clinical Outcomes? Prove It. Moore CL. Crit Care Med. 2015 Dec. PMID: 26575658.
- McConnell's Sign Is Not Specific for Pulmonary Embolism: Case Report and Review of the Literature. Walsh BM, Moore CL. J Emerg Med. 2015 Sep; 2015 May 16. PMID: 25986329.
- The student's dilemma, liver edition: incorporating the sonographer's language into clinical anatomy education. Hall MK, Mirjalili SA, Moore CL, Rizzolo LJ. Anat Sci Educ. 2015 May-Jun; 2015 Jan 8. PMID: 25573229.
- Independent evaluation of a simple clinical prediction rule to identify right ventricular dysfunction in patients with shortness of breath. Russell FM, Moore CL, Courtney DM, Kabrhel C, Smithline HA, Nordenholz KE, Richman PB, O'Neil BJ, Plewa MC, Beam DM, Mastouri R, Kline JA. Am J Emerg Med. 2015 Apr; 2015 Jan 22. PMID: 25769797.
- Use of point-of-care ultrasound in Connecticut emergency departments. Herbst MK, Camargo CA Jr, Perez A, Moore CL. J Emerg Med. 2015 Feb; 2014 Nov 6. PMID: 25440859.
- Effect of provider experience on clinician-performed ultrasonography for hydronephrosis in patients with suspected renal colic. Herbst MK, Rosenberg G, Daniels B, Gross CP, Singh D, Molinaro AM, Luty S, Moore CL. Ann Emerg Med. 2014 Sep; 2014 Mar 11. PMID: 24630203.
- Ultrasound first, second, and last for vascular access. Moore CL. J Ultrasound Med. 2014 Jul. PMID: 24958398.
- Spleen volume and clinical disease manifestations of severe Plasmodium falciparum malaria in African children. Kotlyar S, Nteziyaremye J, Olupot-Olupot P, Akech SO, Moore CL, Maitland K. Trans R Soc Trop Med Hyg. 2014 May; 2014 Mar 16. PMID: 24639372.
- Accuracy of emergency physician-performed limited echocardiography for right ventricular strain. Taylor RA, Moore CL. Am J Emerg Med. 2014 Apr; 2013 Dec 27. PMID: 24559906.
- Bedside optic nerve sheath diameter ultrasound for the evaluation of suspected pediatric ventriculoperitoneal shunt failure in the emergency department. Hall MK, Spiro DM, Sabbaj A, Moore CL, Hopkins KL, Meckler GD. Childs Nerv Syst. 2013 Dec; 2013 Jun 1. PMID: 23728433.
- The vanishing target sign: confirmation of intraluminal needle position for ultrasound guided vascular access. Thomas S, Moore CL. Acad Emerg Med. 2013 Oct. PMID: 24127720.
- Point-of-care focused cardiac ultrasound for prediction of pulmonary embolism adverse outcomes. Taylor RA, Davis J, Liu R, Gupta V, Dziura J, Moore CL. J Emerg Med. 2013 Sep; 2013 Jul 2. PMID: 23827166.
- Sonography first for acute flank pain? Moore CL, Scoutt L. J Ultrasound Med. 2012 Nov. PMID: 23091240.
- Use of ultrasound guidance improves central venous catheter insertion success rates among junior residents. Dodge KL, Lynch CA, Moore CL, Biroscak BJ, Evans LV. J Ultrasound Med. 2012 Oct. PMID: 23011614.
- Point-of-care focused cardiac ultrasound for the assessment of thoracic aortic dimensions, dilation, and aneurysmal disease. Taylor RA, Oliva I, Van Tonder R, Elefteriades J, Dziura J, Moore CL. Acad Emerg Med. 2012 Feb; 2012 Jan 30. PMID: 22288871.
- Development and validation of a web-based assessment tool for the extended focused assessment with sonography in trauma examination. Markowitz JE, Hwang JQ, Moore CL. J Ultrasound Med. 2011 Mar. PMID: 21357559.
- Point-of-care ultrasonography. Moore CL, Copel JA. N Engl J Med. 2011 Feb 24. PMID: 21345104.
- Identification of Sonographic B-lines with Linear Transducer Predicts Elevated B-Type Natriuretic Peptide Level. Manson WC, Bonz JW, Carmody K, Osborne M, Moore CL. West J Emerg Med. 2011 Feb. PMID: 21691481.
- Identification of subcutaneous myiasis using bedside emergency physician performed ultrasound. Schechter E, Lazar J, Nix ME, Mallon WK, Moore CL. J Emerg Med. 2011 Jan; 2008 Oct 23. PMID: 18947960.
- Tissue Doppler of early mitral filling correlates with simulated volume loss in healthy subjects. Moore CL, Tham ET, Samuels KJ, McNamara RL, Galante NJ, Stachenfeld N, Shelley K, Dziura J, Silverman DG. Acad Emerg Med. 2010 Nov. PMID: 21175513.
- Point-of-care sonographic diagnosis of abdominal wall venous thrombosis associated with inferior vena cava ligation and masquerading as cellulitis. Sutijono D, van Tonder R, Moore CL. J Ultrasound Med. 2010 Nov. PMID: 20966476.
- Simulation training in central venous catheter insertion: improved performance in clinical practice. Evans LV, Dodge KL, Shah TD, Kaplan LJ, Siegel MD, Moore CL, Hamann CJ, Lin Z, D'Onofrio G. Acad Med. 2010 Sep. PMID: 20736674.
- Emergency ultrasound diagnosis of a pseudoaneurysm associated with an arteriovenous fistula. Arshad FH, Sutijono D, Moore CL. Acad Emerg Med. 2010 Jun; 2010 May 14. PMID: 20491681.
- Assessing the utility of ultrasound in Liberia. Kotlyar S, Moore CL. J Emerg Trauma Shock. 2008 Jan. PMID: 19561936.
- Diagnosis and guided reduction of forearm fractures in children using bedside ultrasound. Chen L, Kim Y, Moore CL. Pediatr Emerg Care. 2007 Aug. PMID: 17726410.
- Ultrasonography in community emergency departments in the United States: access to ultrasonography performed by consultants and status of emergency physician-performed ultrasonography. Moore CL, Molina AA, Lin H. Ann Emerg Med. 2006 Feb; 2005 Nov 21. PMID: 16431225.
- Utility of bedside bladder ultrasound before urethral catheterization in young children. Chen L, Hsiao AL, Moore CL, Dziura JD, Santucci KA. Pediatrics. 2005 Jan. PMID: 15629989.
- Surgeon-performed ultrasound for pneumothorax in the trauma suite. Moore CL. J Trauma. 2004 Sep. PMID: 15454826.
- Performance, training, quality assurance, and reimbursement of emergency physician-performed ultrasonography at academic medical centers. Moore CL, Gregg S, Lambert M. J Ultrasound Med. 2004 Apr. PMID: 15098862.
- Determination of left ventricular function by emergency physician echocardiography of hypotensive patients. Moore CL, Rose GA, Tayal VS, Sullivan DM, Arrowood JA, Kline JA. Acad Emerg Med. 2002 Mar. PMID: 11874773.
Books and Chapters
The Emergency and Critical Care Ultrasound Pocket Manual, In Press, Thieme Publications.
- Moore CL, Hwang J. Emergency Bedside Echocardiography, in: Clinical Emergency Radiology. Chris Fox (ed.), Cambridge University Press 2007, in press.
- Moore CL. Emergency Pelvic Ultrasound, in: Ultrasound-Guided Procedures and Investigations, David Feller-Kopman (ed.), Marcell Dekker 2006.
- Moore CL, Lin H. Echocardiography, in: Practical Guide to Emergency Ultrasound, John Kendall (ed.), Lipincott Williams and Wilkins; 2005.
- Tayal VS, Moore CL. Emergency Echocardiography, in: Emergency Ultrasound, Ma OJ (ed.), McGraw Hill; 2002.
Department of Circulation and Medical Imaging
- Master's programmes in English
- For exchange students
- PhD opportunities
- All programmes of study
- Language requirements
- Application process
- Academic calendar
- NTNU research
- Research excellence
- Strategic research areas
- Innovation resources
- Student in Trondheim
- Student in Gjøvik
- Student in Ålesund
- For researchers
- Life and housing
- Faculties and departments
- International researcher support
Språkvelger
Master thesis and projects - ultrasound technology - studies - department of circulation and medical imaging.
- Master thesis and projects
- Specialisation courses
Master's thesis and projects
Master's thesis and projects.
The Department of circulation and medical imaging offers projects and master's thesis topics for technology students of most of the different technical study programmes at NTNU. There is a seperate page for the supplementary specialisation courses .
List of topics
Topics for thesis and projects are given below. Most of the topics can be adjusted to the students qualifications and wishes.
Don't hesitate to take contact with the corresponding supervisor - we're looking forward to a discussion with you!
Asset Publisher
Blood flow imaging projects, estimation of true flow velocity using ultrasound, fusion of multi-modal cardiac data, pocket size ultrasound technology, pulse-echo based method for estimation of speed of sound, ultrasonic imaging through solids, surf imaging topics, ultrasound mediated drug delivery, real-time monitoring of left ventricular function under interventional procedures, fighting cancer with cw shear-wave elastography, adaptive clutter filtering for coronary heart disease, patient adaptive imaging in echocardiography, how to write ....
- a good abstract
- a good introduction
person-portlet
Lasse løvstakken professor.
- U.S. Department of Health & Human Services
- National Institutes of Health

En Español | Site Map | Staff Directory | Contact Us
- Science Education
- Science Topics
What is medical ultrasound?
How does it work, what is ultrasound used for, are there risks, what are examples of nibib-funded projects using ultrasound.
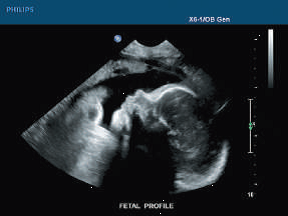
Medical ultrasound falls into two distinct categories: diagnostic and therapeutic.
Diagnostic ultrasound can be further sub-divided into anatomical and functional ultrasound. Anatomical ultrasound produces images of internal organs or other structures. Functional ultrasound combines information such as the movement and velocity of tissue or blood, softness or hardness of tissue, and other physical characteristics, with anatomical images to create “information maps.” These maps help doctors visualize changes/differences in function within a structure or organ.
Therapeutic ultrasound also uses sound waves above the range of human hearing but does not produce images. Its purpose is to interact with tissues in the body such that they are either modified or destroyed. Among the modifications possible are: moving or pushing tissue, heating tissue, dissolving blood clots, or delivering drugs to specific locations in the body. These destructive, or ablative, functions are made possible by use of very high-intensity beams that can destroy diseased or abnormal tissues such as tumors. The advantage of using ultrasound therapies is that, in most cases, they are non-invasive. No incisions or cuts need to be made to the skin, leaving no wounds or scars.
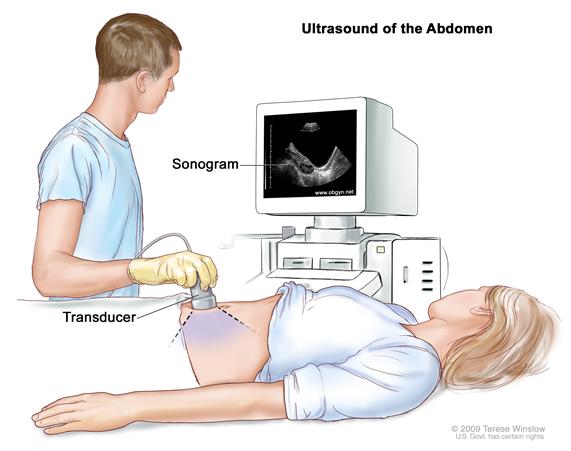
Ultrasound waves are produced by a transducer, which can both emit ultrasound waves, as well as detect the ultrasound echoes reflected back. In most cases, the active elements in ultrasound transducers are made of special ceramic crystal materials called piezoelectrics. These materials are able to produce sound waves when an electric field is applied to them, but can also work in reverse, producing an electric field when a sound wave hits them. When used in an ultrasound scanner, the transducer sends out a beam of sound waves into the body. The sound waves are reflected back to the transducer by boundaries between tissues in the path of the beam (e.g. the boundary between fluid and soft tissue or tissue and bone). When these echoes hit the transducer, they generate electrical signals that are sent to the ultrasound scanner. Using the speed of sound and the time of each echo’s return, the scanner calculates the distance from the transducer to the tissue boundary. These distances are then used to generate two-dimensional images of tissues and organs.
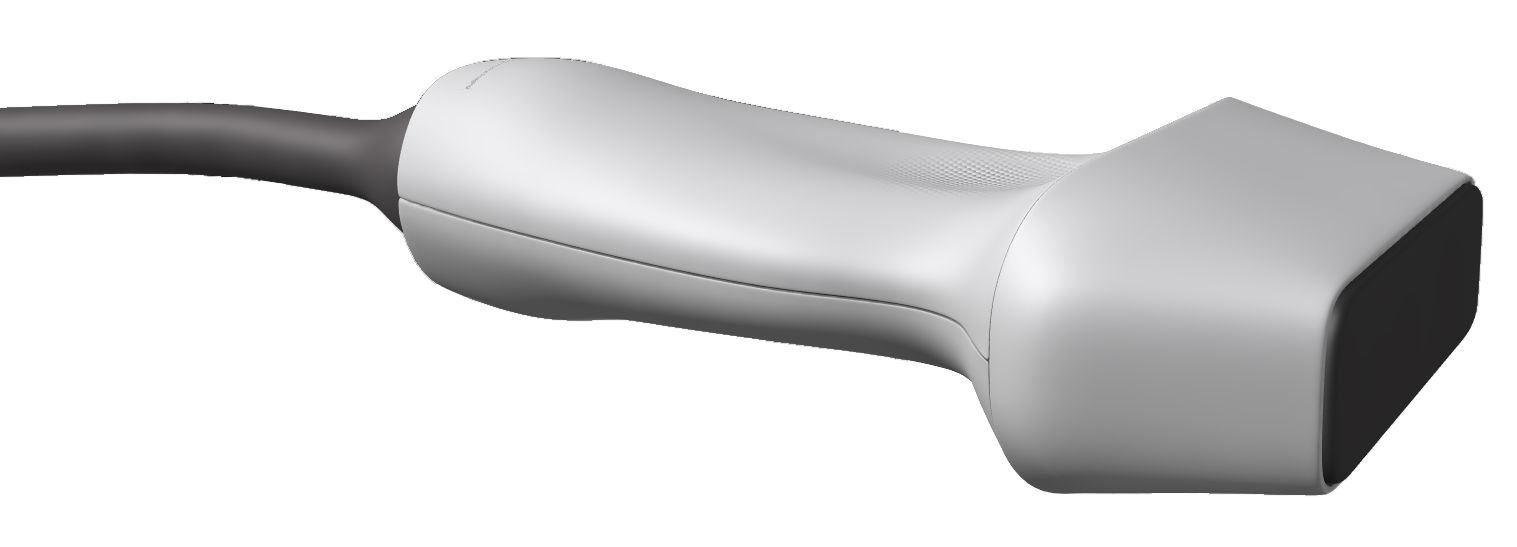
During an ultrasound exam, the technician will apply a gel to the skin. This keeps air pockets from forming between the transducer and the skin, which can block ultrasound waves from passing into the body.
Click here to watch a short video about how ultrasound works.
Diagnostic ultrasound. Diagnostic ultrasound is able to non-invasively image internal organs within the body. However, it is not good for imaging bones or any tissues that contain air, like the lungs. Under some conditions, ultrasound can image bones (such as in a fetus or in small babies) or the lungs and lining around the lungs, when they are filled or partially filled with fluid. One of the most common uses of ultrasound is during pregnancy, to monitor the growth and development of the fetus, but there are many other uses, including imaging the heart, blood vessels, eyes, thyroid, brain, breast, abdominal organs, skin, and muscles. Ultrasound images are displayed in either 2D, 3D, or 4D (which is 3D in motion).
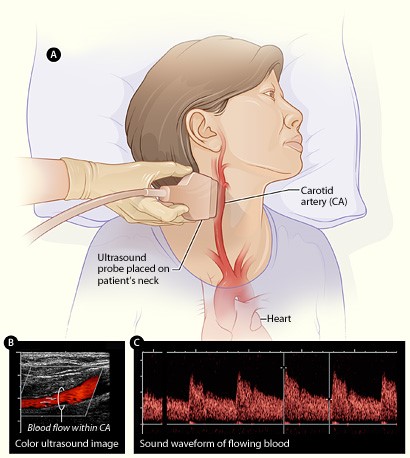
Functional ultrasound. Functional ultrasound applications include Doppler and color Doppler ultrasound for measuring and visualizing blood flow in vessels within the body or in the heart. It can also measure the speed of the blood flow and direction of movement. This is done using color-coded maps called color Doppler imaging. Doppler ultrasound is commonly used to determine whether plaque build-up inside the carotid arteries is blocking blood flow to the brain.
Another functional form of ultrasound is elastography, a method for measuring and displaying the relative stiffness of tissues, which can be used to differentiate tumors from healthy tissue. This information can be displayed as either color-coded maps of the relative stiffness; black-and white maps that display high-contrast images of tumors compared with anatomical images; or color-coded maps that are overlayed on the anatomical image. Elastography can be used to test for liver fibrosis, a condition in which excessive scar tissue builds up in the liver due to inflammation.
Ultrasound is also an important method for imaging interventions in the body. For example, ultrasound-guided needle biopsy helps physicians see the position of a needle while it is being guided to a selected target, such as a mass or a tumor in the breast. Also, ultrasound is used for real-time imaging of the location of the tip of a catheter as it is inserted in a blood vessel and guided along the length of the vessel. It can also be used for minimally invasive surgery to guide the surgeon with real-time images of the inside of the body.
Therapeutic or interventional ultrasound. Therapeutic ultrasound produces high levels of acoustic output that can be focused on specific targets for the purpose of heating, ablating, or breaking up tissue. One type of therapeutic ultrasound uses high-intensity beams of sound that are highly targeted, and is called High Intensity Focused Ultrasound (HIFU). HIFU is being investigated as a method for modifying or destroying diseased or abnormal tissues inside the body (e.g. tumors) without having to open or tear the skin or cause damage to the surrounding tissue. Either ultrasound or MRI is used to identify and target the tissue to be treated, guide and control the treatment in real time, and confirm the effectiveness of the treatment. HIFU is currently FDA approved for the treatment of uterine fibroids, to alleviate pain from bone metastases, and most recently for the ablation of prostate tissue. HIFU is also being investigated as a way to close wounds and stop bleeding, to break up clots in blood vessels, and to temporarily open the blood brain barrier so that medications can pass through.
Diagnostic ultrasound is generally regarded as safe and does not produce ionizing radiation like that produced by x-rays. Still, ultrasound is capable of producing some biological effects in the body under specific settings and conditions. For this reason, the FDA requires that diagnostic ultrasound devices operate within acceptable limits. The FDA, as well as many professional societies, discourage the casual use of ultrasound (e.g. for keepsake videos) and recommend that it be used only when there is a true medical need.
The following are examples of current research projects funded by NIBIB that are developing new applications of ultrasound that are already in use or that will be in use in the future:
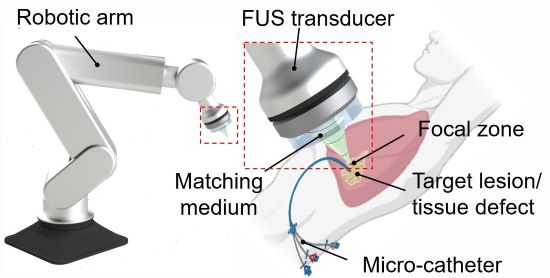
3D printing through the skin : Researchers at Duke University have developed a method to 3D print biocompatible structures through thick, multi-layered tissues. The approach entails using focused ultrasound to solidify a special ink that has been injected into the body to repair bone or repair soft tissues, for example. Initial experiments in animal tissue suggest the method could turn highly invasive surgical procedures into safer, less invasive ones. (Image on left courtesy of Junjie Yao (Duke University) and Yu Shrike Zhang (Harvard Medical School and Brigham and Women’s Hospital)).
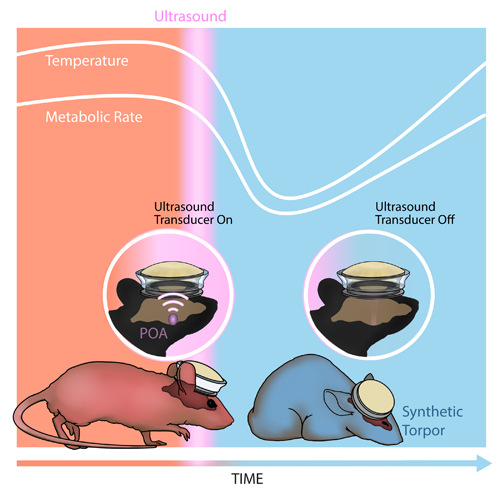
Inducing a hibernation-like state : Researchers at Washington University in St. Louis used ultrasound waves directed into the brain to lower the body temperature and metabolic rates of mice, inducing a hibernation-like state, called torpor. The researchers replicated some of these results in rats, which, like humans, don’t naturally enter torpor. Inducing torpor could help minimize damage from stroke or heart attack and buy precious time for patients in critical care. (Image on right courtesy of Yang et al./Washington University in St. Louis).
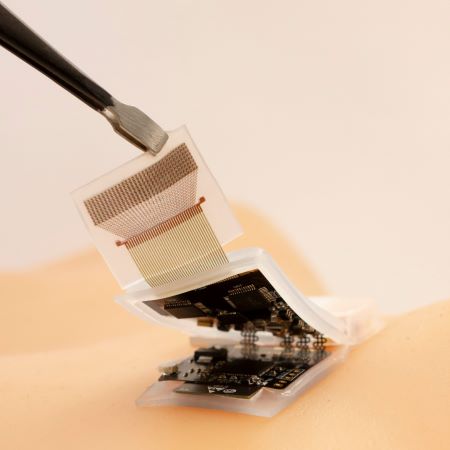
High-quality imaging at home : Brigham and Women’s Hospital researchers compared ultrasound scans acquired by experts with those taken by inexperienced volunteers, finding little difference in the image quality of the two groups. The unconventional approach of having patients take ultrasound images of themselves at home and share them with healthcare professionals could allow for remote monitoring and reduce the need for hospitalization. (Image on right courtesy of Duggan et al./Brigham and Women's Hospital).
Reviewed December 2023

