Sustainable Environment Research
Call for papers: upcoming collection, nature-based solutions for climate change adaptation, guest edited by: pierre-antoine versini, amy oen, natalia rodriguez and daniela rizzi.
- Most accessed
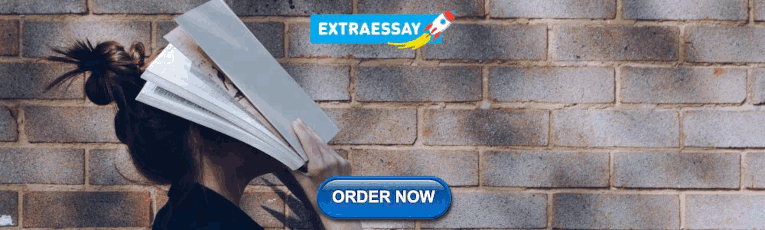
A novel multicriteria assessment framework for evaluating the performance of the EU in dealing with challenges of the low-carbon energy transition: an integrated Fermatean fuzzy approach
Authors: Mahyar Kamali Saraji and Dalia Streimikiene
Improving household water treatment: using zeolite to remove lead, fluoride and arsenic following optimized turbidity reduction in slow sand filtration
Authors: Charles Onyutha, Emmanuel Okello, Rebecca Atukwase, Pamella Nduhukiire, Michael Ecodu and Japheth Nkiriyehe Kwiringira
Enhancing solar still performance with Plexiglas and jute cloth additions: experimental study
Authors: Pankaj Dumka, Dhananjay R. Mishra, Bharat Singh, Rishika Chauhan, Md Irfanul Haque Siddiqui, L Natrayan and Mohd Asif Shah
The Correction to this article has been published in Sustainable Environment Research 2024 34 :5
Multicriteria decision-making tool for investigating the feasibility of the green roof systems in Egypt
Authors: Mahmoud Desouki, Mai Madkour, Ahmed Abdeen and Bahaa Elboshy
Hydrothermal synthesis of zeolites from residual waste generated via indirect carbonation of coal fly ash
Authors: Seonmi Shin and Myoung-Jin Kim
Most recent articles RSS
View all articles
Biological wastewater treatment and bioreactor design: a review
Authors: C. M. Narayanan and Vikas Narayan
A system for monitoring water quality in a large aquatic area using wireless sensor network technology
Authors: Alexander T. Demetillo, Michelle V. Japitana and Evelyn B. Taboada
A comprehensive review on indoor air quality monitoring systems for enhanced public health
Authors: Jagriti Saini, Maitreyee Dutta and Gonçalo Marques
Study of the feasibility of a rice husk recycling scheme in Japan to produce silica fertilizer for rice plants
Authors: Ryoko Sekifuji and Masafumi Tateda
Near infrared band of Landsat 8 as water index: a case study around Cordova and Lapu-Lapu City, Cebu, Philippines
Authors: Jeremy P. Mondejar and Alejandro F. Tongco
Most accessed articles RSS
Journal Announcement
2020-2022 Editor's Choice Articles
Archival Content
The archival content of Sustainable Environment Research can be located here .
Aims and scope
The primary goal of Sustainable Environment Research (SER) is to publish high quality research articles associated with sustainable environmental science and technology and to contribute to improving environmental practice. The scope of SER includes issues of environmental science, technology, management and related fields, especially in response to sustainable water, energy and other natural resources. Potential topics include, but are not limited to:
1. Water and Wastewater
• Biological processes • Physical and chemical processes • Watershed management • Advanced and innovative treatment
2. Soil and Groundwater Pollution
• Contaminant fate and transport processes • Contaminant site investigation technology • Soil and groundwater remediation technology • Risk assessment in contaminant sites
3. Air Pollution and Climate Change
• Ambient air quality management • Greenhouse gases control • Gaseous and particulate pollution control • Indoor air quality management and control
4. Waste Management
• Waste reduction and minimization • Resource recovery and conservation • Solid waste treatment technology and disposal
5. Energy and Resources
• Sustainable energy • Local, regional and global sustainability • Environmental management system • Life-cycle assessment • Environmental policy instruments • Techno-economic assessment
- Editorial Board
- Instructions for Editors
- Sign up for article alerts and news from this journal
- Follow SER on Linkedin
Affiliated with
Sustainable Environment Research is affiliated with the Chinese Institute of Environmental Engineering
Annual Journal Metrics
2022 Citation Impact 4.9 - 2-year Impact Factor 6.4 - 5-year Impact Factor 1.899 - SNIP (Source Normalized Impact per Paper) 0.865 - SJR (SCImago Journal Rank)
2023 Speed 23 days submission to first editorial decision for all manuscripts (Median) 147 days submission to accept (Median)
2023 Usage 354,574 downloads 25 Altmetric mentions
ISSN: 2468-2039
- Submission enquiries: Access here and click Contact Us
- General enquiries: [email protected]
- Browse All Articles
- Newsletter Sign-Up
EnvironmentalSustainability →
No results found in working knowledge.
- Were any results found in one of the other content buckets on the left?
- Try removing some search filters.
- Use different search filters.
Thank you for visiting nature.com. You are using a browser version with limited support for CSS. To obtain the best experience, we recommend you use a more up to date browser (or turn off compatibility mode in Internet Explorer). In the meantime, to ensure continued support, we are displaying the site without styles and JavaScript.
- View all journals
Sustainability articles from across Nature Portfolio
Sustainability is essentially the ability to endure, for example by exploiting resources in a way that does not deplete their future availability or unduly damage the wider environment. This concept has been incorporated in the idea of sustainable development.
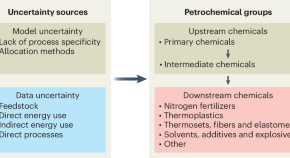
Mitigating uncertainties enables more accurate greenhouse gas accounting for petrochemicals
The carbon footprints of petrochemicals have large uncertainties, challenging decarbonization efforts. Now, a study identifies the main uncertainty sources and strategies for improving the accuracy of greenhouse gas emissions estimations and reporting for petrochemicals.
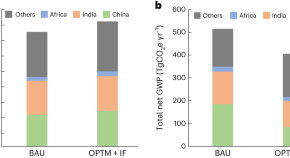
Optimizing organic residue management to improve rice yield and reduce carbon emissions
Returning agricultural organic residues to the soil is imperative for food security and carbon neutrality. We scaled up field findings using machine learning and found that the co-benefits of improved rice yield and reduced net carbon emissions can be realized with integrated management of organic residues and water worldwide.
Latest Research and Reviews
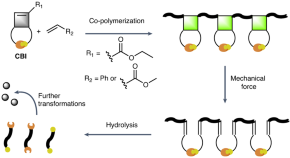
Mechanically triggered on-demand degradation of polymers synthesized by radical polymerizations
Radical polymerizations yield polymers that cannot easily be degraded. The co-polymerization of cyclobutene-based monomers with conventional vinyl monomers has now been shown to result in co-polymers with cyclobutane mechanophores in their backbone, which facilitate on-demand degradation through a combination of mechanical activation and hydrolysis. This approach offers a promising avenue for the degradation of all-carbon-bond-backbone polymers.
- Sètuhn Jimaja
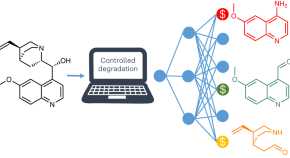
Computational synthesis design for controlled degradation and revalorization
The controlled degradation of larger and potentially harmful molecules into smaller, and preferably valuable, products is a crucial step to close the waste–degradation–synthesis loop envisioned by circular chemistry. Now, a forward-synthesis algorithm is designed to facilitate such degradation-oriented analyses, and proof-of-concept experimental validation is provided.
- Anna Żądło-Dobrowolska
- Karol Molga
- Bartosz A. Grzybowski
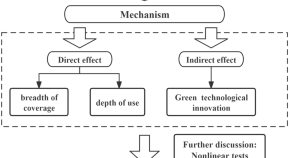
Digital inclusive finance, green technological innovation, and carbon emissions from a spatial perspective
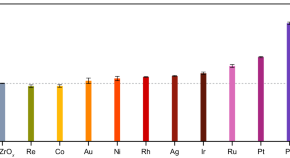
Low-nuclearity CuZn ensembles on ZnZrO x catalyze methanol synthesis from CO 2
The ideal metal selection and atomic-level arrangement for catalysts in CO 2 hydrogenation are still uncertain. Here, copper is identified as the most effective promoter for enhancing ZnZrO x catalysts when precisely structured into CuZn ensembles, offering new insights for designing superior catalysts for CO 2 -based methanol synthesis.
- Thaylan Pinheiro Araújo
- Georgios Giannakakis
- Javier Pérez-Ramírez
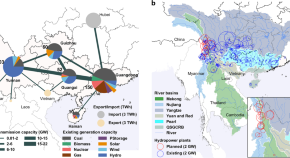
China Southern Power Grid’s decarbonization likely to impact cropland and transboundary rivers
Decarbonization of the Southern Power Grid in China is feasible by 2060 but requires converting a large cropland area to support solar and wind energy; expansion of hydropower will impact the transboundary rivers according to a power system optimization model set up for 2020–2060.
- A.F.M. Kamal Chowdhury
- Stefano Galelli
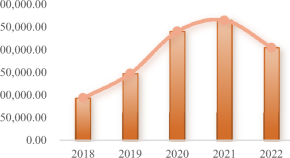
The impact of environmental regulation on green investment efficiency of thermal power enterprises in China-based on a three-stage exogenous variable model
- Fang-rong Ren
- Tao-feng Wu
- Xiaomei Yuan
News and Comment
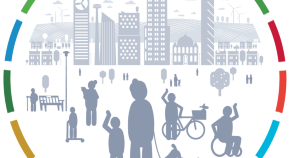
Transformative localization to accelerate the 2030 Agenda
To get the Sustainable Development Goals (SDGs) back on track we need to reshape our approaches to implementation, including localization. Localization done differently involves progressing beyond symbolic piecemeal efforts, prioritizing the SDGs with the greatest gains, and pluralizing interpretations and pathways for actions.