Explore More
Heath Topics
- Breast Cancer
- Cardiovascular Disease
- Digestive Diseases
- Heart Disease
- Musculoskeletal Disease
- Reproductive Health
- Women's Health
Research Topics
- Optical Imaging
- Ultrasound (US) diagnostic
- Ultrasound (US) therapy
PROGRAM AREAS
- Ultrasound: Diagnostic and Interventional
Inside NIBIB
- Director's Corner
- Funding Policies
- NIBIB Fact Sheets
- Press Releases
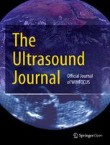
Volume 5 Supplement 1
Topics in emergency abdominal ultrasonography
Edited by Luca Brunese and Antonio Pinto
Publication of this suppement has been funded by the University of Molise, Universiy of Siena, University of Cagliari, University of Ferrara and University of Turin. The Supplement Editors declare that they have no competing interests.
Sources of error in emergency ultrasonography
To evaluate the common sources of diagnostic errors in emergency ultrasonography.
- View Full Text
Accuracy of ultrasonography in the diagnosis of acute appendicitis in adult patients: review of the literature
Ultrasound is a widely used technique in the diagnosis of acute appendicitis; nevertheless, its utilization still remains controversial.
US detection of renal and ureteral calculi in patients with suspected renal colic
The purpose of this study was to determine whether the color Doppler twinkling sign could be considered as an additional diagnostic feature of small renal lithiasis (_5mm).
Gastrointestinal perforation: ultrasonographic diagnosis
Gastrointestinal tract perforations can occur for various causes such as peptic ulcer, inflammatory disease, blunt or penetrating trauma, iatrogenic factors, foreign body or a neoplasm that require an early re...
Sigmoid diverticulitis: US findings
Acute diverticulitis (AD) results from inflammation of a colonic diverticulum. It is the most common cause of acute left lower-quadrant pain in adults and represents a common reason for acute hospitalization, ...
The role of US examination in the management of acute abdomen
Acute abdomen is a medical emergency, in which there is sudden and severe pain in abdomen of recent onset with accompanying signs and symptoms that focus on an abdominal involvement. It can represent a wide sp...
Intestinal Ischemia: US-CT findings correlations
Intestinal ischemia is an abdominal emergency that accounts for approximately 2% of gastrointestinal illnesses. It represents a complex of diseases caused by impaired blood perfusion to the small and/or large ...
US in the assessment of acute scrotum
The acute scrotum is a medical emergency . The acute scrotum is defined as scrotal pain, swelling, and redness of acute onset. Scrotal abnormalities can be divided into three groups , which are extra-testicula...
Contrast enhanced ultrasound ( CEUS ) in blunt abdominal trauma
In the assessment of polytrauma patient, an accurate diagnostic study protocol with high sensitivity and specificity is necessary. Computed Tomography (CT) is the standard reference in the emergency for evalua...
Abdominal vascular emergencies: US and CT assessment
Acute vascular emergencies can arise from direct traumatic injury to the vessel or be spontaneous (non-traumatic).
Accuracy of ultrasonography in the diagnosis of acute calculous cholecystitis: review of the literature
To evaluate the accuracy of ultrasonography in the diagnosis of acute calculous cholecystitis in comparison with other imaging modalities.
Ultrasonography (US) in the assessment of pediatric non traumatic gastrointestinal emergencies
Non traumatic gastrointestinal emergencies in the children and neonatal patient is a dilemma for the radiologist in the emergencies room and they presenting characteristics ultrasound features on the longitudi...
- Editorial Board
- Sign up for article alerts and news from this journal
- Follow us on Twitter
- Follow us on Facebook
- ISSN: 2524-8987 (electronic)
Suggestions or feedback?
MIT News | Massachusetts Institute of Technology
- Machine learning
- Social justice
- Black holes
- Classes and programs
Departments
- Aeronautics and Astronautics
- Brain and Cognitive Sciences
- Architecture
- Political Science
- Mechanical Engineering
Centers, Labs, & Programs
- Abdul Latif Jameel Poverty Action Lab (J-PAL)
- Picower Institute for Learning and Memory
- Lincoln Laboratory
- School of Architecture + Planning
- School of Engineering
- School of Humanities, Arts, and Social Sciences
- Sloan School of Management
- School of Science
- MIT Schwarzman College of Computing
Ultrasound offers a new way to perform deep brain stimulation
Press contact :.
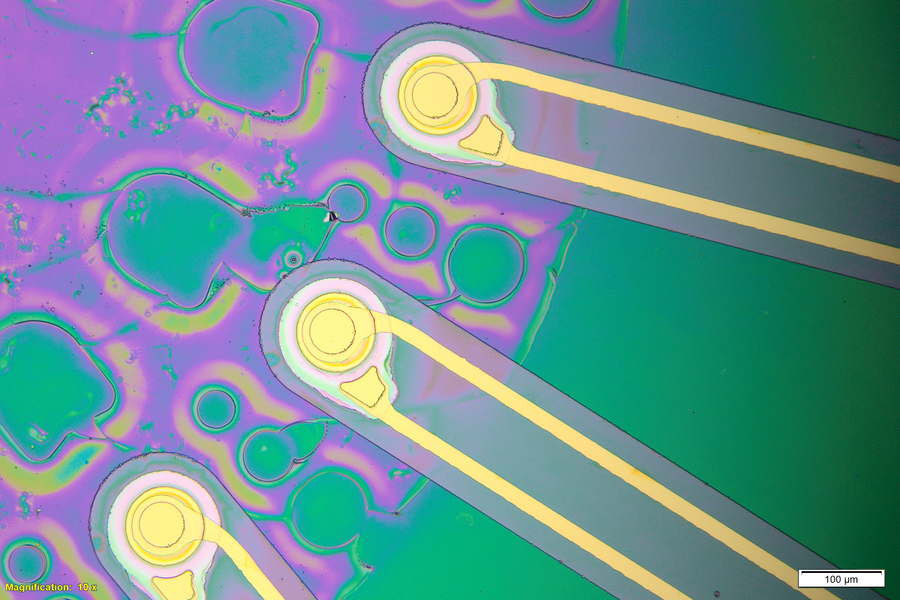
Previous image Next image
Deep brain stimulation, by implanted electrodes that deliver electrical pulses to the brain, is often used to treat Parkinson’s disease and other neurological disorders. However, the electrodes used for this treatment can eventually corrode and accumulate scar tissue, requiring them to be removed.
MIT researchers have now developed an alternative approach that uses ultrasound instead of electricity to perform deep brain stimulation, delivered by a fiber about the thickness of a human hair. In a study of mice, they showed that this stimulation can trigger neurons to release dopamine, in a part of the brain that is often targeted in patients with Parkinson’s disease.
“By using ultrasonography, we can create a new way of stimulating neurons to fire in the deep brain,” says Canan Dagdeviren, an associate professor in the MIT Media Lab and the senior author of the new study. “This device is thinner than a hair fiber, so there will be negligible tissue damage, and it is easy for us to navigate this device in the deep brain.”
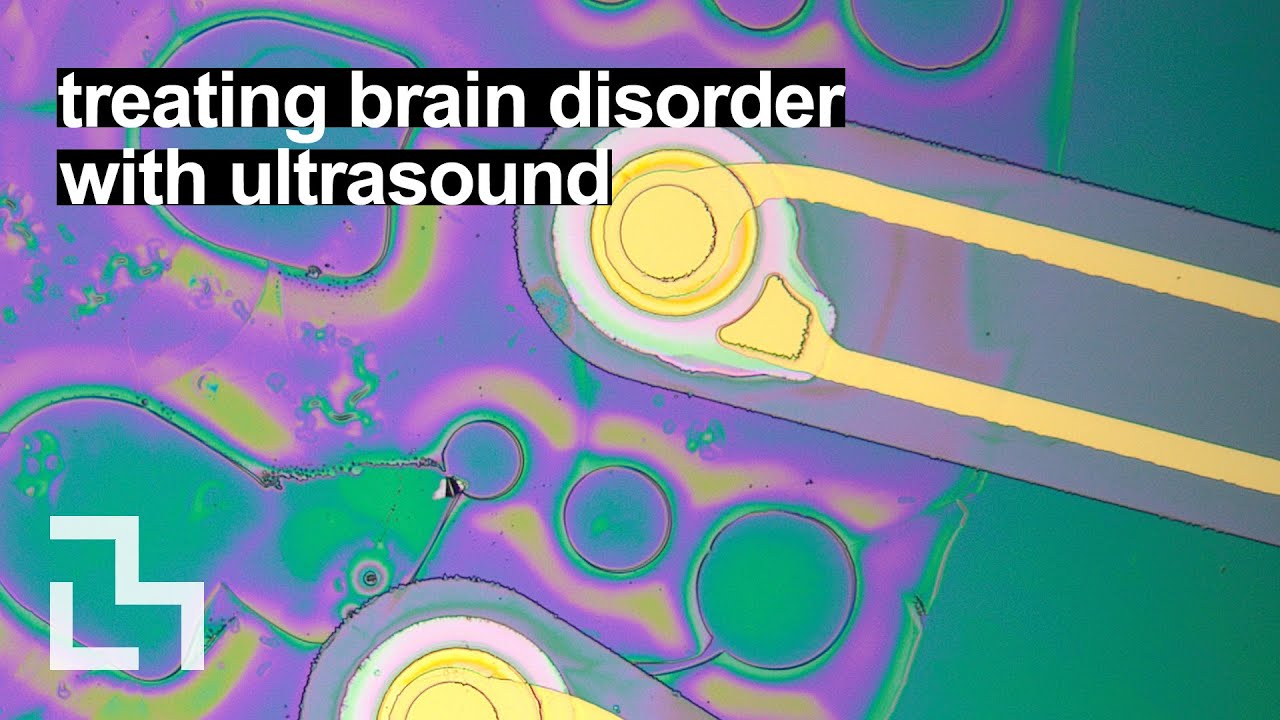
In addition to offering a potentially safer way to deliver deep brain stimulation, this approach could also become a valuable tool for researchers seeking to learn more about how the brain works.
MIT graduate student Jason Hou and MIT postdoc Md Osman Goni Nayeem are the lead authors of the paper, along with collaborators from MIT’s McGovern Institute for Brain Research, Boston University, and Caltech. The study appears today in Nature Communications .
Deep in the brain
Dagdeviren’s lab has previously developed wearable ultrasound devices that can be used to deliver drugs through the skin or perform diagnostic imaging on various organs . However, ultrasound cannot penetrate deeply into the brain from a device attached to the head or skull.
“If we want to go into the deep brain, then it cannot be just wearable or attachable anymore. It has to be implantable,” Dagdeviren says. “We carefully customize the device so that it will be minimally invasive and avoid major blood vessels in the deep brain.”
Deep brain stimulation with electrical impulses is FDA-approved to treat symptoms of Parkinson’s disease. This approach uses millimeter-thick electrodes to activate dopamine-producing cells in a brain region called the substantia nigra. However, once implanted in the brain, the devices eventually begin to corrode, and scar tissue that builds up surrounding the implant can interfere with the electrical impulses.
The MIT team set out to see if they could overcome some of those drawbacks by replacing electrical stimulation with ultrasound. Most neurons have ion channels that are responsive to mechanical stimulation, such as the vibrations from sound waves, so ultrasound can be used to elicit activity in those cells. However, existing technologies for delivering ultrasound to the brain through the skull can’t reach deep into the brain with high precision because the skull itself can interfere with the ultrasound waves and cause off-target stimulation.
“To precisely modulate neurons, we must go deeper, leading us to design a new kind of ultrasound-based implant that produces localized ultrasound fields,” Nayeem says. To safely reach those deep brain regions, the researchers designed a hair-thin fiber made from a flexible polymer. The tip of the fiber contains a drum-like ultrasound transducer with a vibrating membrane. When this membrane, which encapsulates a thin piezoelectric film, is driven by a small electrical voltage, it generates ultrasonic waves that can be detected by nearby cells.
“It’s tissue-safe, there’s no exposed electrode surface, and it’s very low-power, which bodes well for translation to patient use,” Hou says.
In tests in mice, the researchers showed that this ultrasound device, which they call ImPULS (Implantable Piezoelectric Ultrasound Stimulator), can provoke activity in neurons of the hippocampus. Then, they implanted the fibers into the dopamine-producing substantia nigra and showed that they could stimulate neurons in the dorsal striatum to produce dopamine.
“Brain stimulation has been one of the most effective, yet least understood, methods used to restore health to the brain. ImPULS gives us the ability to stimulate brain cells with exquisite spatial-temporal resolution and in a manner that doesn’t produce the kind of damage or inflammation as other methods. Seeing its effectiveness in areas like the hippocampus opened an entirely new way for us to deliver precise stimulation to targeted circuits in the brain,” says Steve Ramirez, an assistant professor of psychological and brain sciences at Boston University, and a faculty member at B.U.’s Center for Systems Neuroscience, who is also an author of the study.
A customizable device
All of the components of the device are biocompatible, including the piezoelectric layer, which is made of a novel ceramic called potassium sodium niobate, or KNN. The current version of the implant is powered by an external power source, but the researchers envision that future versions could be powered a small implantable battery and electronics unit.
The researchers developed a microfabrication process that enables them to easily alter the length and thickness of the fiber, as well as the frequency of the sound waves produced by the piezoelectric transducer. This could allow the devices to be customized for different brain regions.
“We cannot say that the device will give the same effect on every region in the brain, but we can easily and very confidently say that the technology is scalable, and not only for mice. We can also make it bigger for eventual use in humans,” Dagdeviren says.
The researchers now plan to investigate how ultrasound stimulation might affect different regions of the brain, and if the devices can remain functional when implanted for year-long timescales. They are also interested in the possibility of incorporating a microfluidic channel, which could allow the device to deliver drugs as well as ultrasound.
In addition to holding promise as a potential therapeutic for Parkinson’s or other diseases, this type of ultrasound device could also be a valuable tool to help researchers learn more about the brain, the researchers say.
“Our goal to provide this as a research tool for the neuroscience community, because we believe that we don’t have enough effective tools to understand the brain,” Dagdeviren says. “As device engineers, we are trying to provide new tools so that we can learn more about different regions of the brain.”
The research was funded by the MIT Media Lab Consortium and the Brain and Behavior Foundation Research (BBRF) NARSAD Young Investigator Award.
Share this news article on:
Related links.
- Canan Dagdeviren
- Conformable Decoders Group
- School of Architecture and Planning
Related Topics
- Neuroscience
- Brain and cognitive sciences
- Medical devices
- Parkinson's
Related Articles
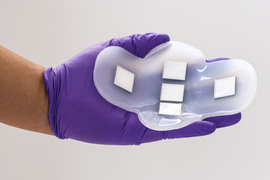
A new ultrasound patch can measure how full your bladder is
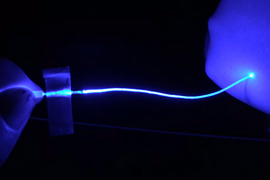
Soft optical fibers block pain while moving and stretching with the body
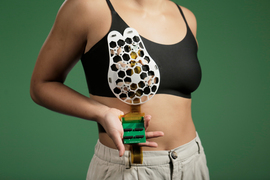
A wearable ultrasound scanner could detect breast cancer earlier
Previous item Next item
More MIT News
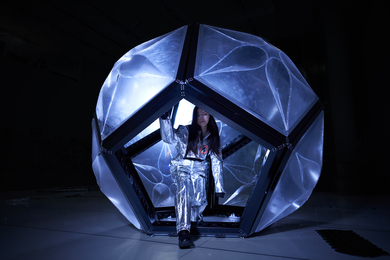
Designing for outer space
Read full story →
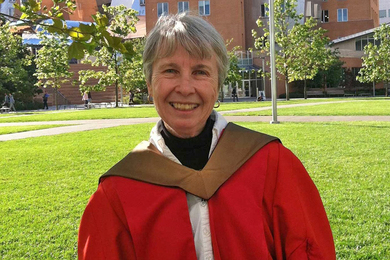
Professor Emerita Mary-Lou Pardue, pioneering cellular and molecular biologist, dies at 90