- Shirin Malekpour
- Brett Bryan
Don’t dismiss carbon credits that aim to avoid future emissions
- Edward Mitchard
- Peter Ellis
- Roselyn Fosuah Adjei
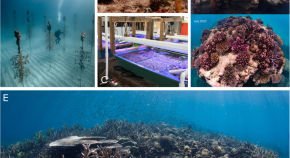
Restoration as a meaningful aid to ecological recovery of coral reefs
Restoration supports the recovery of ecological attributes such as cover, complexity, and diversity to slow the areal decline of natural ecosystems. Restoration activity is intensifying worldwide to combat persistent stressors that are driving global declines to the extent and resilience of coral reefs. However, restoration is disputed as a meaningful aid to reef ecological recovery, often as an expensive distraction to addressing the root causes of reef loss. We contend this dispute partly stems from inferences drawn from small-scale experimental restoration outcomes amplified by misconceptions around cost-based reasoning. Alongside aggressive emissions reductions, we advocate urgent investment in coral reef ecosystem restoration as part of the management toolbox to combat the destruction of reefs as we know them within decades.
- David J. Suggett
- James Guest
Don’t underestimate the rising threat of groundwater to coastal cities
- Daniel J. Rozell
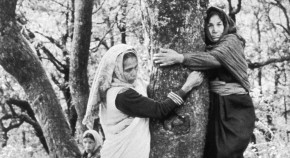
How a tree-hugging protest transformed Indian environmentalism
Fifty years ago, a group of women from the villages of the Western Himalayas sparked Chipko, a green movement that remains relevant in the age of climate change.
- Seema Mundoli
Quick links
- Explore articles by subject
- Guide to authors
- Editorial policies

Research Areas
Learn more about the facets of research in sustainability and how you can contribute to advancing a sustainable future while at Hopkins.
Boundless Potential
The Johns Hopkins community is frequently recognized for groundbreaking research, and every day here is an opportunity for discovery. S ustainability-focused research efforts have expanded significantly in the last several years , including the establishment of the Ralph O’Connor Sustainable Energy Institute and the announcement of two new Bloomberg Distinguished Professor clusters in Climate, Resilience, and Health and Sustainable Transformations and Energy .
Read more about the diverse areas of research in sustainability to discover where your interests lie:
Affordable & Sustainable Energy
The future of sustainable energy is integral to society’s ability to combat the climate crisis. It is imperative that we develop and implement energy infrastructure and systems that make renewable, efficient energy widely accessible. Faculty, staff, and students alike are exploring the technology, policy, and infrastructure that would allow for the widespread adoption of just and sustainable energy alternatives.
Explore JHU Affordable & Sustainable Energy Researchers here .
Clean Water & Sanitation
Water covers over 70% of the Earth’s surface and is a defining feature of our planet. Water availability and access to clean and safe drinking water are essential for human health and sustainable development beyond contributions to the atmospheric processes that allow for life on our planet. Engineering, risk assessment, epidemiology, and agriculture are samples of the subject areas impacted by water and are areas where Hopkins faculty are working to address global water challenges.
Explore JHU Clean Water & Sanitation Researchers here .
Climate Solutions
Climate change poses one of the most — if not the most — pressing threats to this and future generations. It is a dire problem that demands immediate, collective action not just from climate scientists and policymakers, but from individuals across all disciplines. Johns Hopkins is dedicated to leveraging its world-class faculty, resources, and influence to develop and implement solutions to the climate problem.
Explore JHU Climate Solutions Researchers here .
Healthy Communities, Workplaces & Lifestyles
The health and well-being of communities is dependent on a multitude of factors. Environmental sustainability touches many: community food systems, green space & urban tree canopy, and access to clean, drinkable water among them. Sometimes oversimplified to an issue of choice, the health status and lifestyle choices of community members are often a byproduct of the systems — political, economic, social — in which they exist. Many JHU affiliates study and implement methods to increase agency and accessibility to resources within communities across impact areas.
Explore JHU Healthy Communities, Workplaces & Lifestyles Researchers here .
Healthy Air
One of the costs of industrialization, clean and healthy air is not a guarantee and minority, impoverished, and disenfranchised communities are at an elevated risk of exposure to unhealthy, toxic, and even deadly air. The equation for healthy air, however, is much more complex than a formula of emissions from factories and automobiles; it involves the dynamic interplay between infrastructure, waste management, and public health — among other disciplines.
Explore JHU Healthy Air Researchers here .
Innovation & Economic Progress
The development of novel technologies is integral to our ability to mitigate and adapt to the climate crisis. From batteries for the storage of renewable energy and optimized grids for its delivery and use to carbon capture technologies, the potential for innovation is endless and promotes a diversified, strengthened circular economy in the process.
Explore JHU Innovation & Economic Progress Researchers here .
Sustainable Ecosystems & Resource Use
Each day humans use and thus consume a multitude of products, the production and consumption of which directly impact the planet and human health. Hopkins faculty are working to understand how materials and products can be made and used so that they meet the needs of consumers without harming our fragile, diverse ecosystems.
Explore JHU Sustainable Ecosystems & Resource Use Researchers here .
Sustainable Food Production & Diets
Land is where we spend nearly our entire lives and, as such, it provides us with a plethora of environmental services — including food and energy. Yet, current land-use practices and food systems are contributing to ecosystem degradation and chronic diseases. Hopkins faculty across disciplines are working to shape policy and ecosystem landscapes so that all people have access to nutritious, sustainable, and equitable diets.
Explore JHU Sustainable Food Production & Diet Researchers here .
Interested in an Area?
Dive into our curated directory for researchers at Hopkins championing the sustainable movement and filter by your area of choice.
Bloomberg Distinguished Professorships
Among the world’s most accomplished interdisciplinary scholars, these faculty link academic disciplines, open novel fields of inquiry, and invigorate the entire community, all in the service of tackling society’s most complex problems. BDPs are appointed in two or more schools or divisions, including academic centers reporting directly to the provost, or in two or more departments within a single school. They are grouped together as “clusters” under a common theme. In 2021, the Provost’s Office announced a new phase of BDP hires including those for clusters in Climate, Resilience, and Health and Sustainable Transformation and Energy.
Research at JHU
Researchers at our nine academic divisions and at the university’s Applied Physics Laboratory have made us the nation’s leader in federal research and development funding each year since 1979. Find additional information on funding opportunities, awards and initiatives, and ongoing projects.
Log in using your username and password
- Search More Search for this keyword Advanced search
- Latest content
- Current issue
- JME Commentaries
- BMJ Journals More You are viewing from: Google Indexer
You are here
- Volume 49, Issue 6
- Reimagining research ethics to include environmental sustainability: a principled approach, including a case study of data-driven health research
- Article Text
- Article info
- Citation Tools
- Rapid Responses
- Article metrics

- http://orcid.org/0000-0001-8111-2730 Gabrielle Samuel 1 ,
- http://orcid.org/0000-0002-7885-9136 Cristina Richie 2
- 1 Department of Global Health and Social Medicine , King's College London , London , UK
- 2 Philosophy and Ethics of Technology Department , Delft University of Technology , Delft , Netherlands
- Correspondence to Dr Gabrielle Samuel, Global Health and Social Medicine, King's College London - Strand Campus, London, London, UK; gabrielle.samuel{at}kcl.ac.uk
In this paper we argue the need to reimagine research ethics frameworks to include notions of environmental sustainability. While there have long been calls for health care ethics frameworks and decision-making to include aspects of sustainability, less attention has focused on how research ethics frameworks could address this. To do this, we first describe the traditional approach to research ethics, which often relies on individualised notions of risk. We argue that we need to broaden this notion of individual risk to consider issues associated with environmental sustainability. This is because research is associated with carbon emissions and other environmental impacts, both of which cause climate change health hazards. We introduce how bioethics frameworks have considered notions of environmental sustainability and draw on these to help develop a framework suitable for researchers. We provide a case study of data-driven health research to apply our framework.
- research ethics
This is an open access article distributed in accordance with the Creative Commons Attribution 4.0 Unported (CC BY 4.0) license, which permits others to copy, redistribute, remix, transform and build upon this work for any purpose, provided the original work is properly cited, a link to the licence is given, and indication of whether changes were made. See: https://creativecommons.org/licenses/by/4.0/ .
https://doi.org/10.1136/jme-2022-108489
Statistics from Altmetric.com
Request permissions.
If you wish to reuse any or all of this article please use the link below which will take you to the Copyright Clearance Center’s RightsLink service. You will be able to get a quick price and instant permission to reuse the content in many different ways.
Introduction
Dominant research ethics paradigms often revolve around ethics principles that are concerned with the protection, rights, safety and welfare of individual research participants. These paradigms can be traced back to a number of historical ethics frameworks developed in response to atrocities in biomedical/clinical research in the 20th century, 1 and include the 1968 Declaration of Helsinki 1 and the subsequent 1979 Belmont Report. 2 These frameworks aim to guide physicians and researchers in appropriate clinical research ethics conduct, with relevant ethical principles including the need for research to respect individual research participants in group or individual settings; the need to ensure that research design minimises individual risk while maximising potential societal benefit; and the need to ensure fair practices in the selection of individuals for participation in research studies.
While individualised risk has long been a focus of research ethics frameworks, strong criticism exists around it. In an interconnected world it is difficult to argue that the impacts of individual research treatment would not affect others, particularly in the closer communities of friend and family groups. Carol Gilligan’s work on care ethics 4 and the notion of relational autonomy both point to the networks that impact ethical decision-making within healthcare. Furthermore, concerns have long been raised about the appropriateness of placing individual risk ahead of communitarianism , especially in research areas that are less concerned with individual health, such as global health research. Public health scholars have long pointed to the moral status of the community in research ethics considerations, 5–8 whereby community harms are more than the sum of individual values and interests and relate to questions associated with whether communities will be beneficiaries of the research, or even whether they share the same goals as the researchers. 9–11 Multiple authors have pointed to the abusive practices and problematic studies conducted with tribes, indigenous populations, and minoritised and marginalised communities worldwide over the past decades, which have failed to consider community harms associated with violating widespread trust or taking ownership of a community’s stories. 10 For these reasons Emmanuel and Weijer 9 emphasise the importance of an ethical principle of ‘respect for community’ alongside more individual principles related to risk and exploitation, such that scholars need to devote careful attention to understanding the sociopolitical impact of research on communities as a whole and not only to individuals, 7 12 13 remembering that individuals are part of the whole community.
While concerns about community harm have expanded moral status considerations beyond those focused on individual risk alone, they are anthropocentric and have stopped short of considering environment-related harms associated with the research process. The environmental impact of the medical industry and health research can be measured by carbon emissions and resource use. The carbon emissions of global healthcare activities, including research, make up 4%–5% of the total world emissions. 14 The Lancet reports that the Sustainable Clinical Trials Group calculated nearly 350 000 national and international trials registered on ClinicalTrials.gov ‘using the average…(to) give a carbon consumption of an estimated 27.5 million tonnes, which is just under a third of the total annual carbon emissions of Bangladesh, a country of 163 million people’. 15 The impact of carbon emissions includes not only climate change, but also health hazards like pollution, significant environmental destruction, use of scarce resources, loss of biodiversity and diminished quality of life for humans. 16 People affected by climate change require medical care, which is predicated on medical research. 17 These treatments release more carbon, locking healthcare into a self-destructive cycle whereby medical research, care and treatments cause medical needs. Hence, healthcare research has a special interest in carbon reduction, not only as a matter of international priority, but also as a commitment to health. In this paper we draw on the concept of sustainability to provide an ethical basis for the inclusion of such environmental harms in health research.
Environment and (bio)ethics
In 1927 Fritz Jahr described bioethics (German: bio-ethik ) as ‘the assumption of moral obligations not only towards humans, but towards all forms of life’. 18 Jahr drew on Rudolf Eisler’s Bio-Psychik , declaring: ‘Respect every living being on principle as an end in itself and treat it, if possible, as such!’ (p230). Almost half a century later in 1971, the term ‘bioethics’ appeared in English with a parallel scope when Van Rensselaer Potter used it to describe a life-ethic for an industrialised society in a precarious ecosystem. For Potter, bioethics was rooted in an intrinsically practical approach to ecologically sustainable life, inclusive of the earth and other organisms. 19 20 Despite bioethics’ environmental origins, since Beauchamp and Childress’ 21 1979 proposition of ‘biomedical ethics’, which focused on the patient–physician relationship through four principles of respect for autonomy, beneficence, non-maleficence and justice, ‘bioethics’ has become widespread conflated with ‘biomedical ethics’. This has erased the ecological origins of bioethics while simultaneously giving rise to the ‘new’ discipline of environmental bioethics. 22
Nevertheless, an increasing number of scholars have advocated bioethics readopt a broader perspective that aims to explore the relationships between individuals and the natural environment. 23–29 They reject that the land and ecosystems are just instrumentally valuable—good because of how humans can use them—but rather argue that our moral sentiments need to extend to the biotic community, to the soils, waters, plants and animals that make up our planet 30 since nature is both inherently valuable—good in itself—and because humans are a part of, not separate from, nature. 30 Most widely recognised ethical theories acknowledge interconnectedness (with people and communities), and it makes moral sense to include the biotic community within this moral framework. 31 They call for a systems approach that considers individuals, populations and environmental factors in understanding (health) practices and policies (for instance, see Lee 25 ; also see Richie 32 ).
Some effort has ensued in the research ethics community in this regard. The European Commission’s Ethics for Researchers —designed for researchers who are preparing an application for research funding from the European Union—includes respect for biodiversity, the environment and ecological balance as one of its 12 golden rules to ethical research conduct. 33 Equally, the All European Academies Code of Conduct for Research Integrity points to the need not to ‘waste resources and [expose the] environment to unnecessary harm’ during research. 34 The National Institutes for Health Research (NIHR) Carbon Reduction Guidelines ‘highlight areas where sensible research design can reduce waste without adversely impacting the validity and reliability of research’.[ 2 ] Similarly, the UK’s research funding body, UKRI (UK Research and Innovation), emphasises that ‘public funds should be deployed with due consideration to value for money and environmental impact across all activities’. 35
At the same time, a recent review of international research ethics frameworks by RAND suggests that such environmental concerns are primarily applied in non-human-centric disciplines; within human participant research, harm is generally considered anthropocentrically in human terms only. 36 If moral reflections are to consider the environment, key unanswered questions include how we should give respect to non-human worlds, especially since human endeavours will always inevitably lead to the destruction of at least some of the biotic community and ecosystems, and how this respect should or could be weighed next to humans (p235). 37 [ 3 ] Despite this, moral obligations to the environment still exist, even if they are anthropocentric and instrumentalising for reasons of self-preservation. The planet and its ecosystems sustain us. Without these ecosystems, humans can neither survive nor flourish, 37 and indeed the destruction of our ecosystem has led to a diminished quality of life for billions of people, including early death, increased morbidity and psychological suffering. 38
In the following section we argue that in research ethics frameworks, moral decision-making should extend to the environment. Drawing on the concept of sustainability, we map out what such a research ethics framework would look like.
A research ethics framework based on sustainability
As scholars in healthcare increasingly shift to a broader vision of bioethics and take into account factors associated with non-humans and ecosystems, sustainability has become an important concept. 27 31 32 39–47 Following from the well-cited ‘Brundtland Report’, sustainability is viewed as a forward-looking concept for guiding a wide variety of choices that are grounded on the commitment to the well-being of both current and future populations. 48 , 4
In her work on green bioethics, Richie 26 draws on environmental ethics to propose a green bioethics framework for evaluating the sustainability of medical developments, techniques and procedures. This framework includes four normative principles: distributive justice takes a broad view of the moral community and requires the allocation of basic medical resources before special interest access; resource conservation to provide healthcare needs before healthcare wants; simplicity to reduce dependence on medical interventions; and ethical economics to promote humanistic healthcare instead of financial profit. 26 We draw on this and other frameworks of restraint and justice from environmental bioethics (eg, see Potter and Lisa 49 in Jameton and Pierce 31 ). We modify it to be more aligned with current research ethics frameworks (eg, see Weinbaum et al 36 and Emanuel et al 50 ), thus making it intelligible and persuasive for researchers. In the following sections we map our research ethics framework of five substantive ethics principles: social value, scientific quality, respect for persons, communities and environment, justice, and favourable risk to benefit ratio.
Scientific quality
Proposed research must be conducted in a methodologically rigorous manner, using reliable and valid research design and methods. 51 52 Special attention to possible sample bias or underpowered research is important. Execution of the study is also important to ensure results are valid and answer the research question. A lack of quality leads to wasted resources and time. All research has a carbon footprint even if the results of the study are not published, or unusable for reasons of lack of replicability or lack of reproducibility. Hence, the NIHR suggests a thorough literature review prior to developing a research proposal.[ 5 ]
Social value
Research must be beneficial to the participants, community, society 50 51 and environment. More than just refraining from harming the individual, community, society or environment, it should proactively lead to improvements in health, the environment or well-being, or act as a preliminary step towards this. Anything short of this could expose individuals to harms without there being a worthy pursuit (especially if clinical research), or more broadly divert resources from other valuable pursuits. Since all research requires resources, maximal benefits should be prioritised since the consequence of research is increased carbon emissions and risks of climate change health hazards.
Respect for persons, communities and environment
Respect for persons extends further than respect for autonomy, and considers one’s moral attitude towards others and the actions towards others that result from and exemplify this attitude. 53 Respect for communities allows a broadening of this concept to include a variety of cultural norms, including those which place less emphasis on individual autonomy and autonomous decision-making than is the norm in some cultures. 54 Procedural principles to help with respecting persons and communities include, for example, the need for trustworthiness, transparency, privacy and ownership, accountability, autonomy, engagement, the need for consent, and the right to withdraw. 36 51 53 Respect for the environment includes taking environmental destruction into consideration by considering the environmental impacts associated with the research endeavour, particularly when that destruction occurs in places which may not directly benefit from the outputs, for example, clinical trials in the developing world, or in places where natural resources are used, not replenished and not properly compensated for (eg, harvesting of medicinal plants in a rainforest, mining).
This has historically referred to fair participant selection based on the scientific goals of the proposed research. 50 51 This also refers to the fair treatment of individuals and communities beyond research-based activities to ensure that those individuals or communities who take part in research are those most likely to benefit. It also refers to environment-associated harms and benefits associated with the research endeavour. This adheres to Nancy Fraser’s 54 work on justice, which proposes an ‘all subjected principle’, such that ‘all those who are subject to a given governance structure have moral standing as subjects of justice in relation to it’ and that ‘for any such governance structure, the all subjected principle matches the scope of moral concern to that of subjection’. Brock’s work is useful here too. She sees a role for both state-bound and global justice when considering duties in healthcare. 55 She explains that we should give special attention to those within our own state, but we have a moral obligation to make low or reasonable modifications to our own governance structures because of the negative duty to refrain from harming others. Following this premise, if low or reasonable modifications to our own governance structures would decrease harm caused to others, we have a moral responsibility to make these modifications. This is particularly pertinent for people living in affluent countries and their obligations for those who live in extreme poverty in developing countries, and particularly links to the risk to benefit ratio principle that requires finding the optimum research methodology that allows these risks to be minimised.[ 6 ]
Favourable risk to benefit ratio
This is a key aspect of research ethics frameworks that is also related to principles of proportionality, beneficence and non-maleficence. Historically, a favourable risk to benefit ratio involves weighing the individual risk versus individual and/or collective benefit from the research in a utilitarian way (and more recently assessing community risk/benefit). To be truly utilitarian, and to consider all links within a consequentialist pathway, risk to benefit ratios must include environment-related risks. 31 Jameton and Pierce 31 argue that when these harms are put into the research ethics risk/benefit balance, ‘everyday decisions unquestioned by ethicists and regarded as rational and even praiseworthy may be seen as questionable and possibly maleficent’ (p119). 31
Our proposed principles have direct relevance for health research. In the next section, we present a case study and then apply the principle to demonstrate the feasibility and agility.