Helping nonexperts build advanced generative AI models
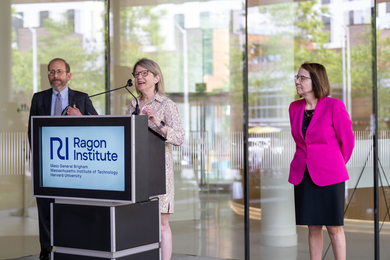
New Ragon Institute building opens in the heart of Kendall Square
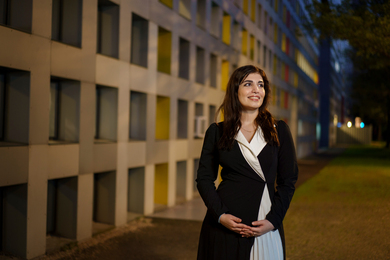
Toward socially and environmentally responsible real estate
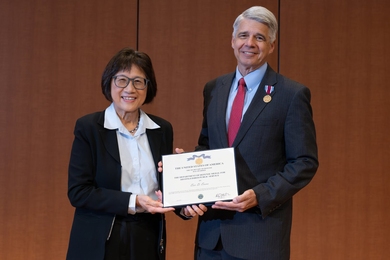
Eric Evans receives Department of Defense Medal for Distinguished Public Service
- More news on MIT News homepage →
Massachusetts Institute of Technology 77 Massachusetts Avenue, Cambridge, MA, USA
- Map (opens in new window)
- Events (opens in new window)
- People (opens in new window)
- Careers (opens in new window)
- Accessibility
- Social Media Hub
- MIT on Facebook
- MIT on YouTube
- MIT on Instagram
Evaluation of U-Nets for object segmentation in ultrasound images
Jun 20, 2024, 2:16 PM
Rui Wang, Katelyn Craft, Elisa Holtzman, Hannah Mason, Christopher Khan, Brett Byram, Jason Mitchell, and Jack H. Noble. “ Evaluation of U-Nets for Object Segmentation in Ultrasound Images .” Proceedings of SPIE Medical Imaging 2024: Ultrasonic Imaging and Tomography, vol. 12932, 129321G, 2024, San Diego, California, United States.
Ultrasound imaging is widely used in medicine due to its safety and cost-effectiveness compared to other methods. However, its quality can vary depending on tissue properties and depth. In this study, researchers tested deep learning techniques to create 3D models of objects imaged with ultrasound. They used three versions of the 3D U-Net model, each trained with different scenarios. The models performed well on specific categories of objects they were trained on but struggled with new categories. Researchers also looked into dual-task autoencoding to improve performance across different object types. These findings set a foundation for further improving the U-Net model to handle a broader range of ultrasound imaging tasks, potentially enhancing visualization and accuracy in medical applications.
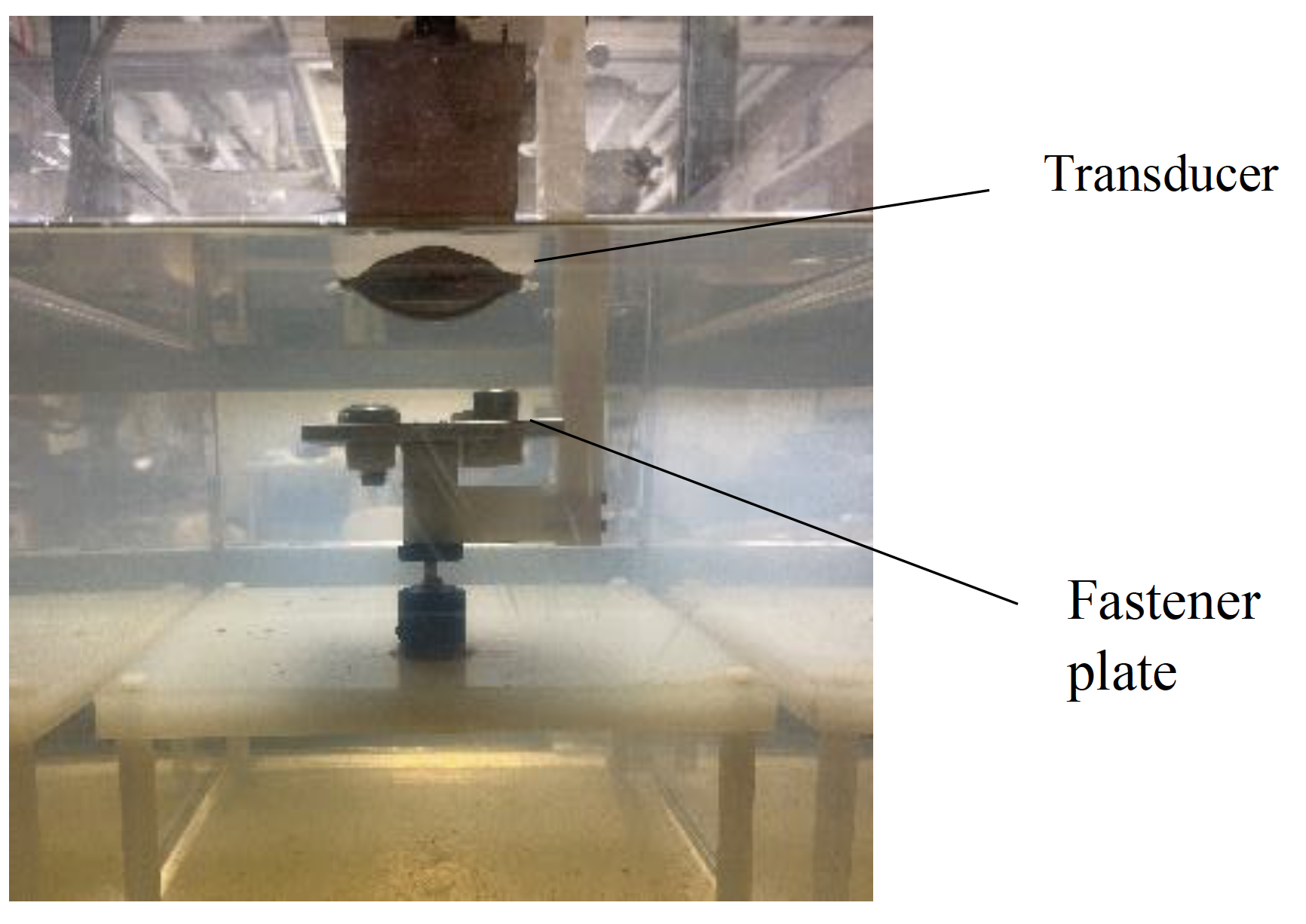
Explore Story Topics
- Deep Learning
- segmentation
Ultrasound imaging and beyond: recent advances in medical ultrasound
- Published: 14 April 2017
- Volume 7 , pages 57–58, ( 2017 )
Cite this article
- Jongbum Seo 1 &
- Young-sun Kim 2
12k Accesses
20 Citations
8 Altmetric
Explore all metrics
Avoid common mistakes on your manuscript.
Medical ultrasound represented by ultrasound imaging is generally believed to be well-known area in research, because ultrasound imaging systems has been widely distributed and actively used in the world since the 1960s. The annual world-wide use of ultrasound imaging in practice is already over billions and all pregnant women have been screened with ultrasound in some countries, such as Germany, Norway, Iceland, and Austria for years [ 1 ]. In industry, not only portable systems were available since the early 2000s, but also wireless ultrasound imaging transducers cooperating with tablet PCs were introduced in the early 2010s. Accordingly, ultrasound in medicine has been believed relatively low-tech and obsolete research field to general audience.
However, medical ultrasound has been one of the most active research areas for last 20 years and numerous outstanding break-troughs has been reported in both diagnostic and therapeutic ultrasound. In ultrasound imaging, elastography, ultrasound contrast agent imaging [ 2 ], super resolution imaging, and 2D array transducer are noteworthy. Elastic property of a given tissue can be critical for cancer diagnosis of a certain fibrous tumor and evaluation of vulnerable plaque in artery during stent implantation [ 3 ]. However, elastic characteristics cannot be clearly visualized in general ultrasound images. In order to quantitative measure of elasticity, radiation force which provides controlled deformation force on target tissue was implemented by ultrasound imaging transducer and speckle tracking algorithm which provides means of local tissue position tracking up to tens of micrometers was developed. These technologies later combined with a high frame late ultrasound imaging system whose frame late can reach up to 10,000/s and presented high resolution quantitative elastography [ 4 , 5 ]. On the other hand, super resolution imaging was achieved by simply tracking microbubbles in blood vessel with a high frame late system introduced above [ 6 ]. Since a single microbubble can be counted as a scatterer whose resolution is several orders higher than the point spread function of ultrasound system, the resultant images can show us excellent vasculature networks in deeply located organ. In order to expand these new technologies of ultrasound imaging in 3D, 2D array transducer is required, so that micro-machined transducers were also developed [ 7 ].
While researches and developments in ultrasound imaging area have been outstanding recently, novel approaches in therapeutic ultrasound have been equally impressive if not superior. 2D phased array transducers in high intensity focused ultrasound (HIFU) not only facilitate fast abdominal cancer treatment [ 8 ] but also enable to treat brain diseases without craniotomy or burr hole trephination. With the help of MRI guidance for brain treatment, ultrasound technology brought non-invasive means for selective treatment brain related disease and the result from clinical trials in tremor patients was exceptionally promising [ 9 , 10 ]. Spatially targeted drug delivery with ultrasound and microbubbles is another hot issue in medical ultrasound. Ultrasound is time varying acoustic pressure field and microbubbles in ultrasound field oscillate and/or rapidly collapse. These bubble activities are generally defined as acoustic cavitation. If cavitation activities occur nearby biological boundary which acts as a barrier, the barrier function of the boundary is temporally disturbed. Combined with the spatially selective nature of focused ultrasound, ultrasound mediated spatially targeted drug delivery with microbubbles has been a main research topic over last 20 years. As for the application, ultrasound mediated drug delivery through blood brain barrier (BBB) has been most successful in animal model experiments [ 11 , 12 ]. Although BBB opening is caught attention from general public, ultrasound mediated drug delivery has also been widely researched for the targeted cancer treatment in both body and brain. The last noteworthy ultrasound therapy is histotripsy [ 13 ]. This term is antonym to lithotripsy which indicates destruction of calcified targets such as kidney stone. In histotripsy, the target soft tissue is completely pulverized with multiple cavitations induced by repetitive high amplitude ultrasound pulses without cavitation seeds. This technique provides clear cut lines between treated tissue and surrounding tissue unlike HIFU which generally uses thermal effect and has smeared boundary. Accordingly, histotripsy can be an extremely selective tool for cancer treatment.
Although it is not mentioned above, there are additional ultrasound related research areas such as photoacoustics and ultrasound neuromodulation. Photoacoustic imaging (PAI) uses a pulsed laser excitation to generate sound in body and receives the signal with an ultrasound transducer. Since the focal spot size of laser is multiple orders smaller than that of ultrasound beam, the image resolution is phenomenally higher compared to general ultrasound images. PAI can be also easily implemented with ultrasound imaging since they can share the identical transducer [ 14 ]. Additionally, most of algorithms developed for ultrasound imaging over the decades can be directed translated to PAI and ultrasound elastography could be one of the top candidates. While most of the introduced topics have at least a specific application, ultrasound neuromodulation is in a still early stage of research to determine a certain definitive application. Since the middle of 2000s, both excitational and inhibitive neural activities induced by low intensity ultrasound have been demonstrated in the number of animal models [ 15 ]. Although the underlying mechanism has not yet been clearly understood, many of researchers expect that ultrasound neuromodulation might replace transcranial magnetic stimulation (TMS).
As described above, medical ultrasound field is boarder than general perception at least in research area. Hence, this special issue is designed to bring in various ultrasound-related topics in medicine. We also tried to invite both engineering and medical sectors to include different perspectives in medical ultrasound. Although we could not cover all the topics mentioned above, articles in this special issue will extend the general perception and will provide broader understanding on ultrasound research field.
Finally, we guest editors would like to express our gratitude to the authors who contributed in this special issue in the field of medical ultrasound.
Szabo TL. Diagnostic ultrasound imaging inside out. Amsterdam: Elsevier Academic Press; 2004.
Google Scholar
Lee H, Kimm H, Han H, Lee M, Lee S, Yoo H, Chang JH, Kim H. Microbubbles used for contrast enhanced ultrasound and theragnosis: a review of principles to applications. Biomed Eng Lett. 2017. doi: 10.1007/s13534-017-0016-5 .
Kwon SJ, Jeong MK. Advances in ultrasound elasticity imaging. Biomed Eng Lett. 2017. doi: 10.1007/s13534-017-0014-7
Sandrin L, Tanter M, Catheline S, Fink M. Shear modulus imaging with 2d transient elastography. IEEE Trans UFFC. 2002;49:426–35.
Article Google Scholar
Bruce M, Kolokythas O, Ferraioli G, Filice C, O’Donnell M. Limitations and artifacts in shear-wave elastography of the liver. Biomed Eng Lett. 2017. doi: 10.1007/s13534-017-0028-1
Errico C, Pierre J, Pezet S, Desailly Y, Lenkei Z, Couture O, Tanter M. Ultrafast ultrasound localization microscopy for deep super-resolution vascular imaging. Nature. 2015;527:499–502.
Lee W, Roh Y. Ultrasonic transducers for medical diagnostic imaging. Biomed Eng Lett. 2017. doi: 10.1007/s13534-017-0021-8 .
Kim Y. Clinical application of high-intensity focused ultrasound ablation for uterine fibroids. Biomed Eng Lett. 2017. doi: 10.1007/s13534-017-0012-9 .
Elias WJ, Lipsman N, Ondo WG, Ghanouni P, Kim YG, Lee W, Schwartz M, Hynynen K, Lozano AM, Shah BB, Huss D, Dallapiazza RF, Gwinn R, Witt J, Ro S, Eisenberg HM, Fishman PS, Gandhi D, Halpern CH, Chuang R, Pauly KB, Tierney TS, Hayes MT, Cosgrove GR, Yamaguchi T, Abe K, Taira T, Chang JW. A randomized trial of focused ultrasound thalamotomy for essential tremor. N Engl J Med. 2016;375:730–9.
Chang WS, Chang JW. Focused ultrasound treatment for central nervous system diseases: neurosurgeon’s perspectives. Biomed Eng Lett. 2017. doi: 10.1007/s13534-017-0013-8 .
Choi JJ, Pernot M, Small SA, Konofagou EE. Noninvasive, transcranial and localized opening of the blood-brain barrier using focused ultrasound in mice. Ultrasouind in Med Biol. 2007;33:95–104.
Han M, Hur Y, Hwang J, Park J. Biological effects of blood-brain barrier disruption using a focused ultrasound. Biomed Eng Lett. 2017. doi: 10.1007/s13534-017-0025-4 .
Xu Z, Raghavan M, Hall TL, Chang CW, Mycek M, Fowlkes JB, Cain CA. High speed imaging of bubble clouds generated in pulsed ultrasound cavitational therapy—histotripsy. IEEE. Trans UFFC. 2007;54:2091–101.
Park S, Jung U, Lee S, Lee D, Kim C. Contrast-enhanced dual mode imaging: photoacoustic imaging plus more. Biomed Eng Lett. 2017. doi: 10.1007/s13534-016-0006-z .
Baek H, Pahk KJ, Kim H. A review of low-intensity focused ultrasound for neuromodulation. Biomed Eng Lett. 2017. doi: 10.1007/s13534-016-0007-y .
Download references
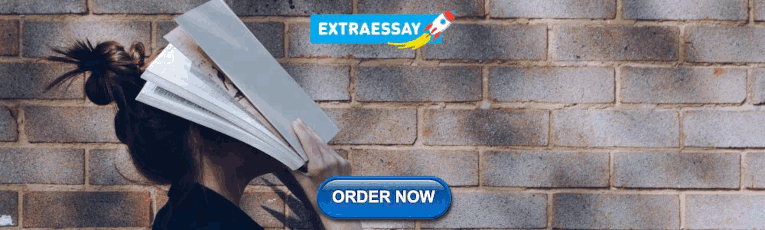
Author information
Authors and affiliations.
Department of Biomedical Engineering, Yonsei University, 304 Medical Industry Techno Tower, Wonju, Gangwon, 220-710, Republic of Korea
Jongbum Seo
Uterine Fibroid Integrated Management Center, MINT Intervention Hospital, Seoul, 26493, Republic of Korea
Young-sun Kim
You can also search for this author in PubMed Google Scholar
Corresponding authors
Correspondence to Jongbum Seo or Young-sun Kim .
Rights and permissions
Reprints and permissions
About this article
Seo, J., Kim, Ys. Ultrasound imaging and beyond: recent advances in medical ultrasound. Biomed. Eng. Lett. 7 , 57–58 (2017). https://doi.org/10.1007/s13534-017-0030-7
Download citation
Received : 04 April 2017
Revised : 09 April 2017
Accepted : 10 April 2017
Published : 14 April 2017
Issue Date : May 2017
DOI : https://doi.org/10.1007/s13534-017-0030-7
Share this article
Anyone you share the following link with will be able to read this content:
Sorry, a shareable link is not currently available for this article.
Provided by the Springer Nature SharedIt content-sharing initiative
Advertisement
- Find a journal
- Publish with us
- Track your research

An official website of the United States government
The .gov means it’s official. Federal government websites often end in .gov or .mil. Before sharing sensitive information, make sure you’re on a federal government site.
The site is secure. The https:// ensures that you are connecting to the official website and that any information you provide is encrypted and transmitted securely.
- Publications
- Account settings
- My Bibliography
- Collections
- Citation manager
Save citation to file
Email citation, add to collections.
- Create a new collection
- Add to an existing collection
Add to My Bibliography
Your saved search, create a file for external citation management software, your rss feed.
- Search in PubMed
- Search in NLM Catalog
- Add to Search
Seeing is believing: Smart use of musculoskeletal ultrasound in rheumatology practice
Affiliations.
- 1 Division of Rheumatology, Women's College Hospital, Toronto, ON, Canada; Department of Medicine, University of Toronto, Toronto, ON, Canada.
- 2 Departamento de Inmunologia Clinica y Reumatologia, Pontificia Universidad Catolica de Chile, Santiago, Chile.
- 3 Division of Rheumatology and Clinical Immunology, University of Florida College of Medicine, Jacksonville, FL, USA.
- 4 Division of Rheumatology, Women's College Hospital, Toronto, ON, Canada; Department of Medicine, University of Toronto, Toronto, ON, Canada. Electronic address: [email protected].
- PMID: 37481369
- DOI: 10.1016/j.berh.2023.101850
Musculoskeletal ultrasonography has become an increasingly valuable tool as a complement to the physical exam in rheumatology practice. Its point-of-care access, low cost, safety, portability, and reliability in trained hands, make this technique especially useful in patients with inflammatory arthritis. Growing evidence has demonstrated the value of musculoskeletal ultrasound in the detection of inflammatory and structural changes in patients with joint pain without obvious joint swelling, in differentiating various inflammatory diagnoses, in the monitoring of inflammatory arthritis, and interventional procedures. The potential role of ultrasound guiding treat-to-target strategies or tapering treatment in inflammatory arthritis requires further research. However, musculoskeletal ultrasound can also have pitfalls and limitations that a clinician should be aware of.
Keywords: Gout; Osteoarthritis; Psoriatic arthritis; Rheumatoid arthritis; Ultrasonography.
Copyright © 2023 Elsevier Ltd. All rights reserved.
PubMed Disclaimer
Conflict of interest statement
Declaration of competing interest The authors declare that they have no known competing financial interests or personal relationships that could have appeared to influence the work reported in this paper.
Similar articles
- Magnetic Resonance Imaging in Rheumatology. Lambert RGW, Østergaard M, Jaremko JL. Lambert RGW, et al. Magn Reson Imaging Clin N Am. 2018 Nov;26(4):599-613. doi: 10.1016/j.mric.2018.06.008. Magn Reson Imaging Clin N Am. 2018. PMID: 30316470 Review.
- One year in review: ultrasound in arthritis. Naredo E, Iagnocco A. Naredo E, et al. Clin Exp Rheumatol. 2016 Jan-Feb;34(1):1-10. Epub 2016 Feb 10. Clin Exp Rheumatol. 2016. PMID: 26892798 Review.
- Optimising ultrasonography in rheumatology. Keen HI, Wakefield R, Conaghan PG. Keen HI, et al. Clin Exp Rheumatol. 2014 Sep-Oct;32(5 Suppl 85):S-13-6. Epub 2014 Oct 30. Clin Exp Rheumatol. 2014. PMID: 25365083 Review.
- Ultrasound imaging in psoriatic arthritis and ankylosing spondylitis. Riente L, Carli L, Delle Sedie A. Riente L, et al. Clin Exp Rheumatol. 2014 Jan-Feb;32(1 Suppl 80):S26-33. Epub 2014 Feb 17. Clin Exp Rheumatol. 2014. PMID: 24528804
- Sensitivity of standardised musculoskeletal examination of the hand and wrist joints in detecting arthritis in comparison to ultrasound findings in patients attending rheumatology clinics. Almoallim H, Attar S, Jannoudi N, Al-Nakshabandi N, Eldeek B, Fathaddien O, Halabi H. Almoallim H, et al. Clin Rheumatol. 2012 Sep;31(9):1309-17. doi: 10.1007/s10067-012-2013-5. Epub 2012 Jun 7. Clin Rheumatol. 2012. PMID: 22673791
- The power of us: breaking barriers and bridging the gap of ultrasound in rheumatology to empower a new generation. Garcia-Pompermayer MR, Ayton SG, Molina-Collada J, Tamborrini G, Sanchez MED, Luna KS, Elizondo MAG. Garcia-Pompermayer MR, et al. Clin Rheumatol. 2024 Jun;43(6):2103-2116. doi: 10.1007/s10067-024-06973-w. Epub 2024 Apr 23. Clin Rheumatol. 2024. PMID: 38653847
Publication types
- Search in MeSH
Related information
Linkout - more resources, full text sources.
- Elsevier Science
- MedlinePlus Health Information
- Citation Manager
NCBI Literature Resources
MeSH PMC Bookshelf Disclaimer
The PubMed wordmark and PubMed logo are registered trademarks of the U.S. Department of Health and Human Services (HHS). Unauthorized use of these marks is strictly prohibited.
share this!
June 19, 2024
This article has been reviewed according to Science X's editorial process and policies . Editors have highlighted the following attributes while ensuring the content's credibility:
fact-checked
trusted source
Ultrasound beam triggers 'nanodroplets' to deliver drugs at exactly the right spot
by Frontiers
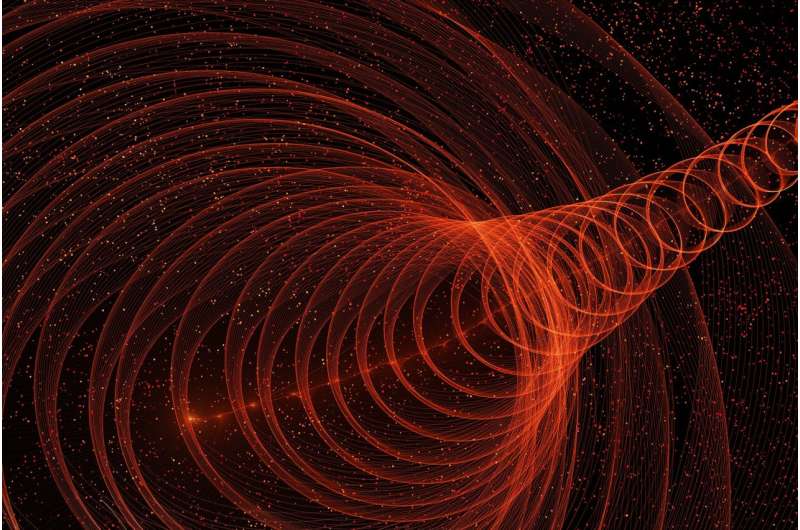
Conventional drug delivery is often like cracking a nut with a sledgehammer. Whether the drug is swallowed, injected, inhaled, or absorbed through the skin, it ultimately diffuses to most parts of the body, including those where it isn't needed—or where it might even cause harm.
But what if the delivery could be targeted at exactly the right spot? This would allow the total dose to be dramatically lower, thus minimizing side-effects.
Now, scientists from the US have found a way to perfect a promising, emerging method that does just that. With their new protocol, the method is made both safe and efficient for the first time, hopefully paving the way for first-in-human trials. The results are published in Frontiers in Molecular Biosciences .
"Here we show a method to deliver drugs to specific areas of the body where they are needed. We do so using ultrasound waves , which trigger drug release from circulating nanocarriers when focused on the target," said Matthew G Wilson, a graduate research assistant at the University of Utah, and the study's first author. "We developed a method to produce stable nanocarriers repeatably, and identified ultrasound parameters that can activate them."
Nanoengineered droplets
The nanocarriers are minuscule droplets, between 470 and 550 nanometers across, with a hollow outer shell composed of polymer molecules. These polymers have two distinct ends: a hydrophilic one, which mixes well with watery solutions like blood and which faces outward, and a hydrophobic one that doesn't mix with water and which faces inwards.
Within the shell is an inner core of hydrophobic perfluorocarbons, molecules that consist mostly of fluorine and carbon, and that are mixed with an equally hydrophobic drug of interest. The shells keep the cores apart, preventing them from coalescing into a single droplet, and form a barrier against the immune system. The effect is much like mayonnaise, where proteins from eggs form droplets of encapsulated oils, where otherwise the oil and water would separate completely.
To release the drug, the researchers played back an ultrasound—a sound wave with a frequency beyond the upper limit of human hearing—of 300 or 900 kilohertz. The beam of ultrasound can be steered across three dimensions, to focus on a desired area within the body that is only a few millimeters across.
The ultrasound is thought to cause the perfluorocarbons to expand, stretching out the droplet's shell and making it more permeable to the drug, which then diffuses out to the organs, tissues, or cells where it is required.
The researchers compared the efficiency of delivery of a representative drug—the anesthetic and sedative propofol—between three different perfluorocarbons, perfluoropentane (PFP), decafluoropentane (DFP), and perfluorooctylbromide (PFOB). The ultrasound was delivered to the nanodroplets in vitro, in 60 pulses of 100 milliseconds over one minute.
Reaching the boiling point
The results showed that the balance between stability of the nanodroplets and the efficiency of delivery was optimal for PFOB cores.
"Previous studies have focused on perfluorocarbons with low boiling points—usually lower than the human body temperature. We found that droplets with a PFOB core, which has a boiling point of 142 °C, are much more stable over time," explained Wilson.
"Despite its high boiling point, PFOB can achieve similar levels of drug release when low-frequency ultrasound of 300 kilohertz is applied. The ultrasound frequency turned out to be a critical factor in our study."
To test for safety, the researchers injected a single long-tailed macaque with six doses of PFOB-based nanodroplets at one-week intervals, and monitored the evolution of a range of blood biomarkers for liver, kidney, and immune response function. This experiment, which had been approved by University of Utah Institutional Animal Care and Use Committee, showed that the nanodroplets were well tolerated, with no detectable side effects. These experiments must be replicated in microdosing or Phase I trials on human volunteers.
The authors also published their protocol for the production of the nanodroplets as open science, so other research groups can learn directly from their findings.
"The method we developed can be applied to any of a variety of conditions depending on the drug used. For psychiatric applications, localized delivery of propofol could be used as a diagnostic tool to identify brain regions causally involved in disorders for individual patients. For more lasting treatment, ketamine delivery could be a potent method to rewire neural circuits ," concluded Wilson's academic supervisor, Dr. Jan Kubanek, an assistant professor at the University of Utah, and the study's senior author.
Provided by Frontiers
Explore further
Feedback to editors

Saturday Citations: Bulking tips for black holes; microbes influence drinking; new dinosaur just dropped
Jun 22, 2024

China, France launch satellite to better understand the universe

Key mechanism in nuclear reaction dynamics promises advances in nuclear physics
Jun 21, 2024

Study challenges popular idea that Easter islanders committed 'ecocide'

New AI-driven tool improves root image segmentation

Many more bacteria produce greenhouse gases than previously thought, study finds

Stacking three layers of graphene with a twist speeds up electrochemical reactions

A black hole of inexplicable mass: JWST observations reveal a mature quasar at cosmic dawn

Beyond CRISPR: seekRNA delivers a new pathway for accurate gene editing

Transforming drug discovery with AI: New program transforms 3D information into data that typical models can use
Relevant physicsforums posts, is meat broth really nutritious.
16 hours ago
COVID Virus Lives Longer with Higher CO2 In the Air
Periodical cicada life cycle, a dna animation.
Jun 15, 2024
Innovative ideas and technologies to help folks with disabilities
Jun 14, 2024
How do fetuses breathe in the womb?
More from Biology and Medical
Related Stories

How ultrasound and microbubbles could deliver immunotherapy to the brain
May 9, 2024

Scientists harness ultrasound for drug delivery and tissue implantation
Apr 22, 2024

Nanodroplets and ultrasound 'drills' prove effective at tackling tough blood clots
Jan 7, 2021

Millimetre-precision drug delivery to the brain
Oct 5, 2020

Vibrating nanodroplets may invade a tumor
Feb 4, 2021

Non-invasive method to deliver drugs to the brain
Feb 4, 2022
Recommended for you

Advances in nanoscale force measurement opens doors to unprecedented biological insights

Mini liver model innovations promise more effective drug testing
Jun 20, 2024

Researchers fabricate eco-friendly pesticide delivery system
Jun 19, 2024

Lung-targeting lipid nanoparticles with CRISPR components successfully treat cystic fibrosis mouse models
Jun 18, 2024

Scientists use tyrosine nanomedicine to halt melanoma growth

Self-assembling and disassembling swarm molecular robots via DNA molecular controller
Let us know if there is a problem with our content.
Use this form if you have come across a typo, inaccuracy or would like to send an edit request for the content on this page. For general inquiries, please use our contact form . For general feedback, use the public comments section below (please adhere to guidelines ).
Please select the most appropriate category to facilitate processing of your request
Thank you for taking time to provide your feedback to the editors.
Your feedback is important to us. However, we do not guarantee individual replies due to the high volume of messages.
E-mail the story
Your email address is used only to let the recipient know who sent the email. Neither your address nor the recipient's address will be used for any other purpose. The information you enter will appear in your e-mail message and is not retained by Phys.org in any form.
Newsletter sign up
Get weekly and/or daily updates delivered to your inbox. You can unsubscribe at any time and we'll never share your details to third parties.
More information Privacy policy
Donate and enjoy an ad-free experience
We keep our content available to everyone. Consider supporting Science X's mission by getting a premium account.
E-mail newsletter
Combining mammography with ultrasound tomography improves cancer detection
Combining full-field digital mammography with ultrasound tomography can improve breast cancer detection and be helpful in imaging dense breasts, according to research published June 18 in Radiology .
A team led by Mary Yamashita, MD, from the University of Southern California in Los Angeles, found that the combined method outperformed full-field digital mammography by itself, including having higher sensitivity for BI-RADS 4 lesions and higher specificity for BI-RADS 3 lesions.
“Our study suggests ultrasound tomography can be another supplemental screening tool,” Yamashita and colleagues wrote.
Previous studies suggest that women with dense breasts benefit from supplemental ultrasound imaging. However, ultrasound has low specificity and automated breast ultrasound (ABUS) prolongs interpretation time.
However, ultrasound tomography provides four coronal volumetric image sequences of B mode and quantitative tissue characterization information. This includes tissue stiffness, speed of sound, and attenuation.
“By merging reflection images with these parameters, ultrasound tomography can potentially differentiate cancer from normal tissue or benign disease in women with dense breasts,” the researchers wrote.
Yamashita and colleagues evaluated the performance of ultrasound tomography combined with full-field digital mammography and compared it with that of full-field digital mammography alone for screening women with dense breasts.
The study included data collected between 2017 and 2019 from 140 women who underwent both modalities at 10 centers as part of a prospective case collection registry. For the study, 32 radiologists who are qualified under the Mammography Quality Standards Act independently evaluated full-field digital mammograms followed immediately by mammography plus ultrasound tomography for suspicious findings and assigned a BI-RADS category.
Of the 140 women (average age, 56 years), 36 had breast cancer and 104 did not.
The team reported that the combined approach demonstrated superior performance compared with mammography alone. Additionally, combining mammography with ultrasound tomography led to superior sensitivity in BI-RADS 4 lesions and superior specificity in BI-RADS 3 lesions.
Performance of full-field digital mammography plus ultrasound tomography, full-field digital mammography alone | |||
---|---|---|---|
Measure | Mammography alone | Combined approach | p-value |
AUC | 0.54 | 0.6 | 0.03 |
Sensitivity (BI-RADS 4) | 30% | 37% | 0.03 |
Specificity (BI-RADS 4) | 88% | 82% | 0.004 |
Sensitivity (BI-RADS 3) | 33% | 40% | 0.08 |
Specificity (BI-RADS 3) | 69% | 75% | 0.04 |
The radiologists performed 4,480 readings using both the combined approach and mammography alone. Of the readings, 4,475 combined approach readings and 4,332 mammography-alone readings took less than 30 minutes to complete.
The team reported that among images read in 30 minutes or less, the median reading time was 3.4 minutes for mammography plus ultrasound tomography and 1.2 minutes for mammography alone. However, for mammography plus ultrasound tomography, images with normal findings had a median reading time of 3.2 minutes compared to 3.9 minutes for mammography alone.
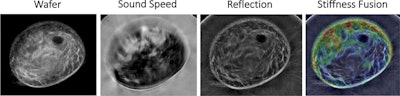
The study authors wrote that while their results indicate ultrasound tomography’s utility in this area, more studies are needed to evaluate the modality’s benefits. This includes determining whether ultrasound tomography can improve cancer detection without increasing the number of benign biopsies.
In an accompanying editorial, Ritse Mann, MD, PhD, from Radboud University Medical Center in Nijmegen, the Netherlands, wrote that the results demonstrate ultrasound tomography being “at best on par” with handheld ultrasound and automated breast ultrasound. This includes allowing detection of some additional cancers at the price of a substantial increase in the number of false-positive findings.
“It seems that ultrasound tomography may be a new implementation of ultrasound, but it unfortunately yields old results,” Mann wrote.
Mann added that it remains to be seen whether “the promise of ultrasound can ever be delivered and whether ultrasound tomography has a role in that.”
The full results can be found here .
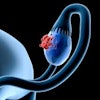
MRI shows ties between ovary removal and brain function later in life
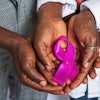
Uniting for health equity: Addressing breast cancer disparities
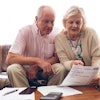
Patients who 'maximize' medical use have more worry about cancer
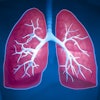
Women favorable toward paired lung, breast cancer screening
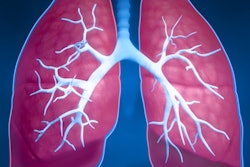
AI reduces breast radiologist workload, false-positive cases
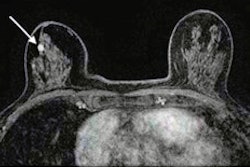
Abbreviated MRI suitable in subsequent screening of dense breasts
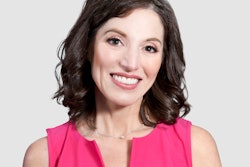
Delphinus names Elise Berman as medical director
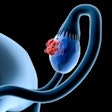
Food insecurity tied to lower likelihood of mammography screening
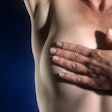
LGBTQ+ individuals show decreased breast cancer screening rates
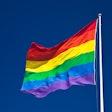
Critics slam Canadian breast screening guidelines
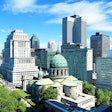
Updated Canadian breast cancer guidelines recommend biennial screening
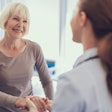
Weekend, evening mammo appointments may increase screening access
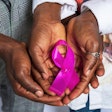
Consideration needed in breast cancer screening for young women
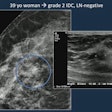
Information
- Author Services
Initiatives
You are accessing a machine-readable page. In order to be human-readable, please install an RSS reader.
All articles published by MDPI are made immediately available worldwide under an open access license. No special permission is required to reuse all or part of the article published by MDPI, including figures and tables. For articles published under an open access Creative Common CC BY license, any part of the article may be reused without permission provided that the original article is clearly cited. For more information, please refer to https://www.mdpi.com/openaccess .
Feature papers represent the most advanced research with significant potential for high impact in the field. A Feature Paper should be a substantial original Article that involves several techniques or approaches, provides an outlook for future research directions and describes possible research applications.
Feature papers are submitted upon individual invitation or recommendation by the scientific editors and must receive positive feedback from the reviewers.
Editor’s Choice articles are based on recommendations by the scientific editors of MDPI journals from around the world. Editors select a small number of articles recently published in the journal that they believe will be particularly interesting to readers, or important in the respective research area. The aim is to provide a snapshot of some of the most exciting work published in the various research areas of the journal.
Original Submission Date Received: .
- Active Journals
- Find a Journal
- Proceedings Series
- For Authors
- For Reviewers
- For Editors
- For Librarians
- For Publishers
- For Societies
- For Conference Organizers
- Open Access Policy
- Institutional Open Access Program
- Special Issues Guidelines
- Editorial Process
- Research and Publication Ethics
- Article Processing Charges
- Testimonials
- Preprints.org
- SciProfiles
- Encyclopedia
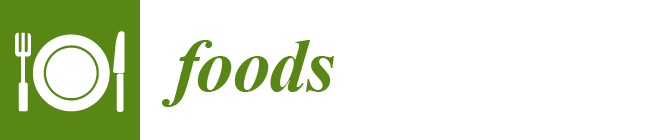
Article Menu
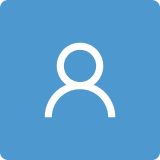
- Subscribe SciFeed
- Recommended Articles
- Google Scholar
- on Google Scholar
- Table of Contents
Find support for a specific problem in the support section of our website.
Please let us know what you think of our products and services.
Visit our dedicated information section to learn more about MDPI.
JSmol Viewer
Effect of multi-mode divergent ultrasound pretreatment on hardness, microstructure and digestion of acid-induced whey protein gels.
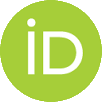
1. Introduction
2. materials and methods, 2.1. materials, 2.2. ultrasound pretreatment on whey protein, 2.3. whey protein gels (wpg) preparation, 2.4. rheological properties analysis, 2.5. turbidity determination, 2.6. gel solubility, 2.7. gel hardness, 2.8. scan electron microscopy (sem), 2.9. sodium dodecyl sulfate-polyacrylamide gel electrophoresis (sds-page), 2.10. simulated digestion of wpg, 2.11. particle size distribution of the solid digesta, 2.12. free amino group determination, 2.13. statistical analysis, 3.1. effect of ultrasonic frequency mode on the viscosity of whey protein solution, 3.2. hardness of wpg, 3.3. rheological properties of wpg, 3.4. protein distribution in whey protein gel, 3.5. turbidity and chemical forces, 3.5.1. turbidity, 3.5.2. chemical forces, 3.6. microstructure of gdl-induced whey protein gel, 3.7. digestion of gdl-induced whey protein gel, 3.7.1. physical digestion of wpg, 3.7.2. chemical digestion of wpg, 4. conclusions, author contributions, institutional review board statement, informed consent statement, data availability statement, conflicts of interest.
- Cao, Y.P.; Mezzenga, R. Design principles of food gels. Nat. Food 2020 , 1 , 106–118. [ Google Scholar ] [ CrossRef ]
- Hebishy, E.; Du, H.; Brito-Oliveira, T.C.; Pinho, S.C.; Miao, S. Saltiness perception in gel-based food systems (gels and emulsion-filled gels). Crit. Rev. Food Sci. Nutr. 2023 , 1–18. [ Google Scholar ] [ CrossRef ]
- Nascimento, L.G.L.; Odelli, D.; de Carvalho, A.F.; Martins, E.; Delaplace, G.; Peixoto, P.P.D.; Silva, N.F.N.; Casanova, F. Combination of Milk and Plant Proteins to Develop Novel Food Systems: What Are the Limits? Foods 2023 , 12 , 2385. [ Google Scholar ] [ CrossRef ]
- Teimouri, S.; Kasapis, S.; Dokouhaki, M. Diffusional characteristics of food protein-based materials as nutraceutical delivery systems: A review. Trends Food Sci. Technol. 2022 , 122 , 201–210. [ Google Scholar ] [ CrossRef ]
- Siddiqui, S.A.; Alvi, T.; Biswas, A.; Shityakov, S.; Gusinskaia, T.; Lavrentev, F.; Dutta, K.; Khan, M.K.I.; Stephen, J.; Radhakrishnan, M. Food gels: Principles, interaction mechanisms and its microstructure. Crit. Rev. Food Sci. Nutr. 2023 , 63 , 12530–12551. [ Google Scholar ] [ CrossRef ]
- Abaee, A.; Mohammadian, M.; Jafari, S.M. Whey and soy protein-based hydrogels and nano-hydrogels as bioactive delivery systems. Trends Food Sci. Technol. 2017 , 70 , 69–81. [ Google Scholar ] [ CrossRef ]
- Cheng, Q.; Liu, C.; Zhao, J.; Guo, F.; Qin, J.; Wang, Y. Hyaluronic acid modulates techno-functional and digestion properties of heat-induced ginkgo seed protein isolate gel. Food Biosci. 2024 , 59 , 104204. [ Google Scholar ] [ CrossRef ]
- Cheng, Y.; Ye, A.Q.; Singh, H. Characterizations of emulsion gel formed with the mixture of whey and soy protein and its protein digestion under in vitro gastric conditions. Curr. Res. Food Sci. 2024 , 8 , 10. [ Google Scholar ] [ CrossRef ]
- Grasberger, K.; Hammershoj, M.; Corredig, M. Lupin protein-stabilized oil droplets contribute to structuring whey protein emulsion-filled gels. Food Res. Int. 2024 , 178 , 10. [ Google Scholar ] [ CrossRef ]
- Zhang, L.Y.; Ge, H.F.; Zhao, J.; Liu, C.Q.; Wang, Y.S. L-Theanine Improves the Gelation of Ginkgo Seed Proteins at Different pH Levels. Gels 2024 , 10 , 131. [ Google Scholar ] [ CrossRef ]
- Chen, W.Q.; Ding, Y.H.; Zhao, Y.M.; Ma, H.L. Strategies to improve the emulsification properties of rice proteins as a promising source of plant-based emulsifiers: An updated mini-review. Food Biosci. 2023 , 53 , 8. [ Google Scholar ] [ CrossRef ]
- Kang, D.C.; Zhang, W.A.; Lorenzo, J.M.; Chen, X. Structural and functional modification of food proteins by high power ultrasound and its application in meat processing. Crit. Rev. Food Sci. Nutr. 2021 , 61 , 1914–1933. [ Google Scholar ] [ CrossRef ]
- Pinton, M.B.; dos Santos, B.A.; Lorenzo, J.P.M.; Cichoski, A.J.P.; Boeira, C.P.; Campagnol, P.C.B. Green technologies as a strategy to reduce NaCl and phosphate in meat products: An overview. Curr. Opin. Food Sci. 2021 , 40 , 1–5. [ Google Scholar ] [ CrossRef ]
- Taha, A.; Mehany, T.; Pandiselvam, R.; Siddiqui, S.A.; Mir, N.A.; Malik, M.A.; Sujayasree, O.J.; Alamuru, K.C.; Khanashyam, A.C.; Casanova, F.; et al. Sonoprocessing: Mechanisms and recent applications of power ultrasound in food. Crit. Rev. Food Sci. Nutr. 2022 , 1–39. [ Google Scholar ] [ CrossRef ]
- Xu, B.G.; Tiliwa, E.S.; Yan, W.Q.; Azam, S.M.R.; Wei, B.X.; Zhou, C.S.; Ma, H.L.; Bhandari, B. Recent development in high quality drying of fruits and vegetables assisted by ultrasound: A review. Food Res. Int. 2022 , 152 , 19. [ Google Scholar ] [ CrossRef ]
- Bhangu, S.K.; Ashokkumar, M. Theory of Sonochemistry. Top. Curr. Chem. 2016 , 374 , 28. [ Google Scholar ] [ CrossRef ]
- Chandrapala, J.; Oliyer, C.; Kentish, S.; Ashokkumar, M. Ultrasonics in food processing. Ultrason. Sonochem. 2012 , 19 , 975–983. [ Google Scholar ] [ CrossRef ]
- Yusof, N.S.M.; Anandan, S.; Sivashanmugam, P.; Flores, E.M.M.; Ashokkumar, M. A correlation between cavitation bubble temperature, sonoluminescence and interfacial chemistry—A minireview. Ultrason. Sonochem. 2022 , 85 , 10. [ Google Scholar ] [ CrossRef ]
- Chen, H.L.; Xu, B.G.; Zhou, C.S.; Yagoub, A.A.; Cai, Z.; Yu, X.J. Multi-frequency ultrasound-assisted dialysis modulates the self-assembly of alcohol-free zein-sodium caseinate to encapsulate curcumin and fabricate composite nanoparticles. Food Hydrocoll. 2022 , 122 , 14. [ Google Scholar ] [ CrossRef ]
- Dabbour, M.; Jiang, H.; Mintah, B.K.; Wahia, H.; He, R.H. Ultrasonic-assisted protein extraction from sunflower meal: Kinetic modeling, functional, and structural traits. Innov. Food Sci. Emerg. Technol. 2021 , 74 , 10. [ Google Scholar ] [ CrossRef ]
- Qayum, A.; Rashid, A.; Liang, Q.F.; Wu, Y.; Cheng, Y.; Kang, L.X.; Liu, Y.X.; Zhou, C.W.; Hussain, M.; Ren, X.F.; et al. Ultrasonic and homogenization: An overview of the preparation of an edible protein-polysaccharide complex emulsion. Compr. Rev. Food. Sci. Food Saf. 2023 , 22 , 4242–4281. [ Google Scholar ] [ CrossRef ]
- Chen, W.Q.; Ma, H.L.; Wang, Y.Y. Recent advances in modified food proteins by high intensity ultrasound for enhancing functionality: Potential mechanisms, combination with other methods, equipment innovations and future directions. Ultrason. Sonochem. 2022 , 85 , 12. [ Google Scholar ] [ CrossRef ]
- Glover, Z.J.; Gregersen, S.B.; Wiking, L.; Hammershoj, M.; Simonsen, A.C. Microstructural changes in acid milk gels due to temperature-controlled high-intensity ultrasound treatment: Quantification by analysis of super-resolution microscopy images. Int. J. Dairy Technol. 2022 , 75 , 321–328. [ Google Scholar ] [ CrossRef ]
- Bangar, S.P.; Esua, O.J.; Sharma, N.; Thirumdas, R. Ultrasound-assisted modification of gelation properties of proteins: A review. J. Texture Stud. 2022 , 53 , 763–774. [ Google Scholar ] [ CrossRef ]
- Wang, Q.; Gu, C.; Wei, R.; Luan, Y.; Liu, R.; Ge, Q.; Yu, H.; Wu, M. Enhanced gelling properties of myofibrillar protein by ultrasound-assisted thermal-induced gelation process: Give an insight into the mechanism. Ultrason. Sonochem. 2023 , 94 , 106349. [ Google Scholar ] [ CrossRef ]
- Ding, Y.H.; Ma, H.L.; Wang, K.; Azam, S.M.R.; Wang, Y.Y.; Zhou, J.; Qu, W.J. Ultrasound frequency effect on soybean protein: Acoustic field simulation, extraction rate and structure. LWT-Food Sci. Technol. 2021 , 145 , 10. [ Google Scholar ] [ CrossRef ]
- Xu, B.G.; Azam, S.M.R.; Feng, M.; Wu, B.G.; Yan, W.Q.; Zhou, C.S.; Ma, H.L. Application of multi-frequency power ultrasound in selected food processing using large-scale reactors: A review. Ultrason. Sonochem. 2021 , 81 , 15. [ Google Scholar ] [ CrossRef ]
- Shen, X.; Zhao, C.H.; Guo, M.R. Effects of high intensity ultrasound on acid-induced gelation properties of whey protein gel. Ultrason. Sonochem. 2017 , 39 , 810–815. [ Google Scholar ] [ CrossRef ]
- Gregersen, S.B.; Wiking, L.; Hammershoj, M. Acceleration of acid gel formation by high intensity ultrasound is linked to whey protein denaturation and formation of functional milk fat globule-protein complexes. J. Food Eng. 2019 , 254 , 17–24. [ Google Scholar ] [ CrossRef ]
- Shi, R.J.; Li, T.; Wang, K.L.; He, Y.T.; Fu, R.X.; Yu, R.; Zhao, P.P.; Oh, K.C.; Jiang, Z.M.; Hou, J.C. Investigation of the consequences of ultrasound on the physicochemical, emulsification, and gelatinization characteristics of citric acid-treated whey protein isolate. J. Dairy Sci. 2021 , 104 , 10628–10639. [ Google Scholar ] [ CrossRef ]
- Akdeniz, V.; Akalin, A.S. New approach for yoghurt and ice cream production: High-intensity ultrasound. Trends Food Sci. Technol. 2019 , 86 , 392–398. [ Google Scholar ] [ CrossRef ]
- Tomczynska-Mleko, M.; Nishinari, K.; Mleko, S.; Terpilowski, K.; Pérez-Huertas, S. Cold gelation of whey protein isolate with sugars in an ultrasound environment. Food Hydrocoll. 2023 , 139 , 9. [ Google Scholar ] [ CrossRef ]
- Zhao, Y.L.; Yan, M.; Xue, S.Q.; Zhang, T.H.; Shen, X. Influence of ultrasound and enzymatic cross-linking on freeze-thaw stability and release properties of whey protein isolate hydrogel. J. Dairy Sci. 2022 , 105 , 7253–7265. [ Google Scholar ] [ CrossRef ]
- Dupont, D.; Le Feunteun, S.; Marze, S.; Souchon, I. Structuring food to control its disintegration in the gastrointestinal tract and optimize nutrient bioavailability. Innov. Food Sci. Emerg. Technol. 2018 , 46 , 83–90. [ Google Scholar ] [ CrossRef ]
- Cheng, Y.; Yeboah, G.B.; Guo, X.Y.; Donkor, P.O.; Wu, J. Gelling Characteristics of Emulsions Prepared with Modified Whey Protein by Multiple-Frequency Divergent Ultrasound at Different Ultrasonic Power and Frequency Mode. Polymers 2022 , 14 , 2054. [ Google Scholar ] [ CrossRef ]
- Cheng, Y.; Donkor, P.O.; Yeboah, G.B.; Ayim, I.; Wu, J.; Ma, H.L. Modulating the in vitro digestion of heat-set whey protein emulsion gels via gelling properties modification with sequential ultrasound pretreatment. LWT-Food Sci. Technol. 2021 , 149 , 10. [ Google Scholar ] [ CrossRef ]
- Frydenberg, R.P.; Hammershoj, M.; Andersen, U.; Greve, M.T.; Wiking, L. Protein denaturation of whey protein isolates (WPIs) induced by high intensity ultrasound during heat gelation. Food Chem. 2016 , 192 , 415–423. [ Google Scholar ] [ CrossRef ]
- Mao, C.; Wu, J.; Zhang, X.; Ma, F.; Cheng, Y. Improving the solubility and digestibility ofpotato protein with an online ultrasound-assisted pH shifting treatment at medium temperature. Foods 2020 , 9 , 1908. [ Google Scholar ] [ CrossRef ]
- Zhang, H.; Wu, J.; Cheng, Y. Mechanical properties, picrostructure, andin vitro digestion of transglutaminase-crosslinked whey protein and potato protein hydrolysate composite gels. Foods 2023 , 12 , 2040. [ Google Scholar ] [ CrossRef ]
- Gornall, A.G.; Bardawill, C.J.; David, M.M. Determination of serum proteins by means of the biuret reaction. J. Biol. Chem. 1949 , 177 , 751–766. [ Google Scholar ] [ CrossRef ]
- Jiang, J.; Xiong, Y.L. Extreme pH treatments enhance the structure-reinforcement role of soy protein isolate and its emulsions in pork myofibrillar protein gels in the presence of microbial transglutaminase. Meat Sci. 2013 , 93 , 469–476. [ Google Scholar ] [ CrossRef ]
- You, J.; Liu, C.; Zhao, J.; Guo, F.; Wang, Y. pH dominates the formation of ginkgo seed protein and whey protein composite gels. Food Biosci. 2024 , 60 , 104245. [ Google Scholar ] [ CrossRef ]
- Minekus, M.; Alminger, M.; Alvito, P.; Ballance, S.; Bohn, T.; Bourlieu, C.; Carrière, F.; Boutrou, R.; Corredig, M.; Dupont, D.; et al. A standardised static in vitro digestion method suitable for food—An international consensus. Food Funct. 2014 , 5 , 1113–1124. [ Google Scholar ] [ CrossRef ]
- Spellman, D.; McEvoy, E.; O’Cuinn, G.; FitzGerald, R.J. Proteinase and exopeptidase hydrolysis of whey protein: Comparison of the TNBS, OPA and pH stat methods for quantification of degree of hydrolysis. Int. Dairy J. 2003 , 13 , 447–453. [ Google Scholar ] [ CrossRef ]
- Meng, Y.Y.; Liang, Z.Q.; Zhang, C.Y.; Hao, S.Q.; Han, H.Y.; Du, P.; Li, A.L.; Shao, H.; Li, C.; Liu, L.B. Ultrasonic modification of whey protein isolate: Implications for the structural and functional properties. LWT-Food Sci. Technol. 2021 , 152 , 9. [ Google Scholar ] [ CrossRef ]
- Ramírez-Suárez, J.C.; Xiong, Y.L. Effect of transglutaminase-induced cross-linking on gelation of myofibrillar/soy protein mixtures. Meat Sci. 2003 , 65 , 899–907. [ Google Scholar ] [ CrossRef ]
- Cheng, Y.; Donkor, P.O.; Ren, X.F.; Wu, J.; Agyemang, K.; Ayim, I.; Ma, H.L. Effect of ultrasound pretreatment with mono-frequency and simultaneous dual frequency on the mechanical properties and microstructure of whey protein emulsion gels. Food Hydrocoll. 2019 , 89 , 434–442. [ Google Scholar ] [ CrossRef ]
- Rabiey, L.; Britten, M. Effect of protein composition on the rheological properties of acid-induced whey protein gels. Food Hydrocoll. 2009 , 23 , 973–979. [ Google Scholar ] [ CrossRef ]
- de Faria, J.T.; Minim, V.P.R.; Minim, L.A. Evaluating the effect of protein composition on gelation and viscoelastic characteristics of acid-induced whey protein gels. Food Hydrocoll. 2013 , 32 , 64–71. [ Google Scholar ] [ CrossRef ]
- Ma, W.; Wang, J.; Xu, X.; Qin, L.; Wu, C.; Du, M. Ultrasound treatment improved the physicochemical characteristics of cod protein and enhanced the stability of oil-in-water emulsion. Food Res. Int. 2019 , 121 , 247–256. [ Google Scholar ] [ CrossRef ]
- Ahmadi, Z.; Razavi, S.M.A.; Varidi, M. Sequential ultrasound and transglutaminase treatments improve functional, rheological, and textural properties of whey protein concentrate. Innov. Food Sci. Emerg. Technol. 2017 , 43 , 207–215. [ Google Scholar ] [ CrossRef ]
- Kharlamova, A.; Nicolai, T.; Chassenieux, C. Heat-induced gelation of mixtures of casein micelles with whey protein aggregates. Food Hydrocoll. 2019 , 92 , 198–207. [ Google Scholar ] [ CrossRef ]
- Guo, Q.; Ye, A.; Lad, M.; Dalgleish, D.; Singh, H. Effect of gel structure on the gastric digestion of whey protein emulsion gels. Soft Matter 2014 , 10 , 1214–1223. [ Google Scholar ] [ CrossRef ]
- Luo, N.; Ye, A.; Wolber, F.M.; Singh, H. In-mouth breakdown behaviour and sensory perception of emulsion gels containing active or inactive filler particles loaded with capsaicinoids. Food Hydrocoll. 2020 , 108 , 106076. [ Google Scholar ] [ CrossRef ]
- Guo, Q.; Bellissimo, N.; Rousseau, D. Role of gel structure in controlling in vitro intestinal lipid digestion in whey protein emulsion gels. Food Hydrocoll. 2017 , 69 , 264–272. [ Google Scholar ] [ CrossRef ]
Click here to enlarge figure
The statements, opinions and data contained in all publications are solely those of the individual author(s) and contributor(s) and not of MDPI and/or the editor(s). MDPI and/or the editor(s) disclaim responsibility for any injury to people or property resulting from any ideas, methods, instructions or products referred to in the content. |
Share and Cite
Cheng, Y.; Shi, X.; Yeboah, G.B.; Chen, L.; Wu, J. Effect of Multi-Mode Divergent Ultrasound Pretreatment on Hardness, Microstructure and Digestion of Acid-Induced Whey Protein Gels. Foods 2024 , 13 , 1926. https://doi.org/10.3390/foods13121926
Cheng Y, Shi X, Yeboah GB, Chen L, Wu J. Effect of Multi-Mode Divergent Ultrasound Pretreatment on Hardness, Microstructure and Digestion of Acid-Induced Whey Protein Gels. Foods . 2024; 13(12):1926. https://doi.org/10.3390/foods13121926
Cheng, Yu, Xiaolong Shi, Georgina Benewaa Yeboah, Lihong Chen, and Juan Wu. 2024. "Effect of Multi-Mode Divergent Ultrasound Pretreatment on Hardness, Microstructure and Digestion of Acid-Induced Whey Protein Gels" Foods 13, no. 12: 1926. https://doi.org/10.3390/foods13121926
Article Metrics
Article access statistics, further information, mdpi initiatives, follow mdpi.
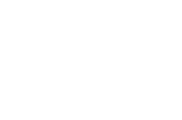
Subscribe to receive issue release notifications and newsletters from MDPI journals
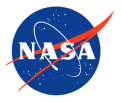
Suggested Searches
- Climate Change
- Expedition 64
- Mars perseverance
- SpaceX Crew-2
- International Space Station
- View All Topics A-Z
Humans in Space
Earth & climate, the solar system, the universe, aeronautics, learning resources, news & events.