Case study: data-driven health research
Health research is becoming increasingly data-intensive. Through the capture and analysis of vast swaths of clinical, imaging and genomic data, other biomarkers, as well as data from wearable devices, social media and environmental exposures, researchers aim to improve detection, diagnosis and treatment of patients and the public. While data-driven health research and any technologies that emerge are viewed as a panacea towards better health and healthcare, they have adverse environmental impacts. This is because they rely on digital infrastructures that are not ‘virtual’ as implied by the metaphors describing them, but have materiality—they involve mining, manufacturing, transport, use and waste, all of which have carbon emissions, and all of which produce toxic and hazardous chemicals as well as other environmental and public health impacts. For health research approaches that rely on artificial intelligence (AI), such as diagnostic tests and healthcare disease prediction, we know that the largest AI models are doubling in necessary compute every 3–4 months, thereby severely outpacing the increasing efficiency of hardware.[ 7 ] Mining and e-waste also have associated environmental, health and well-being harms. 56 58 For example, unregulated resource recovery from e-waste landfills has led to the generation of hazardous by-products shown to be present in those living around informal e-waste sites, at levels vastly exceeding recommended safety levels (see Gabrys 59 and Ngo et al 60 ).
Over the past decades, the digital sector has worked hard to drive efficiency gains.[ 8 ] However, the most recent estimate of the sector’s contribution to global carbon emissions has been calculated between 2.1% and 3.9% global emissions. 61 While health research only comprises a small proportion of all digital technology, health is the fastest growing sector in the datasphere 62 and will become an increasingly important contributor, with proteomics, metabolomics and genomics all data-intensive solutions. Communication and media scholar Mel Hogan emphasised that by 2025, between 100 million and 2 billion human genomes will have been sequenced globally, using some 40 exabytes of data. 63 The UK 100,000 Genomes Project, which has sequenced 100 000 genomes, is 21 petabytes, 64 and by 2025 the UK Biobank database—a leading biobank internationally—is expected to grow to 15 petabytes, an amount of data equivalent to that created annually by the Large Hadron Collider.[ 9 ]
Moreover, as other sectors decrease their environmental impacts, the digital sector, including the digital aspect of health research, will increase consumption as it acts as an enabling technology. Backfire is also a concern, whereby the move towards increased digital efficiency, without constraints, results in more, not less, consumption. For example, app-based ridesharing increases use of vehicles instead of carbon neutral forms of transportation like walking and biking, thus ‘cancelling out 68% to 77% of CO 2 emission reductions and 52% to 73% of aggregated social benefits (including congestion, air quality, carbon dioxide emissions, noise) expected from ridesharing’. 66 While increasing the efficiency of digital technologies has historically been drawn upon as a solution to increased consumption, these efficiency gains are slowing.
The move to renewables is also only a partial solution because of its large dependency on mining, as well as its poor recycling prospects. Finally, while health research promises to lead to better health, there is often a lack of clarity about whose health and whether those who will benefit are those who are already experiencing greater access to healthcare. For those not receiving these benefits, health research may amount to only health risks in the form of environmental impacts. 67
In the following sections we map out how researchers, ethicists and healthcare professionals can think about these issues through our principle-based research ethics framework.
Data should not be collected and analysed without ensuring that the research outputs will be of sufficient quality (considering issues of bias, etc). The storage and processing of data are not harm-free and should only be collected and/or analysed if there is an appropriate reason for doing so, such as translatability to significant medical progress, deep gains in knowledge, and the potential for widespread and just dissemination of any developments.
Research should cobenefit humans, communities, society and environment. Social value could mean prioritising more low-tech research rather than energy-hungry data analyses, especially when low-tech research is likely to produce positive health benefits that are equal or greater than high-tech. For example, addressing social, economic, commercial and political determinants of health is likely less impactful on the environment. This is because it is often based on preventive medicine and low-tech interventions, rather than high-tech, reactive solutions that may only lead to benefit for the few who have access to medical infrastructures and sophisticated medical care.
Respect for persons, communities and the environment
For data-driven health research, respect for persons and communities entails respecting all of those affected by the research. It involves community and individual engagement, the availability of readable and digestible information, transparency on how the data are regulated and the protections in place for individuals and communities whose data may be used, and accountability pathways. 53 This can be collected and published online in an easily searchable database. Moreover, how this is used should be part of open-access articles and reports for the benefit of those in the broader scientific community.
Respect for the environment includes awareness of the environmental impact of the research and taking steps to reduce this. At one level, this could involve, for example, optimising algorithms to ensure they have as minimal impact on resource use and carbon emissions or choosing data centres with considerations of sustainability in mind (eg, if the energy they use to power them is ‘dirty’ or ‘clean’, non-renewable or renewable). A range of calculators can help researchers assess the environmental impact of their data-driven practices, and there are various guidelines and frameworks to assist. 68 At a higher level, as researchers use more data, consumption and environmental impact will increase and this must be considered. Respecting the environment means minimising our data use as much as feasibly possible.
For data-driven health research, this refers to, for example, the fair collection, storage, use, linkage and sharing of data, 53 as well as attention to equity and benefit sharing of research outcomes. Consideration must also be given to environment-related harms. This includes those involved in mining minerals used in digital technologies, manufacturing them and recycling/disposing of them. This also includes aspects of social justice, for example, questioning the inequalities associated with the use of turks to analyse data. Justice must also consider how research results will be used in terms of the long-term implications and carbon expenditures.
Risk to benefit ratios need to include weighing up individual, community and environmental risk against benefit. As historically noted, this decision will include some measure of subjectivity, but overall should focus on minimising harm as much as possible. This can be achieved by, for example, buying repurposed machines where possible, using data centres that are powered by renewables and having appropriate recycling infrastructures for digital technologies. However, reliance on ‘recycling’ still requires resources. Hence, the familiar environmental manta ‘reduce, reuse, recycle’ is relevant: recycling should be the last resort on the path to sustainability, not the default.
As the levels of atmospheric carbon are already over safe levels of 350 parts per million, 69 research must be done parsimoniously in ways that neither suppress scientific invention and creative nor threaten the health of people and the planet. We have mapped out a research ethics framework that allows us to do this.
Ethics statements
Patient consent for publication.
Not required.
- World Medical Association
- National Commission for the Protection of Human Subjects of Biomedical and Behavioral Research
- Kraft SA , et al
- Buchanan DR ,
- Adashi EY ,
- Walters LB ,
- Menikoff JA
- Gbadegesin S ,
- Emmanuel EJ ,
- Friesen P ,
- Redman B , et al
- Tsosie KS ,
- Yracheta JM ,
- Dickenson D
- Nelson RM , et al
- Emanuel EJ ,
- Karliner J ,
- Slotterback S
- Adshead F ,
- Al-Shahi Salman R ,
- Aumonier S , et al
- McMichael AJ
- Knowlton K ,
- Rotkin-Ellman M ,
- Geballe L , et al
- Van Rensselaer P
- Beauchamp T ,
- Childress J
- ↵ Richie C. a brief history of environmental bioethics . AMA J Ethics 2014 ; 16 ( 9 ): 749 – 52 . doi:10.1001/virtualmentor.2014.16.9.mhst2-1409 OpenUrl
- Jennings V ,
- Jameton A ,
- European Commission
- Weinbaum C ,
- Landree E ,
- Blumenthal MS
- Cunsolo A ,
- ten Have H ,
- Lucivero F ,
- Lucassen AM
- Health Care without Harm
- Buettner PG ,
- Eckelman MJ ,
- Mortimer F ,
- Isherwood J ,
- Wilkinson A , et al
- Social Care Institute for Excellence
- Oxford University Press
- Potter VR ,
- Wendler D ,
- Tsoka-Gwegweni JM ,
- Wassenaar DR
- Owen Schaefer G ,
- Mancini L ,
- Eslava NA ,
- Traverso M , et al
- Dorninger C ,
- Wieland H , et al
- Watchalayann P ,
- Nguyen DB , et al
- Freitag C ,
- Berners-Lee M ,
- Widdicks K , et al
- Reinsel D ,
- Somavilla L
- Coulombel N ,
- Boutueil V ,
- Liu L , et al
- Hardcastle F ,
- Grealey J ,
- Lannelongue L ,
Funding This work received funding from Wellcome (222180/Z/20/Z).
Competing interests None declared.GS is the guarantor. CR's research was partially funded by the Technology University of Delft/ Erasmus Medical College Convergence ethics project.
Provenance and peer review Not commissioned; internally peer reviewed.
↵ Such as World War II, the Tuskegee Syphilis Study and the Henrietta Lacks case. The Tuskegee Syphilis Study was a longitudinal study conducted by the US Public Health Service in Tuskegee, Alabama, in which approximately 600 African Americans participated between 1932 and 1972. In 1972 it was revealed that the participants had received a dishonest explanation for their involvement in the research, and despite existing treatment for their condition—penicillin—they had been prevented from getting this treatment so that the research could continue. Lacks was an African American woman whose biospecimens were collected during a cervical cancer biopsy and later developed into the profitable HeLa cell line without her consent. 3
↵ See https://www.nihr.ac.uk/documents/the-nihr-carbon-reduction-guidelines/21685 .