NASA Invites Public to Share Excitement of NOAA GOES-U Launch

NASA’s ELaNa 43 Prepares for Firefly Aerospace Launch
Why scientists are intrigued by air in nasa’s mars sample tubes.
- Search All NASA Missions
- A to Z List of Missions
- Upcoming Launches and Landings
- Spaceships and Rockets
- Communicating with Missions
- James Webb Space Telescope
- Hubble Space Telescope
Why Go to Space
- Commercial Space
- Destinations
- Living in Space
- Explore Earth Science
- Earth, Our Planet
- Earth Science in Action
- Earth Multimedia
- Earth Science Researchers
- Pluto & Dwarf Planets
- Asteroids, Comets & Meteors
- The Kuiper Belt
- The Oort Cloud
- Skywatching
- The Search for Life in the Universe
- Black Holes
- The Big Bang
- Dark Energy & Dark Matter
- Earth Science
- Planetary Science
- Astrophysics & Space Science
- The Sun & Heliophysics
- Biological & Physical Sciences
- Lunar Science
- Citizen Science
- Astromaterials
- Aeronautics Research
- Human Space Travel Research
- Science in the Air
- NASA Aircraft
- Flight Innovation
- Supersonic Flight
- Air Traffic Solutions
- Green Aviation Tech
- Drones & You
Technology Transfer & Spinoffs
- Space Travel Technology
- Technology Living in Space
Manufacturing and Materials
- Science Instruments
- For Kids and Students
- For Educators
- For Colleges and Universities
- For Professionals
- Science for Everyone
- Requests for Exhibits, Artifacts, or Speakers
- STEM Engagement at NASA
- NASA's Impacts
- Centers and Facilities
- Directorates
- Organizations
- People of NASA
- Internships
- Our History
- Doing Business with NASA
- Get Involved
- Aeronáutica
- Ciencias Terrestres