↵ Holmes Rolston III discusses that obligations to protect non-human worlds are perhaps better understood at the species and ecosystem level. 30 He also provides more detail on the various ways in which value is ascribed to non-humans.
↵ In this report, sustainable development is defined as ‘meet(ing) the needs of the present without compromising the ability of future generations to meet their own needs’.
↵ https://www.nihr.ac.uk/documents/the-nihr-carbon-reduction-guidelines/21685 .
↵ Also see Mancini et al 56 and Hickel et al . 57
↵ Open AI, “AI and Compute,” May 16, 2018, at https://openai.com/blog/ai-and-compute/ .
↵ Mainly for business reasons, but more recently to address considerations of the environment. 65
↵ https://www.ukbiobank.ac.uk/learn-more-about-uk-biobank/news/uk-biobank-creates-cloud-based-health-data-analysis-platform-to-unleash-the-imaginations-of-the-world-s-best-scientific-minds .
Read the full text or download the PDF:
Other content recommended for you.
- Environmental sustainability and the paradox of prevention Cristina Richie, Journal of Medical Ethics, 2023
- What would an environmentally sustainable reproductive technology industry look like? Cristina Richie, Journal of Medical Ethics, 2014
- Call for sustainable food systems including (medical) nutrition for hospitalised children and their families Sascha C A T Verbruggen et al., Frontline Gastroenterology, 2024
- Health justice in the Anthropocene: medical ethics and the Land Ethic Alistair Wardrope, Journal of Medical Ethics, 2020
- Environmental sustainability and the carbon emissions of pharmaceuticals Cristina Richie, Journal of Medical Ethics, 2021
- Climate change and cattle farming Jonathan Statham et al., In Practice, 2017
- Assessing the impact on chronic disease of incorporating the societal cost of greenhouse gases into the price of food: an econometric and comparative risk assessment modelling study Adam D M Briggs et al., BMJ Open, 2013
- Carbon footprinting for the gastroenterologist Sandeep Shivananda Siddhi et al., Frontline Gastroenterology, 2024
- Integrating climate action for health into covid-19 recovery plans Kristine Belesova et al., BMJ, 2020
- Carbon footprint of hospital laundry: a life-cycle assessment Joseph John et al., BMJ Open, 2024
Loading metrics
Open Access
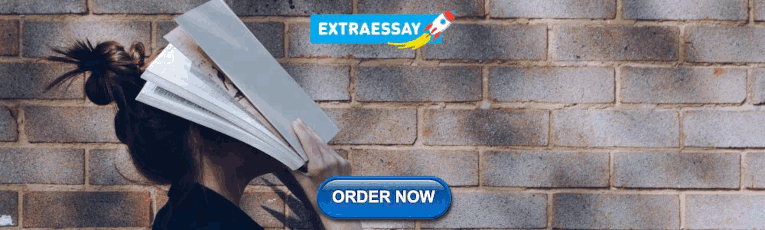
Ten simple rules to make your research more sustainable
Contributed equally to this work with: Anne-Laure Ligozat, Aurélie Névéol
* E-mail: [email protected]
Affiliations Université Paris-Saclay, CNRS, LIMSI, Orsay, France, ENSIIE, Evry-Courcouronnes, France

Affiliation Université Paris-Saclay, CNRS, LIMSI, Orsay, France
- Anne-Laure Ligozat,
- Aurélie Névéol,
- Bénédicte Daly,
- Emmanuelle Frenoux
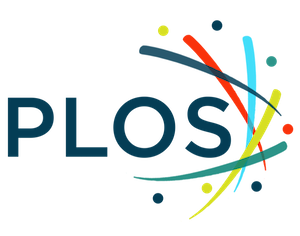
Published: September 24, 2020
- https://doi.org/10.1371/journal.pcbi.1008148
- Reader Comments
Citation: Ligozat A-L, Névéol A, Daly B, Frenoux E (2020) Ten simple rules to make your research more sustainable. PLoS Comput Biol 16(9): e1008148. https://doi.org/10.1371/journal.pcbi.1008148
Editor: Russell Schwartz, Carnegie Mellon University, UNITED STATES
Copyright: © 2020 Ligozat et al. This is an open access article distributed under the terms of the Creative Commons Attribution License , which permits unrestricted use, distribution, and reproduction in any medium, provided the original author and source are credited.
Funding: This work was supported by CNRS, ENSIIE, and Université Paris Saclay. The funders had no role in study design, data collection and analysis, decision to publish, or preparation of the manuscript.
Competing interests: The authors have declared that no competing interests exist.
Introduction
Sustainable development can be defined as a principle that regulates human activity without causing irreparable damage to the Earth's natural system. It also aims to preserve resources so that future generations can benefit from them as much as present generations. To address the global challenges today's world faces to manage the impact of human activity on the environment, the United Nations have defined a set of sustainable development goals to be achieved in the next decade [ 1 ].
The climate changes induced by human activities have been accelerating alarmingly as reported by scientists since 1979 [ 2 ]. Scientists can observe an increase in pollution (e.g., depletion of oxygen in water, eutrophication), natural resource scarcity, and a significant and accelerated loss of biodiversity. All these changes have led geologists to propose the Anthropocene as a new geological epoch, reflecting the impact of human activities on Earth's ecosystems[ 3 ].
To mitigate this effect, a paradigmatic shift represented by sustainable development is needed in all fields of human activities, including research. As individuals and researchers, we are concerned with these challenges and deeply aware of the necessity to be more sustainable. But in practice, what does this entail? How can a researcher's activity be “sustainable,” and how do we integrate sustainable practices into research projects? Where do we start?
There is currently no global policy from French research institutes to federate collective action of the scientific community towards sustainable development goals, but working groups focusing on sustainable development have published recommendations [ 4 ]. There are also examples of good practice in the United Kingdom (S-Labs [ 5 ], Laboratory Efficiency Assessment Framework [ 6 ]) and the United States (International Institute for Sustainable Laboratories [ 7 ], My Green Lab [ 8 ]).
Taking action to address the emergency situation is not only a moral responsibility we have as citizens but also a necessary contribution to gathering an understanding of the impact of research activities on the environment and how to make them more sustainable.
This article is the result of the work carried out by the "sustainable development" committee created at the French Laboratoire d'informatique pour la Mécanique et les Sciences de l'Ingénieur (LIMSI) [ 9 ] in 2019 to bring together researchers interested in addressing these questions in the context of the activities of our laboratory, which conducts theoretical and experimental research in a diversity of scientific fields, including fluid mechanics, energetics, human language technology, human machine interaction, medical informatics, and augmented and virtual reality.
The first task of the committee was to assess the carbon footprint of research activities over the year 2018 [ 10 ]. The next task is to analyze the results of this study and draw a roadmap towards reducing the carbon footprint and, more generally, the environmental impact [ 11 ] of our research activities in subsequent years.
Herein, we propose a list of actionable rules to facilitate the contribution of anyone in the community towards sustainable research. We selected a set of rules that address a variety of topics with different levels of potential impact with the goal of illustrating the breadth of possible actions.
Rule 1: Cherry-picking is allowed
Why does it matter.
It can be daunting to think about all you should be doing to strive towards sustainability. However, in the words of popular wisdom, Rome wasn't built in a day, and every little thing helps. We want to acknowledge here that integrating sustainability into our research is a big step for many of us and that this change may need to be gradual, according to behavioral theory [ 12 ]
How to address it?
You can start your path towards sustainable research today by picking only one of the suggestions below and committing to it. Depending on your particular field of research or interests, some rules may be easier to implement than others.
Rule 2: Be informed
Information is the foundation of sustainable action. According to the Paris Agreement [ 13 ], we have a global goal of achieving carbon neutrality in 2050 and halving current carbon emissions by 2030. Drastically reducing the carbon footprint of human activities can only be achieved if we are well aware of the specific impact of the different carbon-emitting activities.
Research institutes should ensure that their staff receive some training on environmental issues related to energy, climate and biodiversity. Training courses are available on this subject, including Massive Open Online Courses (MOOC) through popular platform such as Université Virtuelle Environnement et Développement Durable (UVED) [ 14 ] in French and Coursera [ 15 ] in English. The sustainability literacy test [ 16 ] is a tool approved by the United Nations for learning general knowledge relating to the environment that could also be used to enhance workers’ and students’ knowledge. In addition, general public documents such as summaries of Intergovernmental Panel on Climate Change (IPCC) reports or reports from think tanks such as the The Shift Project or the Green Alliance can also be used as information material, as well as documents from environmental nongovernmental organizations such as 350.org or Greenpeace. Awareness of these issues will facilitate their inclusion in lab operations and scientific work.
Evaluating the carbon footprint of your lab/institute is an excellent start. An information search for reports of carbon footprint assessments conducted by laboratories or institutes in the same field can also help identify major trends. Typically, for research labs, travel accounts for a significant portion of carbon emissions. Other major sources of carbon emissions include electricity used for building operations as well as computer power. In France, the labos1point5 [ 17 ] collective is offering support to labs interested in assessing their carbon footprint.
Carbon footprint assessment can also be done at the scale of specific research activities. Researchers can apply their knowledge of how to evaluate and compare the impacts of two alternatives. For example, from an environmental point of view, is it better to continue using legacy equipment that may require more power or to invest in new equipment that will require less power but incur environmental construction costs [ 18 ]? Which videoconference system incurs the lowest energy consumption [ 19 ]?