- Sistema Solar
- All NASA News
- Video Series on NASA+
- Newsletters
- Social Media
- Media Resources
- Upcoming Launches & Landings
- Virtual Events
- Sounds and Ringtones
- Interactives
- STEM Multimedia
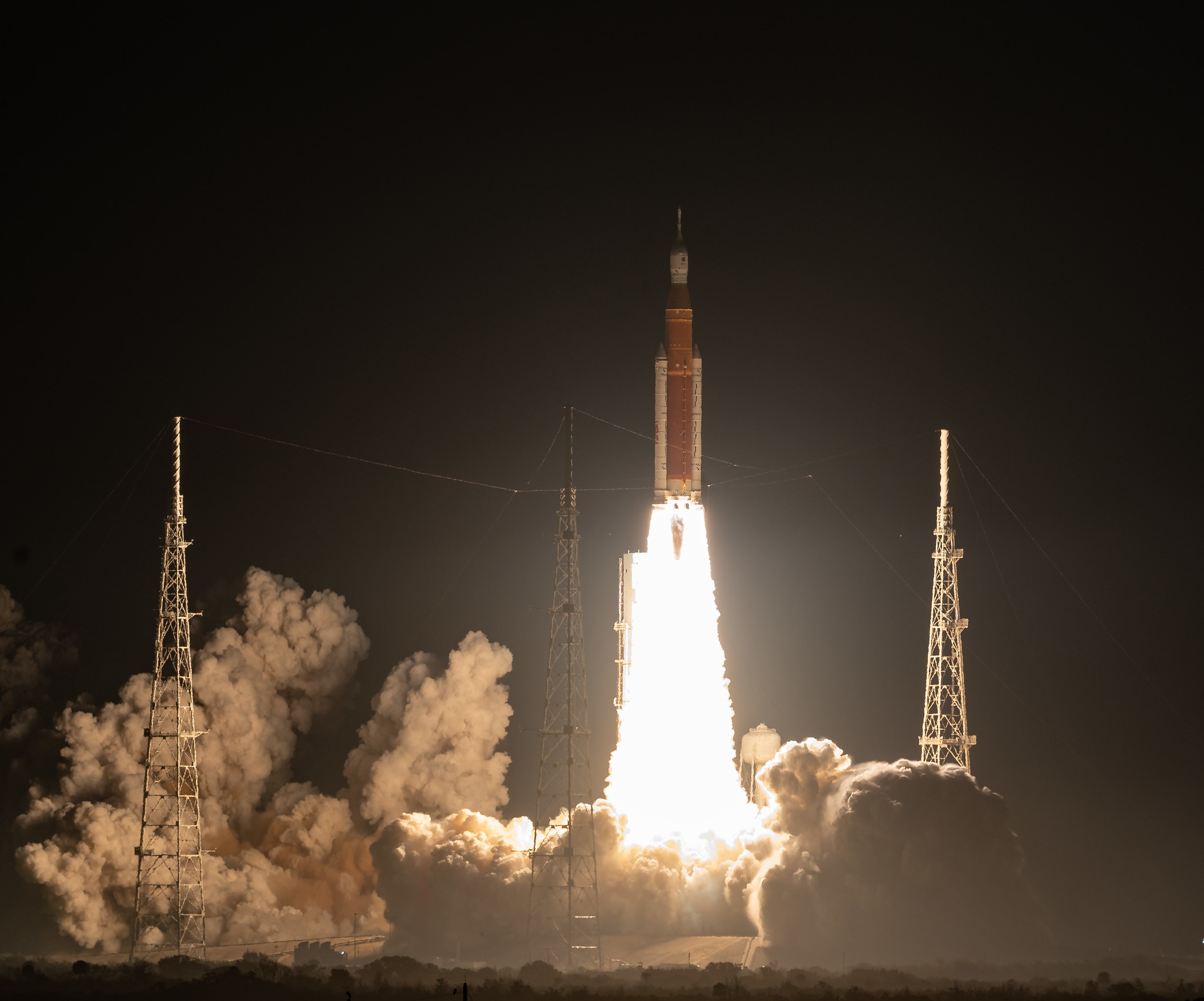
Amendment 22: Heliophysics Flight Opportunities in Research and Technology Final Text and Due Date
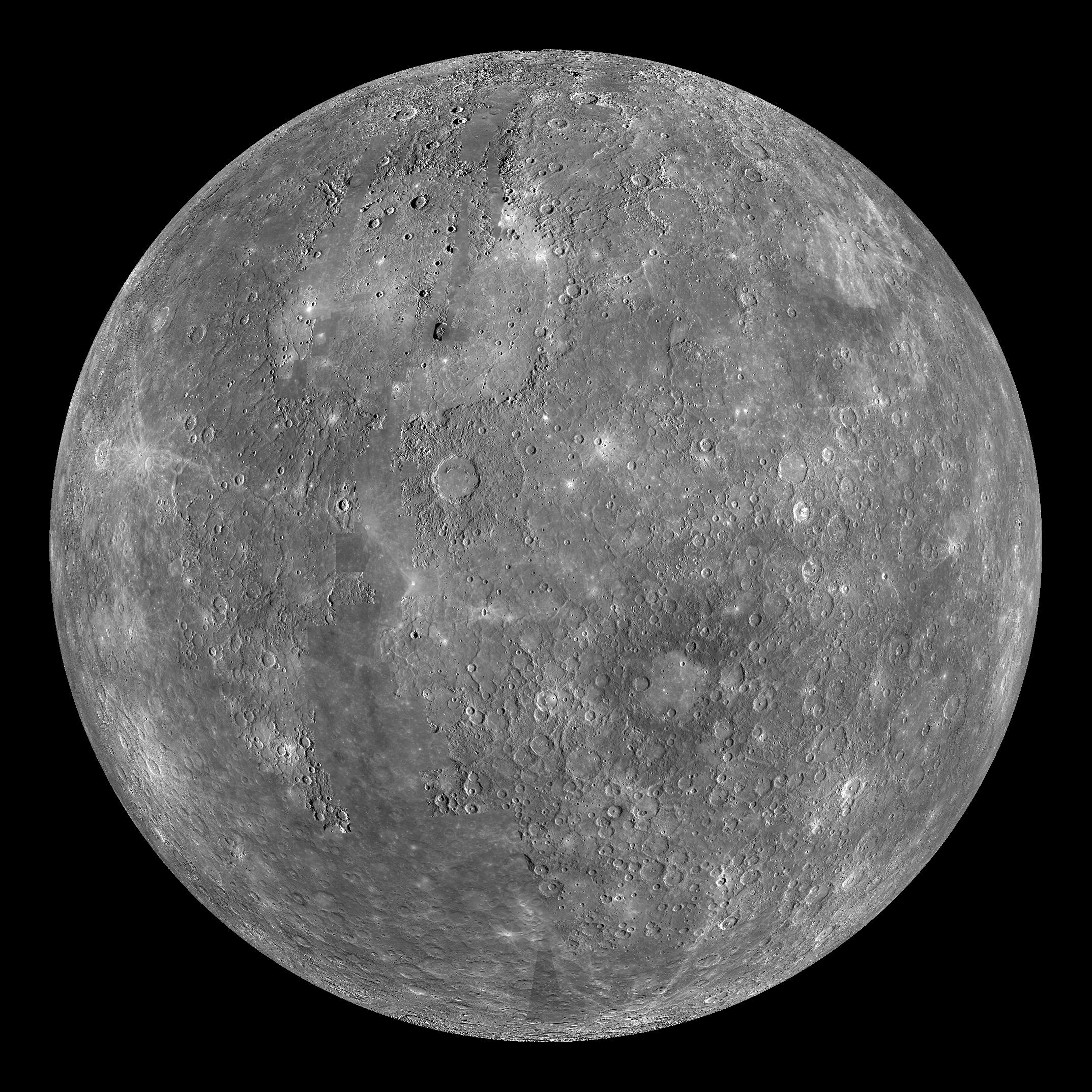
Mercury Resources
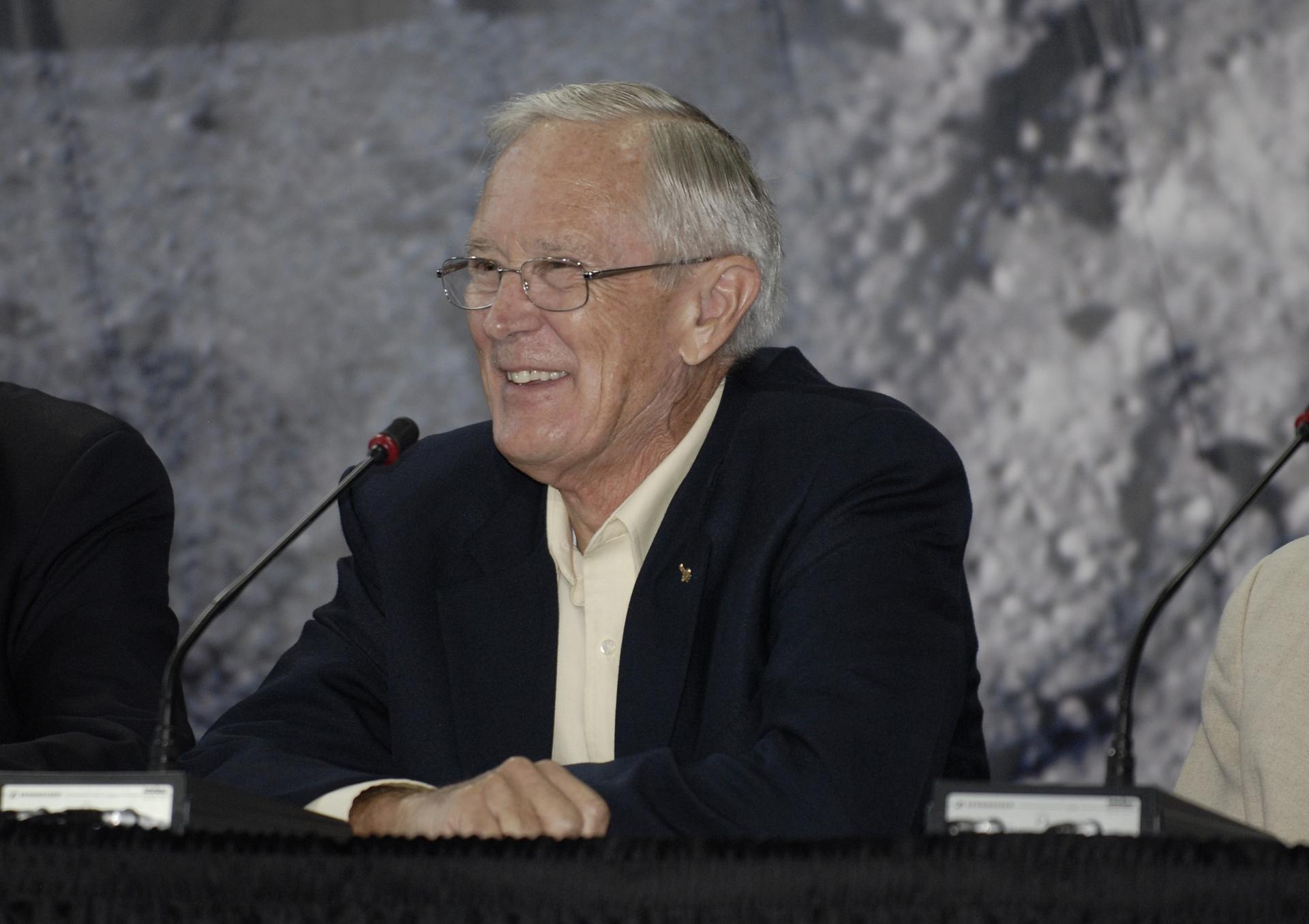
Former Astronaut Charles M. Duke, Jr.
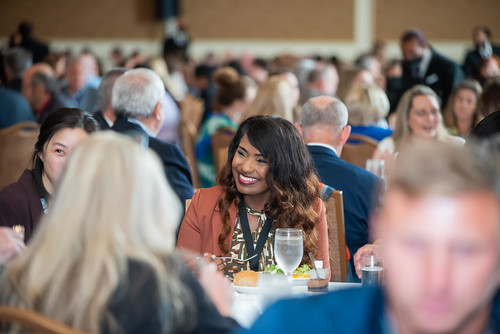
Lakita Lowe: Leading Space Commercialization Innovations and Fostering STEM Engagement
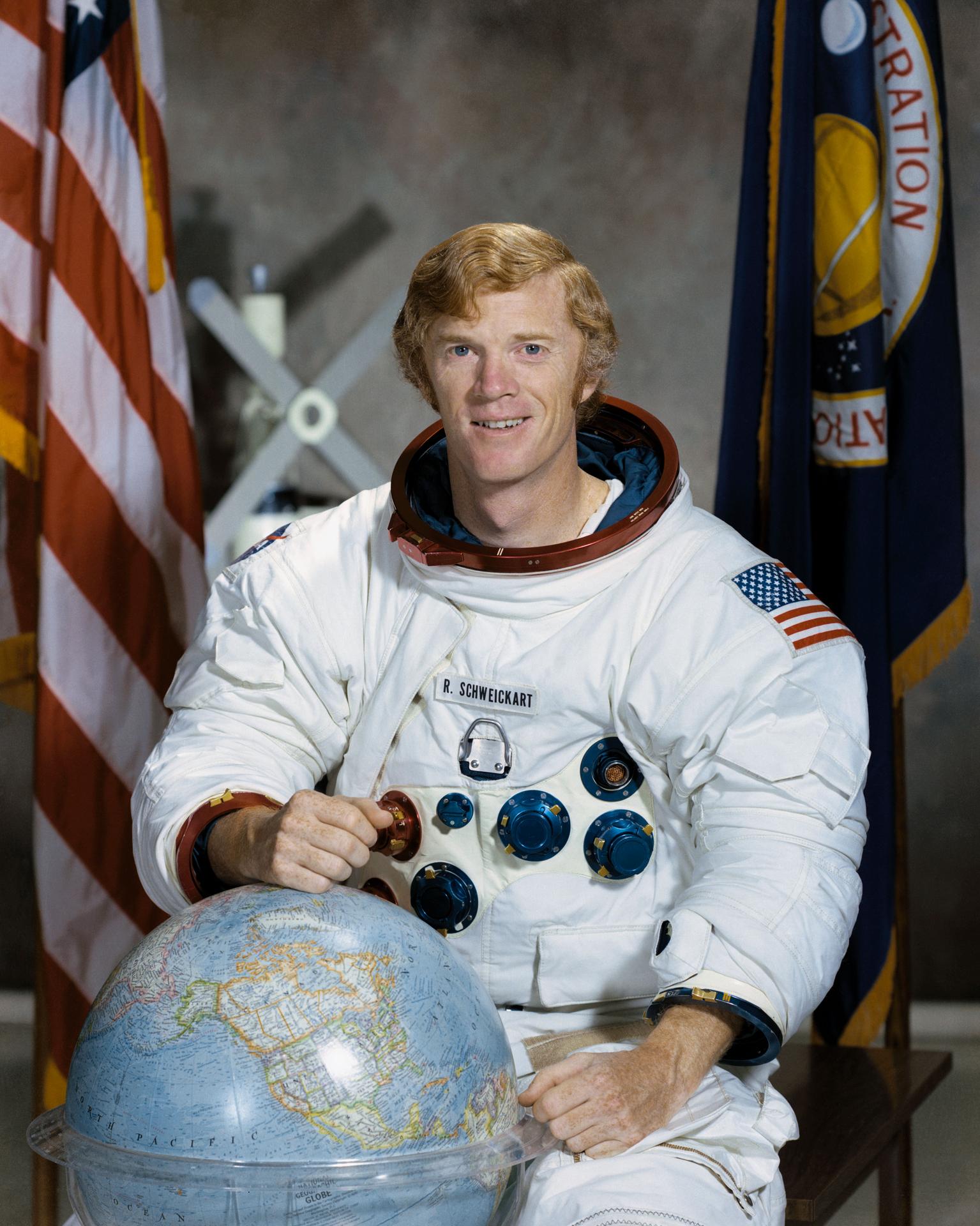
Former Astronaut Russell L. “Rusty” Schweickart
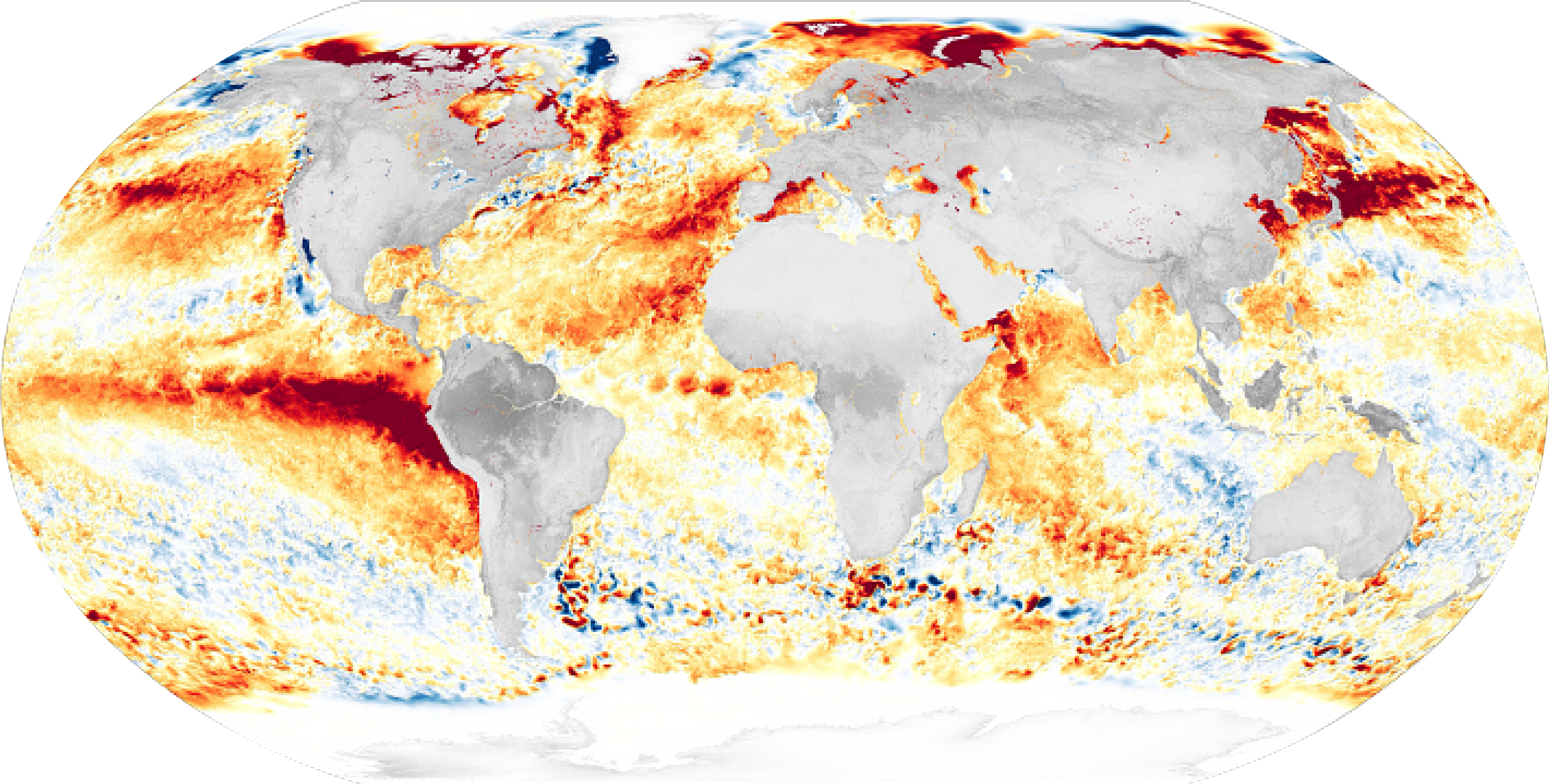
The Ocean and Climate Change
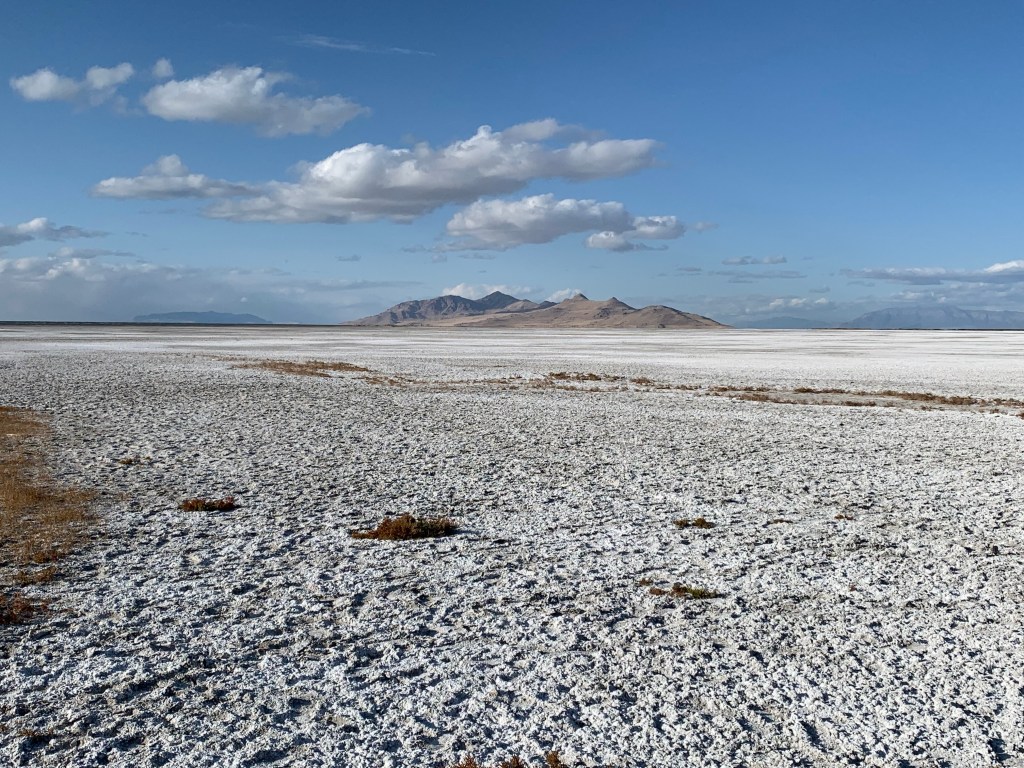
NASA Satellites Find Snow Didn’t Offset Southwest US Groundwater Loss
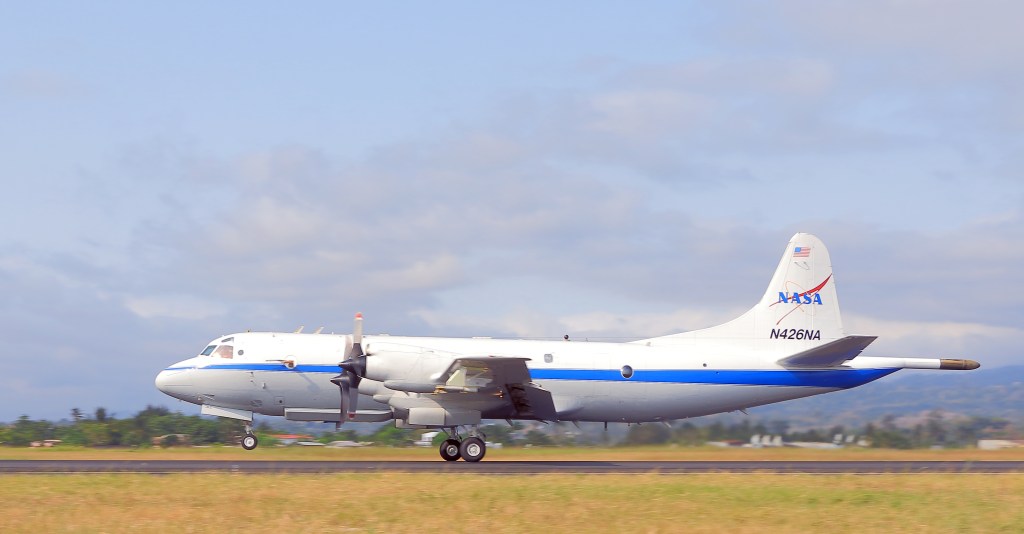
NASA-Led Mission to Map Air Pollution Over Both U.S. Coasts
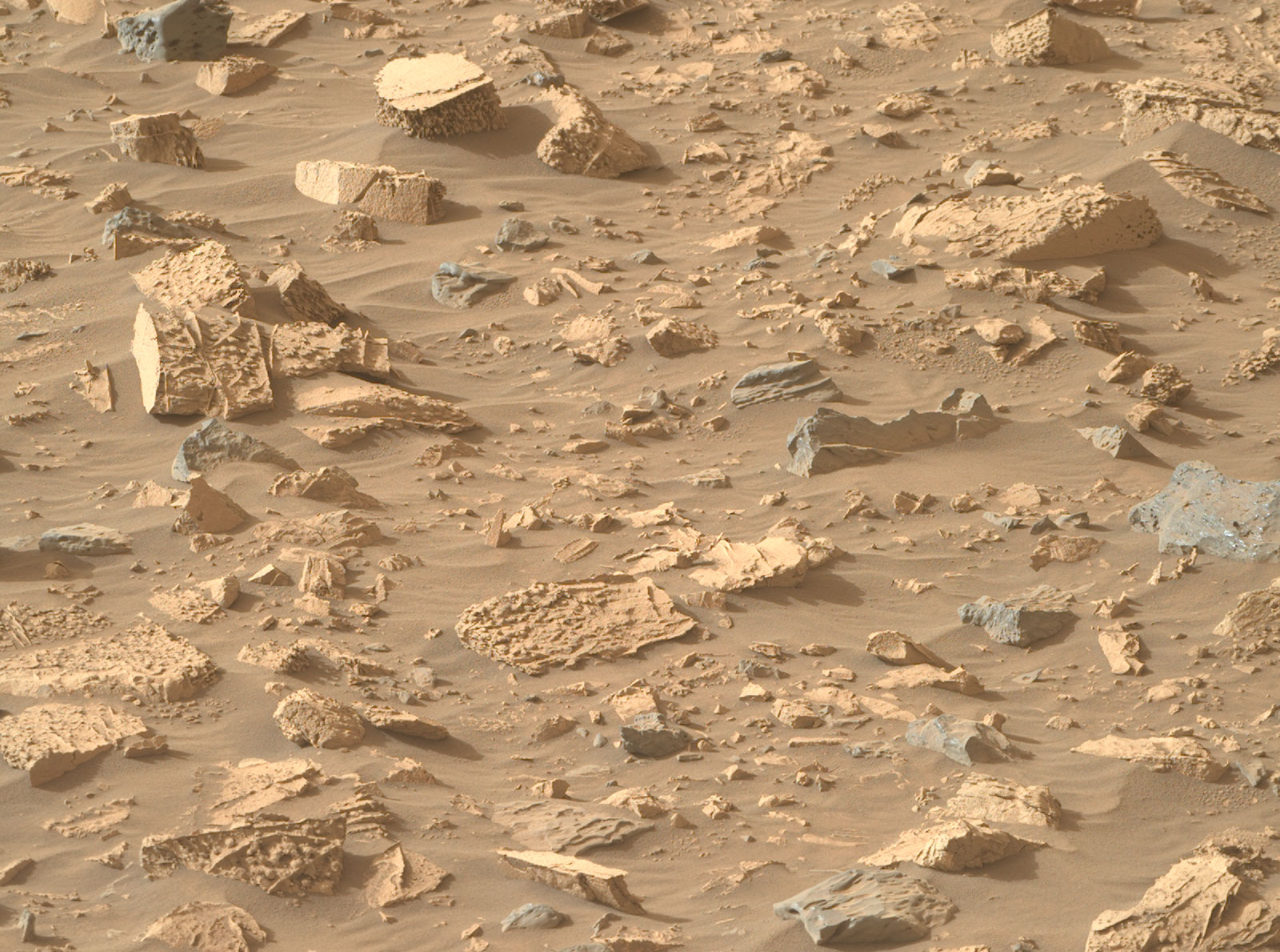
Perseverance Finds Popcorn on Planet Mars
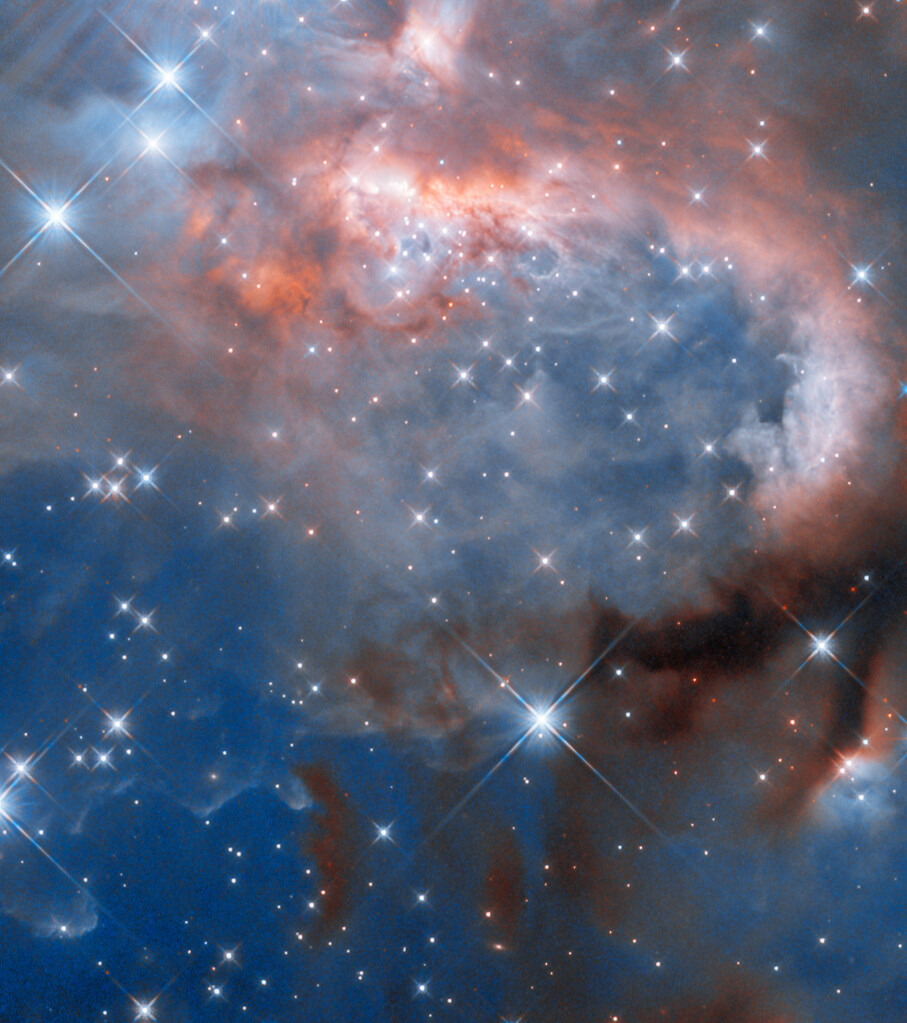
Hubble Captures Infant Stars Transforming a Nebula
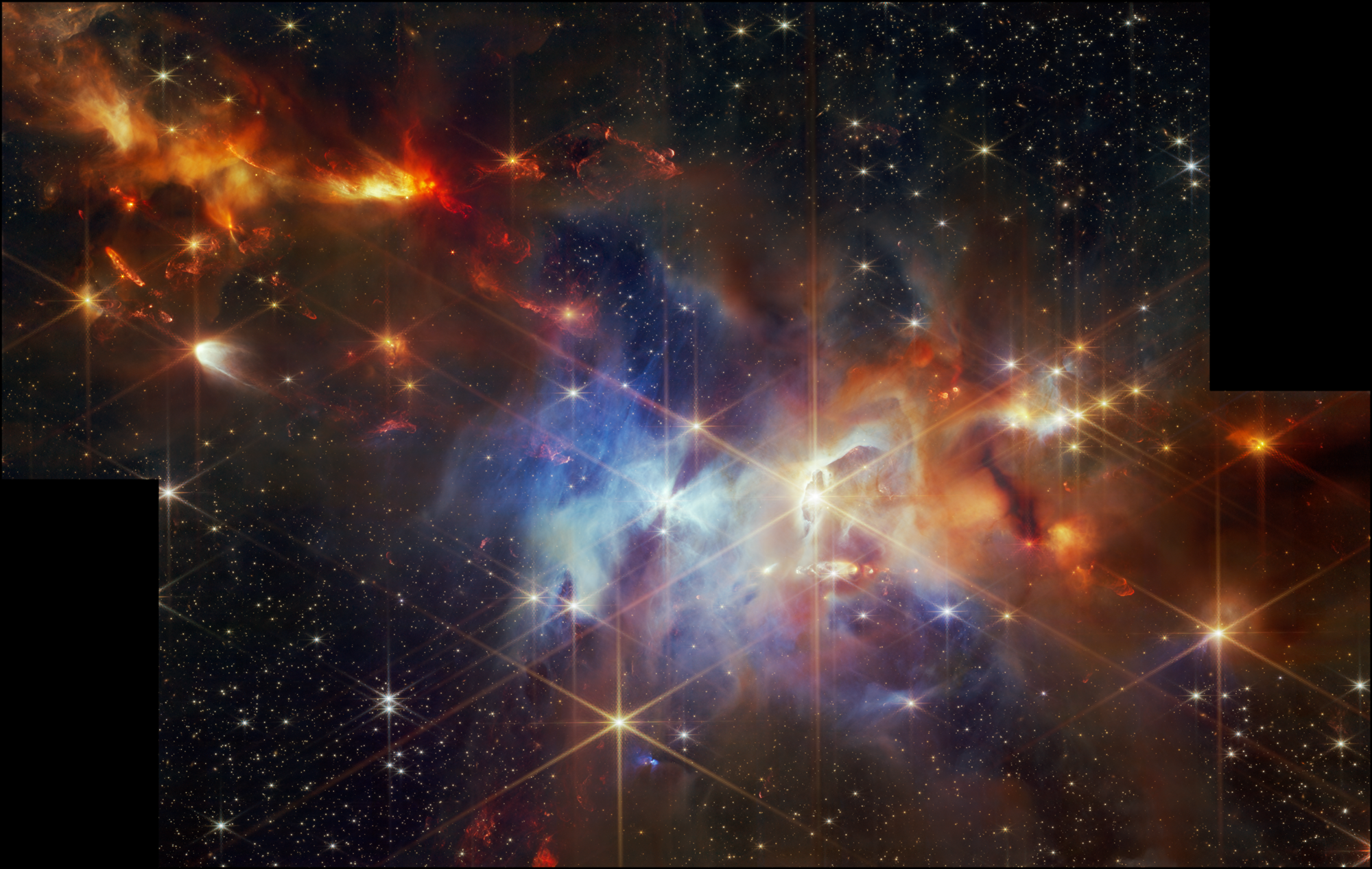
First of Its Kind Detection Made in Striking New Webb Image
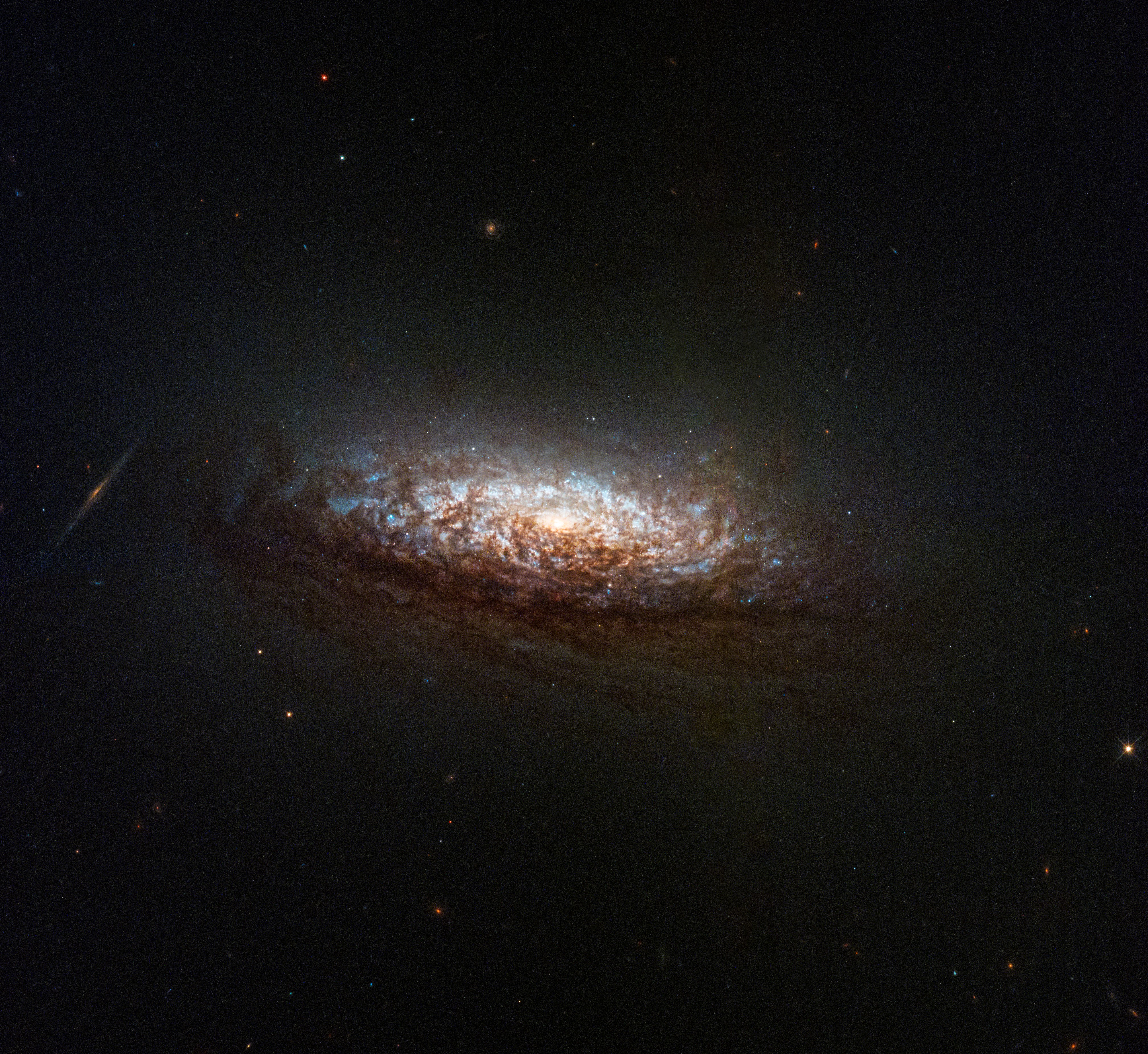
NASA Releases Hubble Image Taken in New Pointing Mode
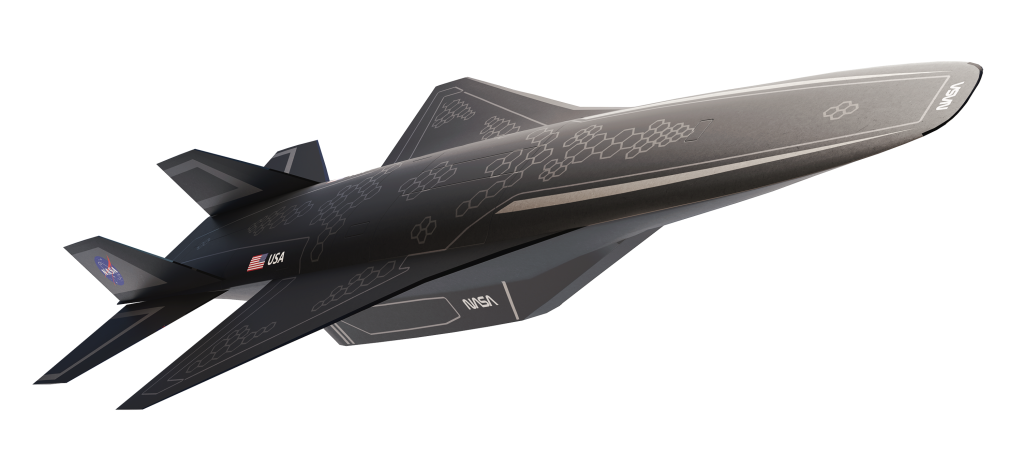
Hypersonic Technology Project
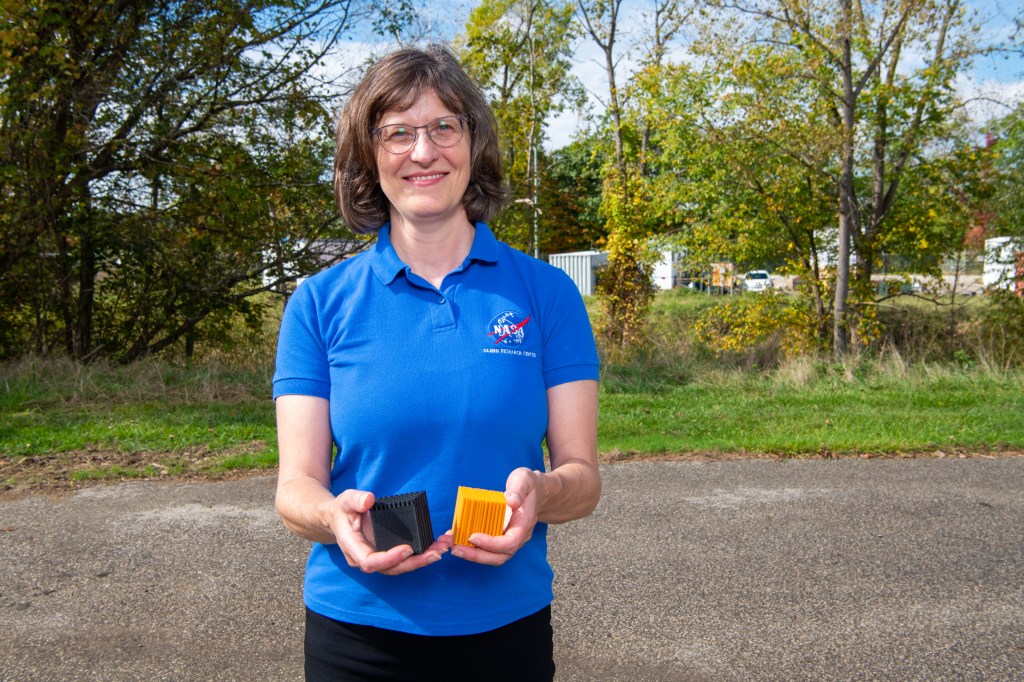
NASA Engineer Honored as Girl Scouts ‘Woman of Distinction’
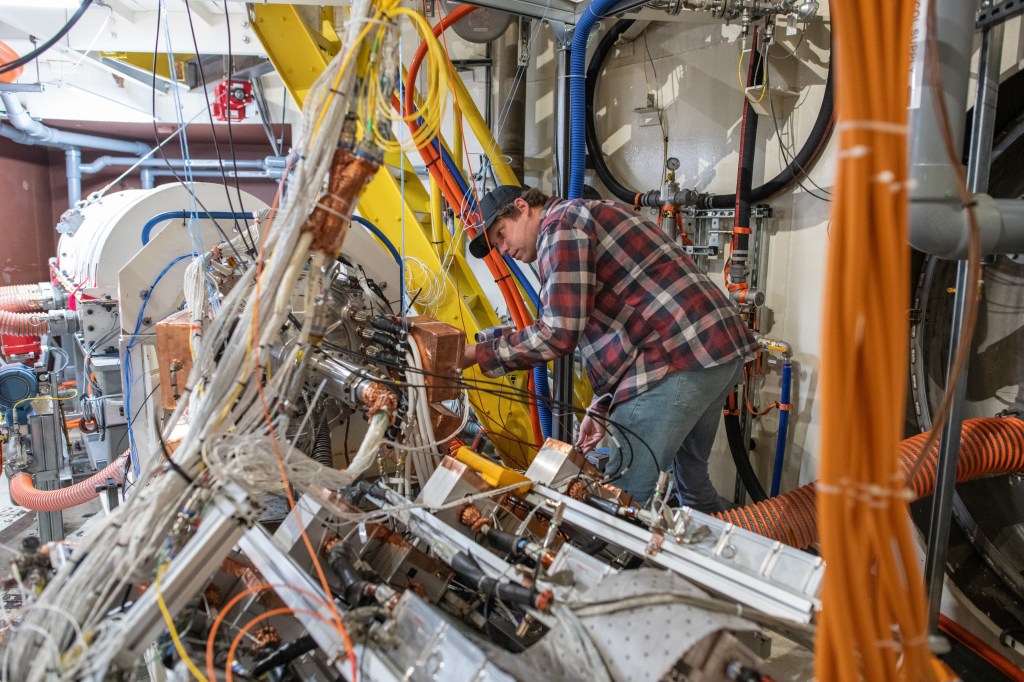
NASA, MagniX Altitude Tests Lay Groundwork for Hybrid Electric Planes