Although these questions may be hard to answer, some of them can be addressed using widely recognized methodologies, such as Life Cycle Assessment (LCA). LCA enables the assessment of environmental impacts of a service or product by taking into account all the stages of its life cycle according to different criteria, including but not limited to carbon dioxide CO 2 measurement. This again requires that research staff be trained on these methodologies, in order to apply them properly. The results obtained may differ substantially on a case-by-case basis because the assessment is dependent on the location and specific set-up. For example, whether the electricity used comes from low-carbon sources will have an impact. In some cases, the LCA will nevertheless remain difficult if key relevant data (e.g., electric power provenance) is not available.
Rule 3: Prefer train over plane
The main source of global CO 2 equivalent emissions in several research institutes is travel. For example, at LIMSI, in 2018, travel accounted for 50% of the total emissions [ 10 ], including about 35% for transportation to attend scientific meetings or conduct field work and an additional 15% for employees’ commutes. Other case studies at two academic institutions in Switzerland and in the US also found travel to account for a large share of their carbon footprint, with air travel alone accounting for 30% of all CO 2 emissions [ 20 , 21 ].
Fig 1 presents a sample comparison between plane and train travel for two sample itineraries to illustrate the CO 2 emission gain offered by train travel for medium range journeys: one international journey within Europe (Paris–Turin, 584 kilometers/363 miles) and one domestic journey withing the US (New York, New York–Washington, DC, 474 kilometers/295 miles).
- PPT PowerPoint slide
- PNG larger image
- TIFF original image
Plane emissions were calculated with the https://co2.myclimate.org/en/flight_calculators/newmyclimate flight calculator. Flight durations are estimated using https://www.expedia.fr/expedia . Train emissions and durations come from oui.sncf/SNCF for the Paris–Turin journey and from https://www.virail.frvirail for the New York, New York–Washington, DC journey.
https://doi.org/10.1371/journal.pcbi.1008148.g001
This data shows that carbon emissions associated with train travel represent a mere fraction of those associated with plane travel (9.2% for New York/Washington and 1% for Paris/Turin), while travel duration increases by about 2 hours (precisely 2 hours 12 minutes for New York/Washington and 2 hours 21 minutes for Paris/Turin), which is arguably equivalent to the time associated with air travel formalities, including city/airport commute and airport security procedures.
This data shows that traveling by train instead of plane can massively reduce the footprint of academic travel. While travel is perceived as essential to a researcher's activity [ 22 ], it was also shown that air travel has a limited influence on academic professional success [ 23 ] for senior researchers. Therefore, train travel should be favored whenever possible. We also encourage researchers to reduce their travel footprint by favoring attendance to scientific meetings in locations that can be reached by train and to limit their conference travel, which scientists seem willing to do [ 24 , 25 ].
This rule applies mainly for short distance travel, which only accounts for a fraction of academic travel. Typically, the train is not an option for traveling from Paris (France) to San Franciso, California (US). The impact of a Paris–San Francisco round-trip flight in terms of CO 2 emissions (2.9 tons according to myclimate) is roughly equivalent to 10 times that of a domestic Paris–Toulouse round trip (314 kg according to myclimate). Favoring train over plane will not reduce emissions related to long-distance trips and thereby may have limited impact over global travel-related emissions if long-distance travel accounts for the majority of travel. As a result, it is necessary to limit long-distance travel by assessing the need for travel, encouraging local collaboration, and adopting publication methods that restrict travel, such as journal publications or domestic conferences.
Rule 4: Take advantage of remote participation
As discussed above, it is necessary to limit long distance travels because they incur a high level of carbon emissions within the overall travel category, which is a major source of emissions for research institutions.
Remote participation in conferences and webinars can be encouraged and facilitated.
Pioneer events showed that entirely remote conferences can be organized to the satisfaction of an overwhelming majority of participants: 10 years ago, computational biologists published a set of guidelines for organizing such events as an effective low-cost educational strategy [ 26 ]. More recently, the University of California at Santa Barbara also devised a plan to organize remote conferences [ 21 ] in which conference participants were invited to record their talks ahead of the event; the videos were then made available on the conference website, and direct interaction with the authors was supported by message forum within the conference timeline. A similar set-up was used for the Cochrane Colloquium 2019 after the event had to be cancelled due to local political events [ 27 ]. A more complete list of conferences aiming to limit their carbon emissions is available at https://www.appropedia.org/List_of_low-carbon_conferences , and recent guidelines provide support for organizing non–real-time events [ 28 ]. The recent global public health crisis due to the COVID-19 outbreak is providing additional motivation for organizing remote events[ 29 ].
These events show that it is technically feasible to engineer fully remote participation in conferences. However, one of the benefits of conference participation is the networking and personal interactions between colleagues occurring during coffee breaks and social events. For this reason, the scientific community may want to continue fostering in-person meetings. To support this middle-ground solution, remote participation can be promoted as a systematically available option for all conferences and meetings [ 30 ]. This way, researchers may balance their conference attendance between in-person and remote attendance.
Another option that can help reduce the carbon footprint of conferences is to create small groups of participants that can meet in person in a local venue to attend a remote event. The 2010 "Signs of Change" conference was organized according to this model and offered five locations throughout New Zealand for participants to meet [ 31 ].
Rule 5: Work collectively and reproducibly
Many of the resources allocated to research activities—including resources that have an impact on the environment—are wasted due to a multiplicity of factors related to the organization, planning, and evaluation of research [ 32 ].
For example, the inadequate use of statistical methods or the fact that novelty is valued more than reproducibility results in waste that could be otherwise avoided. The practice of sharing protocols, research material such as code, and results contributes to collective work and avoids waste in the form of duplicate efforts.
Applying the classic scientific method is particularly useful here. First, do a thorough literature search to identify useful related work to a new project. If existing systems or models addressing the task at hand are identified, they should be reused. Novelty and originality does not necessarily come from new methods; an existing method can be novel if applied to a new context or used differently from usual practice. Furthermore, reusing existing material can also bring added value by demonstrating its reproducibility, documenting a reuse case from the perspective of users with a different background or experimental set-up [ 33 , 34 ].
If a thorough literature search does not uncover readily usable solutions, it makes sense to develop new methods or tools. In this case, working reproducibly will help researchers make the most of their work, by documenting protocols and sharing material. In fact, the value of reproducibility is increasingly promoted by "reproducibility challenges" that seek to reproduce prominent work and gather information about the process. The events Neural Information Processing Systems (NeurIPS) 2019 Reproducibility challenge [ 35 ] and the Shared Task on the Reproduction of Research Results in Science and Technology of Language,"REPROLANG 2020" [ 36 ] are examples of reproducibility tasks in the fields of Natural Language Processing and Machine Learning. In 2020, the Empirical Methods in Natural Language Processing (EMNLP) conference added reproducibility criteria during the submission process about the description of experimental results, parameters, and datasets, based on [ 37 ] and [ 38 ], which is also a good way to promote reproducibility of research work.
Rule 6: Encourage bottom-up sustainable initiatives
Engaging the community on the topic of sustainability will create interest in the topic and in how it is addressed within the community. Empowering members of the community will contribute to the emergence of solutions that are tailored to the community culture and that will find stronger support within the community [ 39 ].
Researchers are creative. Let them implement ideas towards more sustainable practice.
Typically, many of the initiatives cited in this manuscript came from researchers themselves and have been widely adopted. Methods for organizing remote conferences are one example which has shown increased popularity with the recent pandemic.
Supporting this type of initiative (environmental impact of research operation/policy) as a research question will help with researching and adopting some of these ideas. There are many ways this support can translate into actionable policies for a variety of actors in higher education and research: by funding researchers' work in this area, even if it is outside of their main expertise, e.g., labs could fund researchers experiments towards addressing these questions, journals could waive publication fees for this type of work, and institutes could recognize this type of contribution in staff evaluation or provide special allowance to allocate a portion of their time working on these issues.
Rule 7: Evaluate the impact of your research practices
Like all human activities, research has an environmental impact that we need to be aware of (see Rule 2). Until now, we have mainly discussed the impact of the research environment rather than research activities in and of themselves. The raising awareness for environmental issues combined with the increasing energy needed for implementing modern machine learning algorithms has brought about the emerging field of so-called "green" artificial intelligence, which seeks to reconcile powerful computing with environment friendly research [ 40 ].
When conducting research, factor in the direct, indirect, and structural environmental impacts of your research. Direct impact covers the carbon footprint of the operational conduct of the research. For example, it includes the carbon footprint of overall research practice and can be taken into account by addressing questions such as the following: When two methods are otherwise equivalent in performance, which one has the smaller carbon footprint? Did you take computational cost and its environmental impact in your method evaluation/reporting [ 38 , 41 ]?
Indirect and structural impact covers the consequences of the new knowledge or findings obtained as a result of the research. For example, improving the energy efficiency of a learning algorithm could lead to increased experimentation, which, in the long run, does not reduce energy use (this typical rebound effect is described in [ 18 ]). Indirect and structural impact can be taken into account by addressing questions such as the following: What are the consequences of the discoveries we make? Do they contribute to a more sustainable world or, on the contrary, to a runaway machine, even indirectly?
Rule 8: Ask sustainability research questions
Rule 2 and others have highlighted how information is key to address sustainability. Research aims to create new knowledge and therefore can have a contribution to our information on sustainability issues and how to address them.
Computer science (CS) is well positioned for offering analysis of problems using predictive models, simulation and visualization methods that can be applied to a large range of sustainability problems. The National Research Council Committee on Computing Research for Environmental and Societal Sustainability has suggested that "[s]marter energy grids, sustainable agriculture, and resilient infrastructure provide three concrete and important examples of the potential role of IT innovation and CS research in sustainability." [ 42 ]
However, the benefit of work on sustainability research questions must be balanced with the impact of such research (see Rule 7). For example, although information and communication technologies are often considered as a means for reducing energy demands and emissions, a recent study [ 43 ] showed that digitalization actually increases energy consumption. Standard methodologies can be used here, such as attributional LCA (see Rule 2), which takes into account the direct environmental impacts. The more general and long-term impact of research work can be difficult to anticipate or predict. Typically, researchers investigating electricity in the 17th and 18th century may not have foreseen the climate crisis we are facing today due to CO 2 emissions generated by electricity-powered technology. In order to assess research impact at a global level, consequential LCA can also be used. It goes beyond the direct impacts and may require multidisciplinary work involving economists, sociologists, philosophers, etc.
Furthermore, researchers can be encouraged to think about research questions outside of their main expertise (see Rule 6). For example, several French groups with an interest in advancing sustainability policies [ 17 ] drafted incentive and coercive plans to reduce airplane travel in research labs. The incentive measure consists in making it mandatory to compensate the carbon emissions linked to travel by funding compensating projects. The coercive measure consists in banning travel as soon as the yearly carbon quota defined either per lab or per researcher has been reached. They are suggesting enforcing these policies in pilot institutes as a scientific experiment to test adherence and impact.
Rule 9: Transfer ecofriendly gestures from home to the lab
Studies show that individual actions towards sustainability in the form of ecofriendly gestures can contribute towards achieving up to 45% of the carbon footprint reduction that needs to be achieved collectively by 2050 [ 44 ].
Ecofriendly gestures practiced at home by many are also relevant in a professional setting and can have a significant impact. These ecofriendly gestures are not necessarily trivial to implement in the workplace because individuals are not directly involved in the global administration of the infrastructure. Typically, for security and logistical reasons, electric facilities cannot be openly accessible to all. Nonetheless, based on recommendations for carbon reduction [ 44 ] and our own example of carbon emissions at LIMSI [ 10 ], the following points can be brought to the attention of infrastructure management officials within an institute:
- Limit the use of (plastic) disposable material [ 45 ].
- Practice printing sobriety: think before you print and collect printed documents.
- Encourage employees to use soft modes of transport for local commutes (see also Rules 3 and 4).
- Favor local, seasonal, and vegetarian food when organizing events.
- Practice digital sobriety: use less material, make it last as long as possible, and consider donations when research use of otherwise-functioning equipment is no longer appropriate.
- Organize trash collection to encourage or facilitate recycling.
- Consider the feasibility of switching off lights and servers during off–peak-use periods.
Rule 10: Raise awareness
Sustainable actions have an impact both at the individual and collective level. The strength of the impact directly depends on the support of many individuals. As a result, it is important to raise awareness to convince and gain the support of others.
Communication officers at research institutes are in charge of internal communication using diverse means including custom visual tools and social media. They are excellent assets to support an awareness campaign on sustainability issues. For example, the contribution of LIMSI's communication director to the sustainable development committee includes the creation of a series of handouts around the theme of "Mon labo écolo" (My green lab). Fig 2 presents sample handouts produced by the LIMSI communication officer to raise awareness of lab members on major issues such as power use ( Fig 2A ) and travel ( Fig 2B ).
(A) Translation of hand-out content into English: “My Green Lab—BUILDINGS—(electricity) 90,000 € pa, 2/3 for server room (heat) 26,000 € pa (action) Switch off servers, computers, screens, lights when not in use. Open window? Radiator off!” (B) Translation of hand-out content into English: “My Green Lab—TRAVEL—(map) travel is responsible for half of the lab’s carbon footprint, with plane travel accounting for 99% of the travel footprint (action) prefer train over plane; question the necessity of travel.” Text and design by Bénédicte Daly .
https://doi.org/10.1371/journal.pcbi.1008148.g002
These documents are used at LIMSI to promote sustainable action around the lab and can be used/customized as desired.
The goal of this article is to provide researchers with guidance for integrating sustainable practices into their activities. These 10 rules are positioned in the paradigm of science as it is conducted today. We posit that modern environmental and public health issues suggest the need for a massive paradigm shift. Calls to this effect have already been issued within the scientific community [ 46 ], such as the "slow science manifesto" [ 47 ].
In this situation, we stress the importance of cherry-picking and easing into change step by step to avoid being overwhelmed by the magnitude of the task.
A major step towards achieving sustainable research requires being informed about the impact of our activities as well as the impact of the simple choices we can make, as outlined by this set of 10 rules.
Acknowledgments
The authors thank Gabriel Illouz, Mathilde Véron, and members of the sustainable development committee at LIMSI for fruitful conversations during the preliminary stages of preparing this manuscript. Portions of this article were written by post-editing text that was translated from French into English with www.DeepL.com/Translator (free version).
- 1. Sustainable Development Goals;. Available from: https://www.un.org/sustainabledevelopment/sustainable-development-goals/ [cited 2020-04-02].
- View Article
- Google Scholar
- 3. Bonneuil C, Fressoz JB. The shock of the Anthropocene: The earth, history and us. Verso Books; 2016.
- 5. Safe, Successful, Sustainable Lab;. Available from: http://www.effectivelab.org.uk/ [cited 2020-06-23].
- 6. Laboratory Efficiency Assessment Framework;. Available from: https://www.ucl.ac.uk/sustainable/staff/labs/leaf-laboratory-efficiency-assessment-framework [cited 2020-06-23].
- 7. I2SL;. Available from: https://www.i2sl.org/ [cited 2020-06-22].
- 8. my green lab;. Available from: https://www.mygreenlab.org/ [cited 2020-06-22].
- 9. Laboratoire d'Informatique pour la Mécanique et des Sciences de l'Ingénieur LIMSI;. Available from: https://www.limsi.fr/en/ [cited 2020-04-02].
- 10. LIMSI. Bilan d’émissions de Gaz à Effet de Serre 2018 du LIMSI; 2019.
- 11. Global Footprint Network;. Available from: https://www.footprintnetwork.org/ [cited 2020-04-02].
- 13. Paris Agreement; 2016. Available from: https://treaties.un.org/pages/ViewDetails.aspx?src=TREATY mtdsg_no=XXVII-7-d chapter=27 clang=_en .
- 14. Université Virtuelle Environnement et Développement Durable UVED;. Available from: https://uved.fr [cited 2020-04-02].
- 15. Coursera;. Available from: https://www.coursera.org [cited 2020-04-02].
- 16. Sulitest;. Available from: https://www.sulitest.org/ [cited 2020-06-22].
- 17. Labos 1point5;. Available from: https://labos1point5.org/ [cited 2020-04-02].
- 18. Owen D. The Conundrum: How Scientific Innovation, Increased Efficiency, and Good Intentions Can Make Our Energy and Climate Problems Worse. Penguin , editor. Riverhead Books; 2012.
- 27. Cochrane Colloquium 2019;. Available from: https://colloquium2019.cochrane.org/ [cited 2020-04-02].
- 30. Open letter to the American Geophysical Union and to the European Geosciences Union;. Available from: https://climactions.ipsl.fr/wp-content/uploads/2019/10/Open_letter_AGU_EGU.pdf [cited 2020-04-02].
- 31. Signs of Change;. Available from: http://www.signsofchange.org.nz/ [cited 2020-04-02].
- 35. Reproducibility Challenge @NeurIPS 2019;. Available from: https://reproducibility-challenge.github.io/neurips2019/task/ [cited 2020-04-02].
- 36. OLANG 2020. Shared Task on the Reproduction of Research Results in Science and Technology of Language;. Available from: https://lrec2020.lrec-conf.org/en/reprolang2020/ [cited 2020-04-02].
- 37. Joelle Pineau's reproducibility checklist;. Available from: https://www.cs.mcgill.ca/jpineau/ReproducibilityChecklist.pdf .
- 38. Dodge J, Gururangan S, Card D, Schwartz R, Smith NA. Show Your Work: Improved Reporting of Experimental Results. In: Proceedings of the 2019 Conference on Empirical Methods in Natural Language Processing and the 9th International Joint Conference on Natural Language Processing (EMNLP-IJCNLP). Hong Kong, China: Association for Computational Linguistics; 2019. p. 2185–2194. Available from: https://www.aclweb.org/anthology/D19-1224 .
- 39. Zimmerman MA. Empowerment theory. In: Handbook of community psychology. Springer; 2000. p. 43–63.
- 40. Schwartz R, Dodge J, Smith NA, Etzioni O. Green AI; 2019. Available from: https://arxiv.org/abs/1907.10597 [cited 2020-06-17].
- 41. Strubell E, Ganesh A, McCallum A. Energy and Policy Considerations for Deep Learning in NLP. In: Proceedings of the 57th Annual Meeting of the Association for Computational Linguistics; 2019. p. 3645–3650. Available from: https://www.aclweb.org/anthology/P19-1355.pdf .
- 42. Council NR. Computing Research for Sustainability. Millett LI, Estrin DL, editors;.
- 47. Slow Science Manifesto;. Available from: http://slow-science.org/ [cited 2020-04-02].
Environmental Sustainability Research
Microsoft corporate environmental sustainability.
Driving a more sustainable world through mission-driven scientific research and applied technology
Research and innovation to make the world more sustainable
Environmental sustainability is a complex global challenge. Climate change is causing dramatic shifts to the environment and worldwide disruptions of unprecedented scale. The world needs to mobilize to understand and tackle these issues. Yet, many of the technologies we need to make the world more sustainable do not yet exist.
As a research community, we have an opportunity to leverage our expertise in computation, machine learning, modeling and simulation, systems and networking, computer vision and other disciplines to help us understand, model and predict our resource use and our changing climate – and engineer appropriate and effective solutions. As an industrial research lab, Microsoft Research has the capability to take on bold, high risk/high-reward projects, the opportunity to make long-term investments, as well as the ability to devote resources to shorter-term projects to achieve immediate impact.