Augmented Reality Speeds Spacecraft Construction at NASA Goddard

Giant Batteries Deliver Renewable Energy When It’s Needed
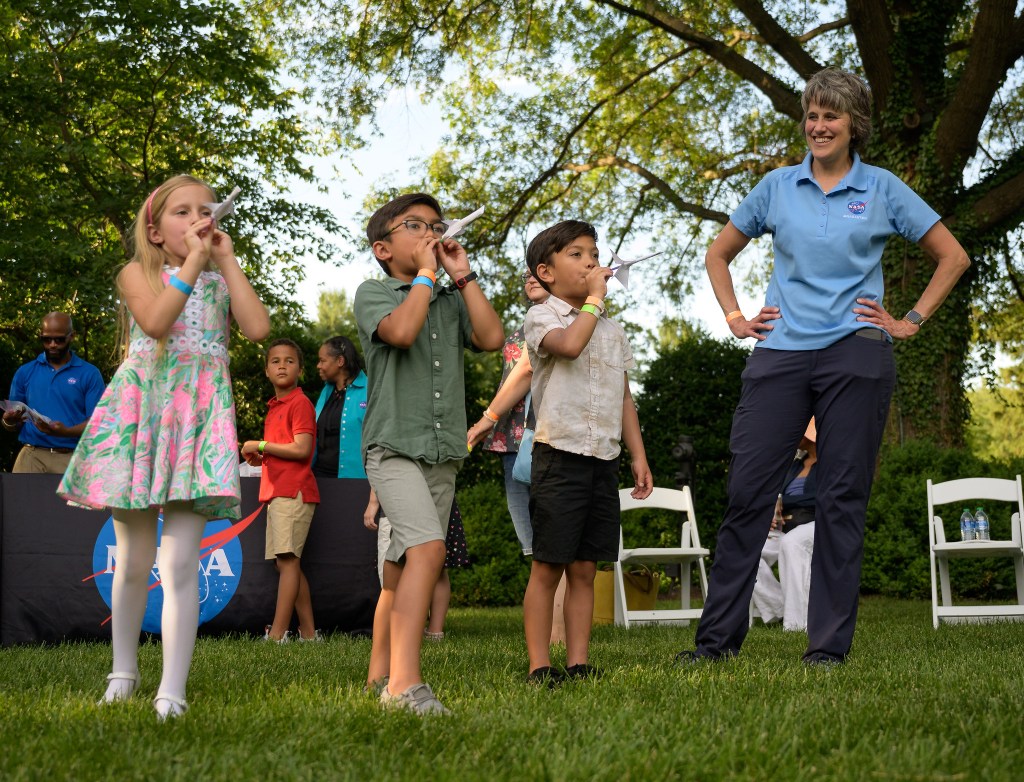
Slow Your Student’s ‘Summer Slide’ and Beat Boredom With NASA STEM
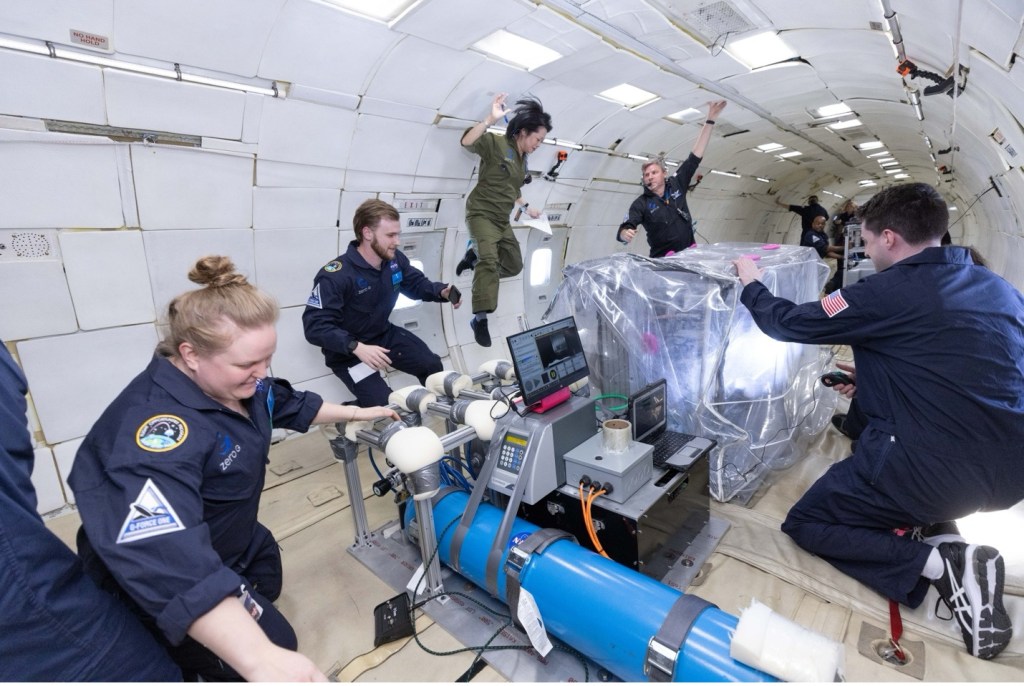
Next Generation NASA Technologies Tested in Flight
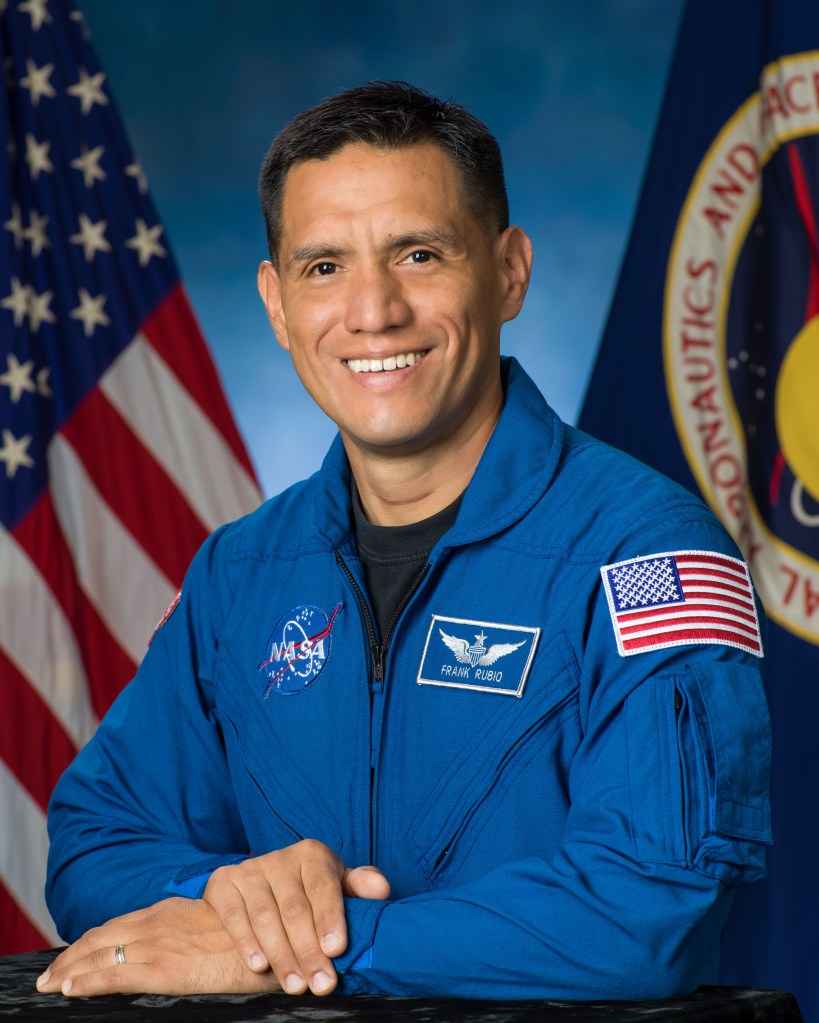
Astronauta de la NASA Frank Rubio
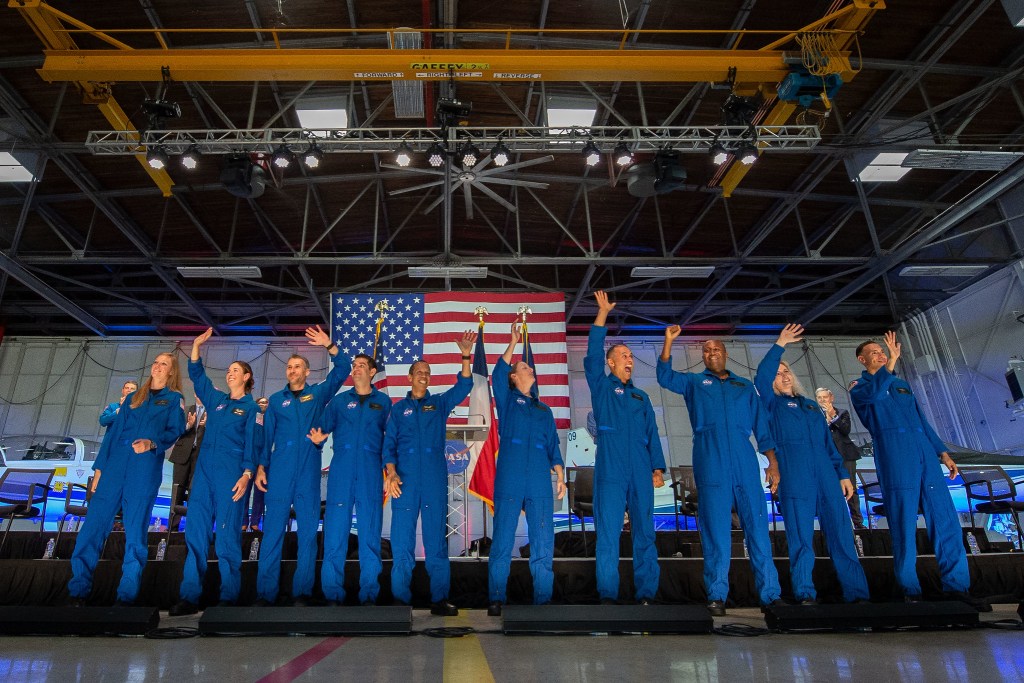
Diez maneras en que los estudiantes pueden prepararse para ser astronautas
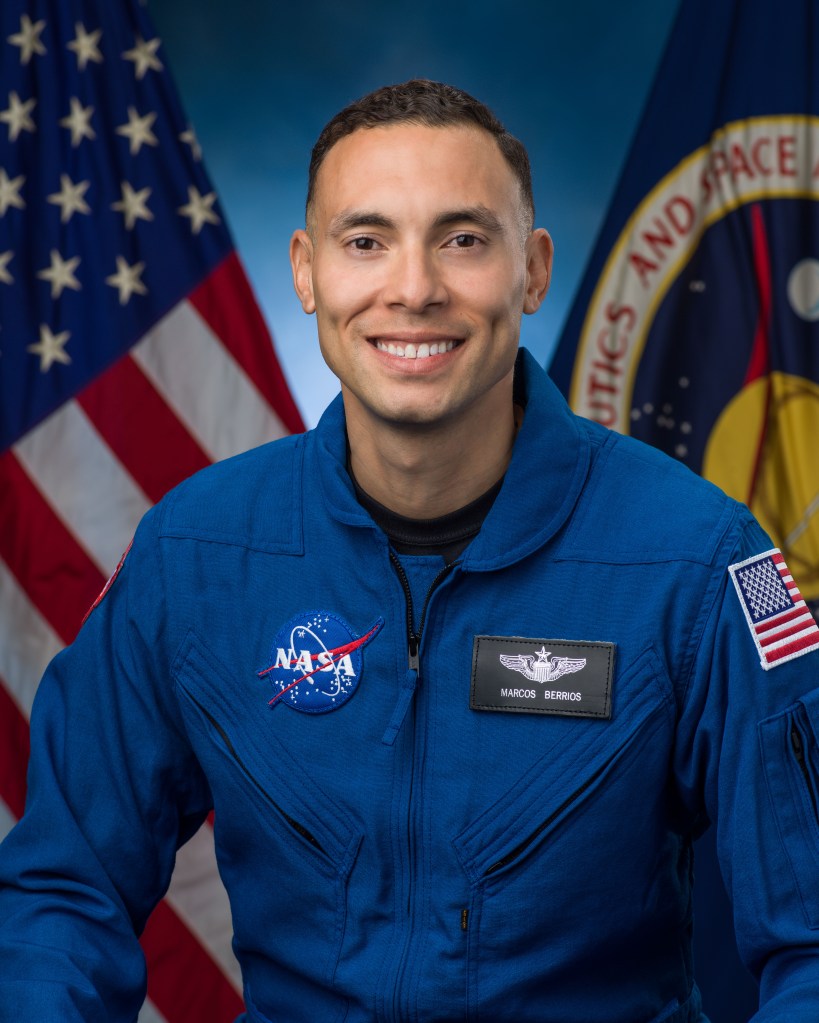
Astronauta de la NASA Marcos Berríos
Hypersonic research topics.

Shannon Eichorn
System-level design and analysis, propulsion technologies, vehicle technologies, high temperature materials.
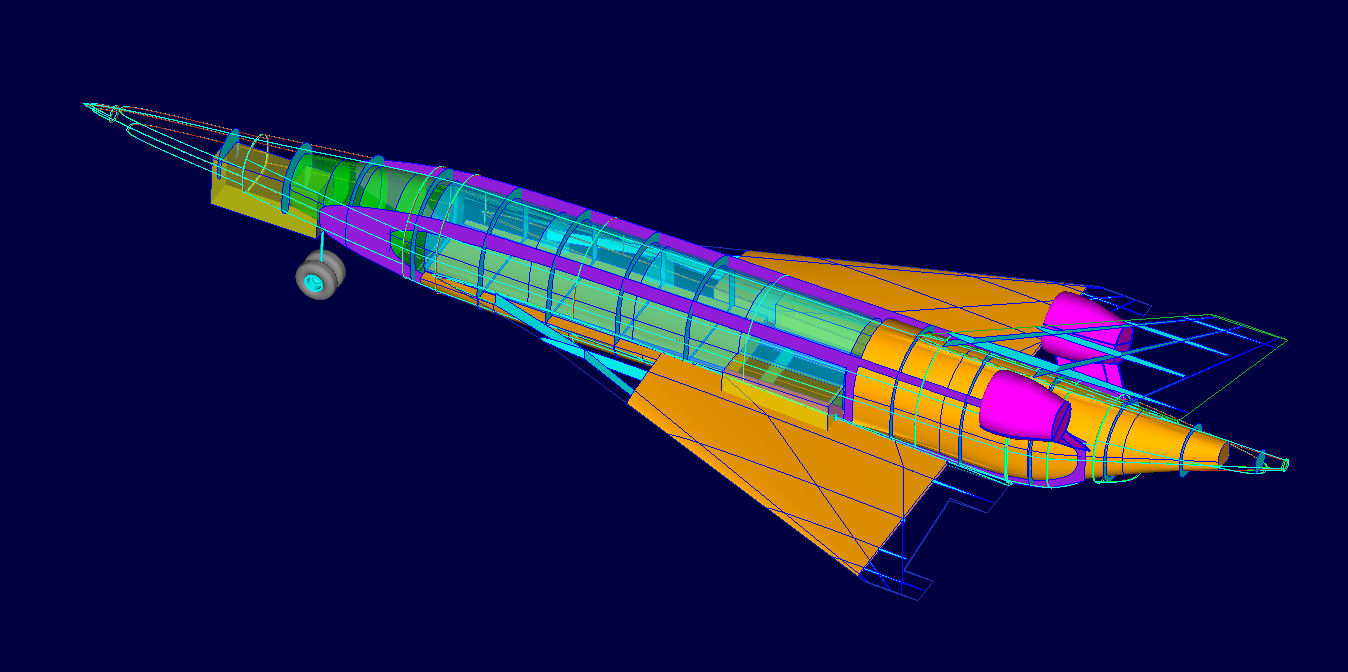
The Hypersonic Technology project is divided into four research topic areas. The first research topic is system-level design, analysis, and validation, which explores the impacts of technologies on vehicle performance. The second and third topics focus more specifically on propulsion technologies and vehicle technologies enabling hypersonic flight. The fourth topic area explores material technology that can survive and be reused in high-temperature hypersonic flight.
The System-Level Design, Analysis, and Validation research topic (RT-1) investments are focused on computational tool development and validation for hypersonic propulsion and vehicle system analysis methods including uncertainty quantification. RT-1 coordinates and performs definitive systems analysis studies to clarify the potential benefits of hypersonic vehicles and technologies for both high-speed civilian travel and space access and will use these studies to drive a technology portfolio focused on reusability, affordability, and reliability.

The Propulsion Technologies research topic (RT-2) focuses on turboramjet, ramjet, integrated combined-cycle, dual-mode, and scramjet propulsion systems and associated propulsive mode transitions, combustor operability, fuels, controls, and sensors. RT-2 develops computational fluid dynamic technologies to enable predictive simulations of these systems.
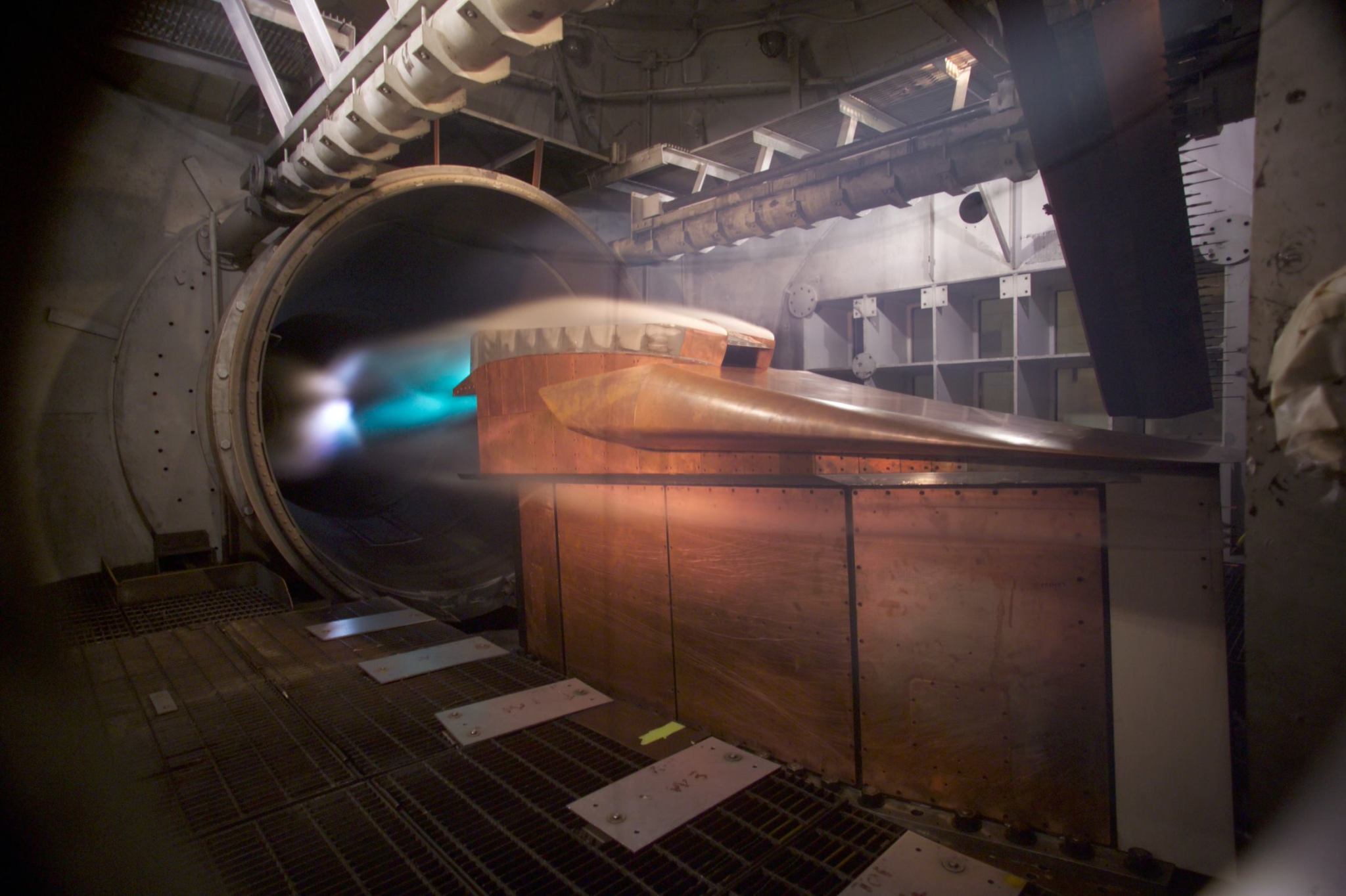
The Vehicle Technologies research topic (RT-3) investments focus on understanding aerodynamic and aerothermodynamic phenomena, such as high-speed boundary-layer transition and shock-dominated flows, to further technologies that improve aerodynamic performance as well as reduce aerodynamic heating.
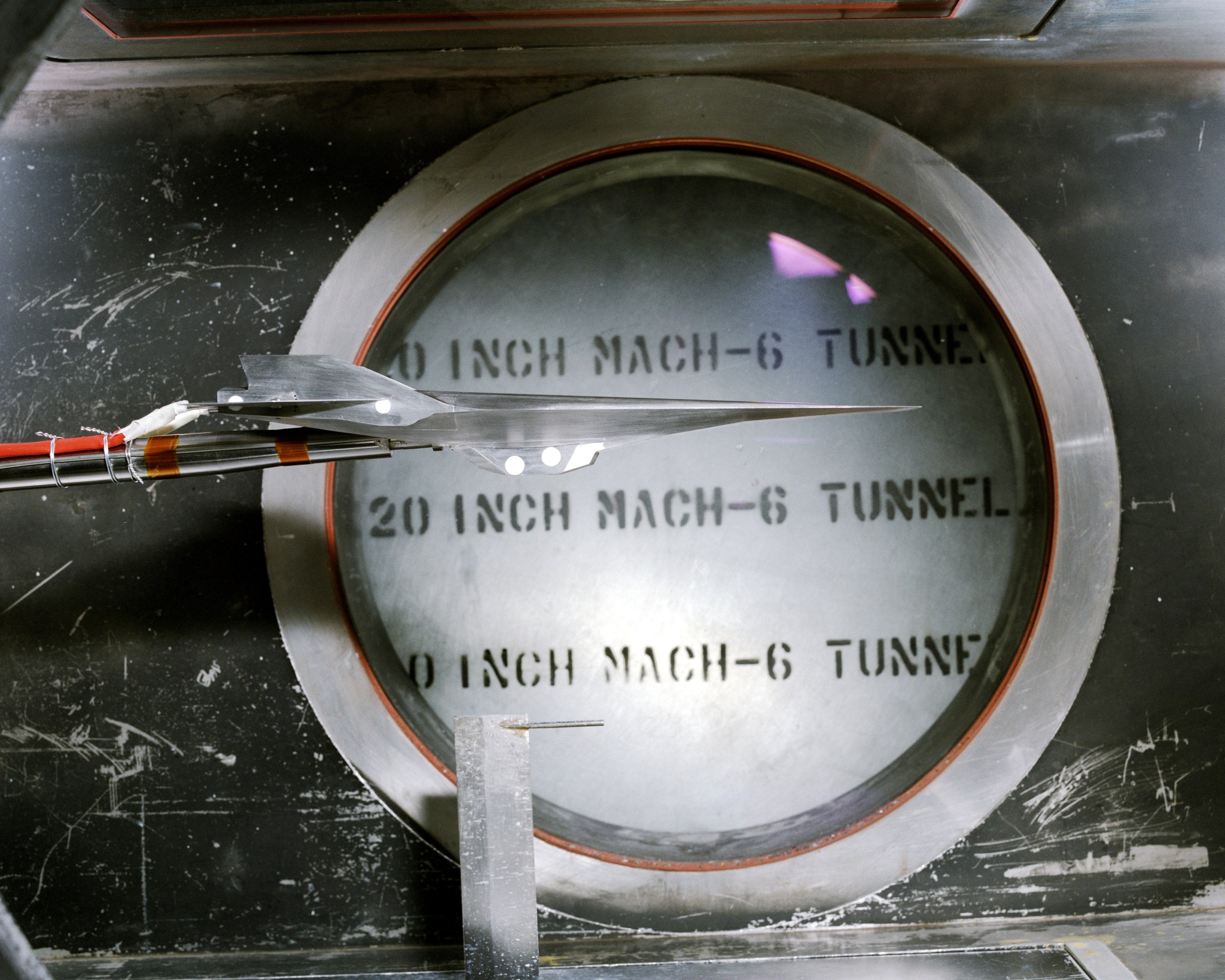
The High Temperature Durable Materials research topic (RT-4) investments focus on advanced propulsion and vehicle materials research. Due to the operating conditions of hypersonic vehicles, most of the structures and materials are shared between propulsion and vehicle components, which include aeroshell, control surface, leading edge, propulsion, and sealing concepts. RT-4 examines the design and evaluation of potential structure and material concepts through component development and testing under relevant environments. In addition, because of the extreme environments the materials and structures must endure, RT-4 also includes development of advanced thermal and structural measurement methods.
About the Author

Shannon Eichorn is the Strategic Engagement Lead for NASA’s Advanced Air Vehicles Program. She is a former test engineer in supersonic wind tunnels and former engineer managing facilities, such as the Aeroacoustic Propulsion Lab, Glenn Extreme Environments Rig, and Creek Road Cryogenics Complex.
Explore More
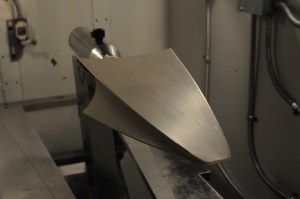
NASA Launches Rocket to Study Hypersonic Aircraft
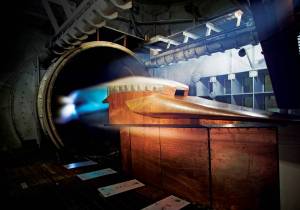
AETC Hypersonic Facilities
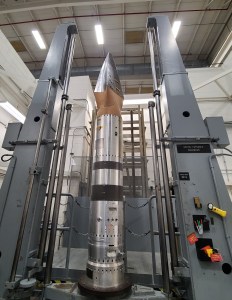
Rocket Launch Scheduled March 21 from NASA’s Wallops Flight Facility
Discover related topics.
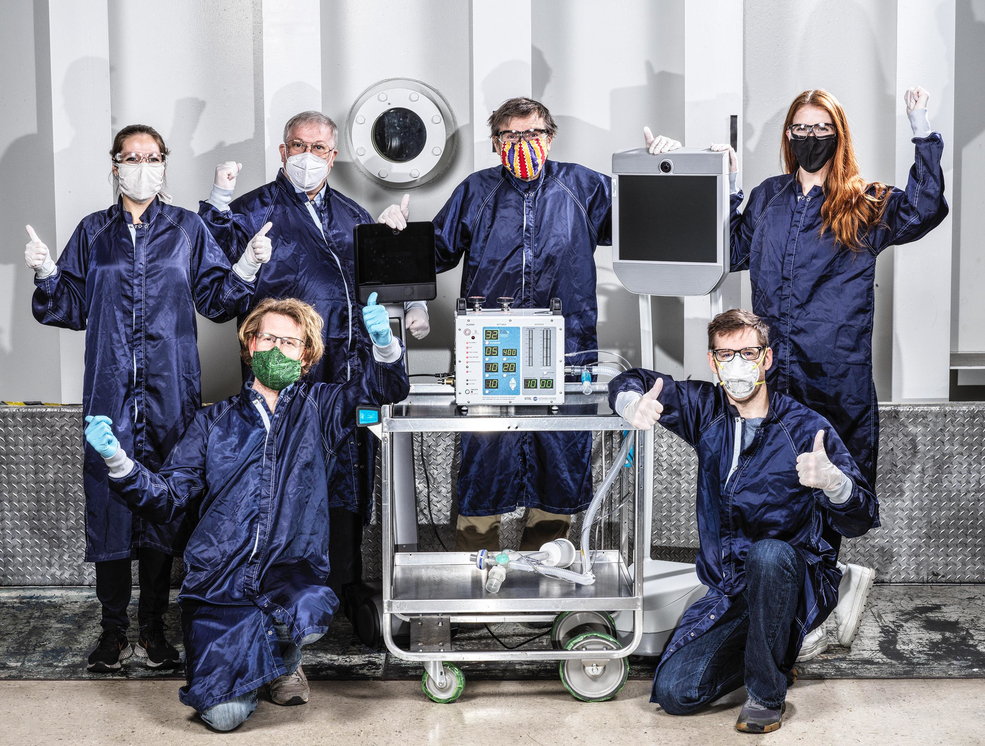
Small Business Innovation Research (SBIR) / Small Business Technology Transfer (STTR)
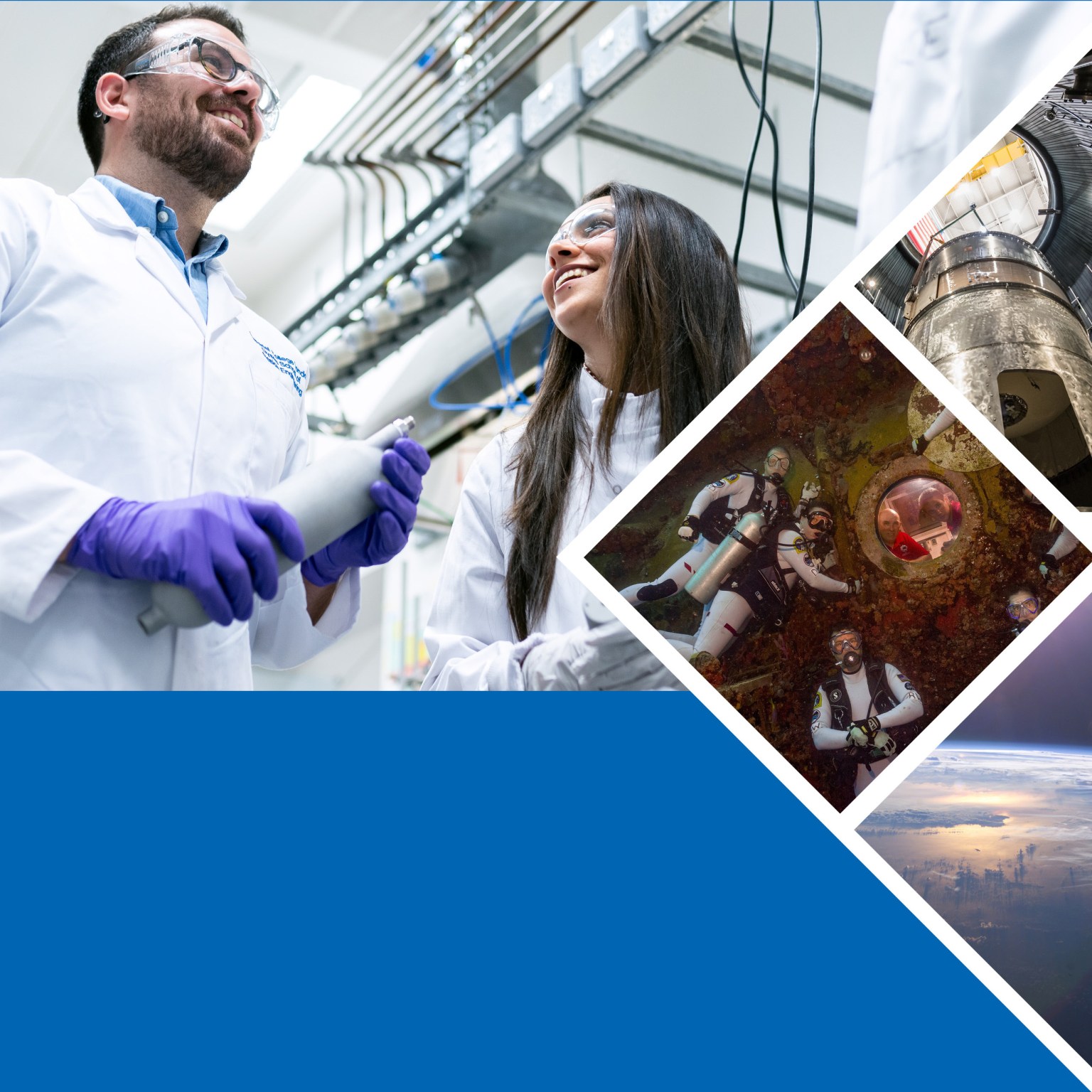
Related Terms
- Hypersonic Technology
- Advanced Air Vehicles Program
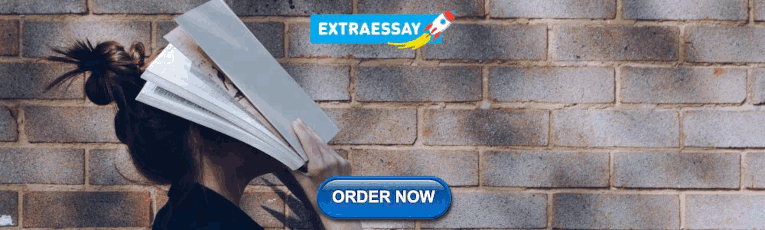
COMMENTS
Relationship Between Ultrasound Viewing and Proceeding to Abortion. Transrectal Ultrasound of the Prostate With a Biopsy. Iron-Based Catalysts Used in Water Treatment Assisted by Ultrasound. 88 Tourism Management Essay Topic Ideas & Examples 64 Virtual Team Essay Topic Ideas & Examples.
Ultrasound articles from across Nature Portfolio. Ultrasound is a non-invasive imaging technique that uses the differential reflectance of acoustic waves at ultrasonic frequencies to detect ...
Correction: Feasibility of using a handheld ultrasound device to detect and characterize shunt and deep vein thrombosis in patients with COVID-19: an observational study. Rajkumar Rajendram, Arif Hussain, Naveed Mahmood and Mubashar Kharal. The Ultrasound Journal 2023 15 :44. Correction Published on: 23 November 2023.
Medical ultrasound represented by ultrasound imaging is generally believed to be well-known area in research, because ultrasound imaging systems has been widely distributed and actively used in the world since the 1960s. ... Hence, this special issue is designed to bring in various ultrasound-related topics in medicine. We also tried to invite ...
Feb. 13, 2023 — Scientists have created a new technology to assemble matter in 3D. Their concept uses multiple acoustic holograms to generate pressure fields with which solid particles, gel ...
The ongoing rapid expansion of point-of-care ultrasound ... This timely paper 10 aims to improve CCE research data reporting, such that clinical providers may more easily use data to support their clinical decision making when caring for critically ill patients. A critical appraisal of the existing literature from 2000-2017 was conducted, and ...
A wearable ultrasonic device to image cardiac function. Researchers have engineered a wearable device that adheres to the skin and uses ultrasound imaging and a deep learning model to produce a ...
The purpose of this editorial update is to provide readers with some recent information and to share my views on the journal and its future directions. During the last 4 years, Ultrasonography has published a total of 160 peer-reviewed articles. In 2017, the Ultrasonography home page had over 85,000 hits per month, with over 2,300 downloads of ...
The Journal of the British Medical Ultrasound Society, Ultrasound is dedicated solely to publishing ultrasound-related topics for users of this rapidly evolving specialty. It provides a forum for presentation of relevant scientific and technical advances in both diagnostic and therapeutic applications of ultrasound. ... Sage Research Methods ...
Keywords: medical ultrasound imaging, therapeutic ultrasound, non-destructive evaluation, nonlinear ultrasonics, physics of ultrasound, underwater ultrasound, ultrasonic transducers . Important Note: All contributions to this Research Topic must be within the scope of the section and journal to which they are submitted, as defined in their mission statements.
Ultrasound Imaging - Current Topics presents complex and current topics in ultrasound imaging in a simplified format. It is easy to read and exemplifies the range of experiences of each contributing author. ... Open Access is an initiative that aims to make scientific research freely available to all. To date our community has made over 100 ...
A thesis or dissertation, as some people would like to call it, is an integral part of the Radiology curriculum, be it MD, DNB, or DMRD. We have tried to aggregate radiology thesis topics from various sources for reference. Not everyone is interested in research, and writing a Radiology thesis can be daunting.
After years of research, an NIH-funded team has developed a wearable cardiac ultrasound imager that can non-invasively capture real-time images of the human heart. The prototype patch, which is about the size of a postage stamp, can be worn during exercise, providing valuable cardiac information when the heart is under stress.
Optimizing current ultrasound technology and a look to the future | Explore the latest full-text research PDFs, articles, conference papers, preprints and more on ULTRASOUND IMAGING. Find methods ...
The Journal for Vascular Ultrasound (JVU) is the official quarterly Journal of the Society for Vascular Ultrasound and publishes peer-reviewed articles on all aspects of the noninvasive diagnosis of vascular disease, including original research, reviews, case reports, letters to the editor, and editorials. Continuing Medical Education (CME) test questions are included with many JVU articles ...
J Ultrasound Med. 2017 Oct; 2017 Jun 6. PMID: 28586152. Emergency physician performed tricuspid annular plane systolic excursion in the evaluation of suspected pulmonary embolism. Daley J, Grotberg J, Pare J, Medoro A, Liu R, Hall MK, Taylor A, Moore CL. Am J Emerg Med. 2017 Jan; 2016 Oct 11.
List of topics. Topics for thesis and projects are given below. Most of the topics can be adjusted to the students qualifications and wishes. Don't hesitate to take contact with the corresponding supervisor - we're looking forward to a discussion with you!
The goals of research in ultrasound usage in space environments are: (1) Determine accuracy of ultrasound in novel clinical conditions. (2) Determine optimal training methodologies, (3) Determine microgravity associated changes and (4) Develop intuitive ultrasound catalog to enhance autonomous medical care.
The researchers also envision that miniature ultrasound transducers, fitted into phones and other portable devices, might be capable of shielding people from the virus. Wierzbicki stresses that there is much more research to be done to confirm whether ultrasound can be an effective treatment and prevention strategy against coronaviruses.
One of the most common uses of ultrasound is during pregnancy, to monitor the growth and development of the fetus, but there are many other uses, including imaging the heart, blood vessels, eyes, thyroid, brain, breast, abdominal organs, skin, and muscles. Ultrasound images are displayed in either 2D, 3D, or 4D (which is 3D in motion).
Topics in emergency abdominal ultrasonography Research. Edited by Luca Brunese and Antonio Pinto. Publication of this suppement has been funded by the University of Molise, Universiy of Siena, University of Cagliari, University of Ferrara and University of Turin. The Supplement Editors declare that they have no competing interests.
In tests in mice, the researchers showed that this ultrasound device, which they call ImPULS (Implantable Piezoelectric Ultrasound Stimulator), can provoke activity in neurons of the hippocampus. Then, they implanted the fibers into the dopamine-producing substantia nigra and showed that they could stimulate neurons in the dorsal striatum to ...
Rui Wang, Katelyn Craft, Elisa Holtzman, Hannah Mason, Christopher Khan, Brett Byram, Jason Mitchell, and Jack H. Noble. "Evaluation of U-Nets for Object Segmentation in Ultrasound Images." Proceedings of SPIE Medical Imaging 2024: Ultrasonic Imaging and Tomography, vol. 12932, 129321G, 2024, San Diego, California, United States. Ultrasound imaging is
Combined with the spatially selective nature of focused ultrasound, ultrasound mediated spatially targeted drug delivery with microbubbles has been a main research topic over last 20 years. As for the application, ultrasound mediated drug delivery through blood brain barrier (BBB) has been most successful in animal model experiments [11, 12 ...
Preclinical ultrasound imaging. Our team characterized vision in NBCe1-knockout animals, laying the foundation for using ultrasound to image and measure the physical properties and function of the kidney, heart and liver. Tools to measure cell and kidney function.
The potential role of ultrasound guiding treat-to-target strategies or tapering treatment in inflammatory arthritis requires further research. However, musculoskeletal ultrasound can also have pitfalls and limitations that a clinician should be aware of. Keywords: Gout; ...
We do so using ultrasound waves, which trigger drug release from circulating nanocarriers when focused on the target," said Matthew G Wilson, a graduate research assistant at the University of ...
Combining full-field digital mammography with ultrasound tomography can improve breast cancer detection and be helpful in imaging dense breasts, according to research published June 18 in Radiology.. A team led by Mary Yamashita, MD, from the University of Southern California in Los Angeles, found that the combined method outperformed full-field digital mammography by itself, including having ...
Whey protein was pretreated with multi-frequency ultrasound in mono-, dual-, and tri-frequency modes. The effect of multi-frequency ultrasound pretreatment on the hardness, chemical forces, and microstructure of acid-induced whey protein gel was investigated. Whey protein gels pretreated with dual- and tri-frequency ultrasound showed higher hardness (p < 0.05) and a denser network than mono ...
The first research topic is system-level design, analysis, and validation, which explores the impacts of technologies on vehicle performance. The second and third topics focus more specifically on propulsion technologies and vehicle technologies enabling hypersonic flight. The fourth topic area explores material technology that can survive and ...