Environmental sustainability is a significant research area at Microsoft. We are working on a wide portfolio of research projects that push the state of the art in science, engineering and economics to make Microsoft, our customers, and the world more sustainable. We are building on our long-standing relationships with the academic community and external partners, and our interdisciplinary work across chemistry, biology, physics and other fields. From modeling and prediction to understand our changing climate, to prevention and mitigation, we collaborate broadly and tackle difficult challenges using an interdisciplinary approach.
Check out the Microsoft Climate Research Initiative to learn more about how Microsoft and partners are driving toward a carbon negative future together.
Learn more about complementary work on Societal Resilience >

Land Cover Mapping
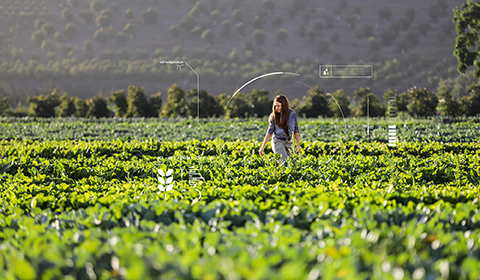
FarmBeats: AI, Edge & IOT for Agriculture
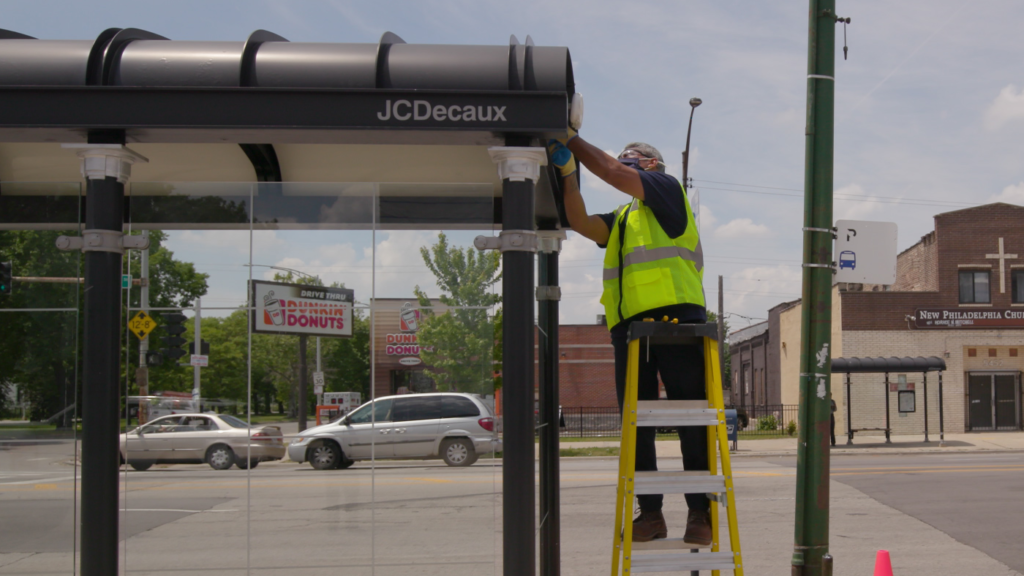
Urban air sensing in Chicago with Project Eclipse
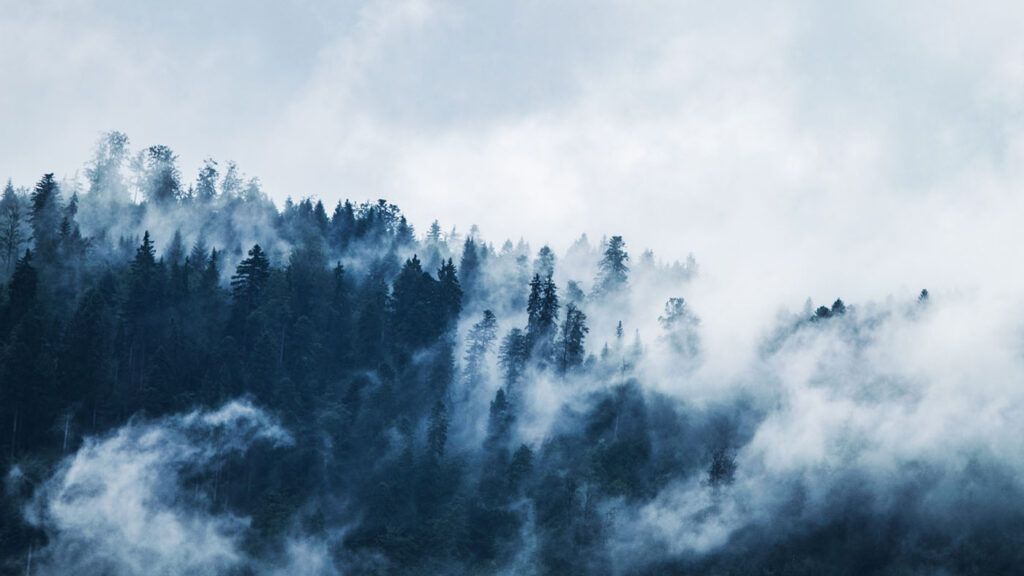
Subseasonal Climate Forecasting
- Follow on Twitter
- Like on Facebook
- Follow on LinkedIn
- Subscribe on Youtube
- Follow on Instagram
- Subscribe to our RSS feed
Share this page:
- Share on Twitter
- Share on Facebook
- Share on LinkedIn
- Share on Reddit
Advertisement
Integrating environmental sustainability into universities
- Published: 05 June 2013
- Volume 67 , pages 71–90, ( 2014 )
Cite this article
- Meredith Ralph 1 &
- Wendy Stubbs 2
7285 Accesses
165 Citations
Explore all metrics
Universities play a fundamental role in addressing global environmental challenges as their education, research and community involvement can produce long-lasting environmental effects and societal change. By demonstrating best practice in their operations, research and teaching, universities have both multiple and multiplier effects on society. For universities to comprehensively address sustainability, a ‘learning for sustainability’ approach needs to be embedded across every aspect of institutional operations in a synergistic way. Using semi-structured interviews, this research explored the factors that influence the integration of sustainability into the operations, teaching and research activities of universities in Australia and England. The research found that individuals, committed to the goal of a more sustainable world, play a vital role in the success of integrating environmental sustainability into universities. The factors critical to enabling universities to undertake the transformational changes necessary to embed environmental sustainability into all university areas included: a strong policy environment, resourcing of strategies, and encouragement of leaders and environmental sustainability advocates. Educating and building the awareness of university staff of the importance of environmental sustainability to future generations was key to a successful strategy.
This is a preview of subscription content, log in via an institution to check access.
Access this article
Price includes VAT (Russian Federation)
Instant access to the full article PDF.
Rent this article via DeepDyve
Institutional subscriptions
Similar content being viewed by others
Enhancing Our Social, Economic & Physical Environments by Embedding Sustainability into University Core Business
Sustainability Science and Education for Sustainable Development in Universities: A Way for Transition
Benchmarking sustainability research: a methodology for reviewing sustainable development research in universities.
Anderberg, E., Plotkin, S., Norden, B., & Hansson, B. (2009). Global learning for sustainable development in higher education: Recent trends and a critique. International Journal of Sustainability in Higher Education, 10 (4), 368–378.
Article Google Scholar
Bekessy, S., Samson, K., & Clarkson, R. (2007). The failure of non-binding declarations to achieve university sustainability: a need for accountability. International Journal of Sustainability in Higher Education, 8 (3), 301–316.
Breakwell, G., & Tytherleigh, M. (2010). University leaders and university performance in the United Kingdom: is it ‘who’ leads, or ‘where’ they lead that matters most? Higher Education, 60 , 491–506.
Brundtland, G. (Ed.). (1987). Our Common Future . Oxford, Oxford University Press: The World Commission on Environment and Development.
Google Scholar
Chambers, D. (2010). Education for sustainability in Australia. The current situation. In: K. Hegarty, D. Chambers, A. Beringer (Eds), 10th Australasian Campuses Towards Sustainability Conference, Melbourne.
Clugston, R., & Calder, W. (1999). Critical dimensions of sustainability in higher education. In W. Filho (Ed.), Sustainability and University Life (pp. 1–15). New York: Peter Lang.
Corcoran, P., & Wals, A. (2004). Preface. In: P. Corcoran, & A. Wals (Eds.), Higher education and the challenge of sustainability problematics, promise, and practise (pp. xiii–xiv). MA, USA: Kluwer Academic Publishers.
Dahle, M., & Neumayer, E. (2001). Overcoming barriers to campus greening. A survey among higher educational institutions in London, UK. International Journal of Sustainability in Higher Education, 2 (2), 139–160.
Daley, J., Edis, T., & Reichl, J. (2011). Learning the hard way: Australian policies to reduce carbon emissions . Melbourne: Grattan Institute.
Evangelinos, K. I., & Jones, N. (2009). An analysis of social capital and environmental management of higher education institutions. International Journal of Sustainability in Higher Education, 10 (4), 334–342.
Fankhauser, S., Kennedy, D., & Skea, J. (2009). The UK’s carbon targets for 2020 and the role of the Committee on Climate Change. In A. Giddens, S. Latham, & R. Liddle (Eds.), Building a low-carbon future The politics of climate change (pp. 100–111). London: Policy Network.
Ferrer-Balas, D., Adachi, J., Banas, S., Davidson, C. I., Hoshikoshi, A., Mishra, A., et al. (2008). An international comparative analysis of sustainability transformation across seven universities. International Journal of Sustainability in Higher Education, 9 (3), 295–316.
Fisher, J., & Bonn, I. (2011). Business sustainability and undergraduate management education: An Australian study. Higher Education, 62 (5), 563–571.
Gagnon, Y.-C. (2010). The case study as a research method. A Practical Handbook . Quebec: Presses de l’Universitie du Quebec.
Haigh, M. (2005). Greening the university curriculum: Appraising an international movement. Journal of Geography in Higher Education, 29 (1), 31–48.
Helferty, A., & Clarke, A. (2009). Student-led campus climate change initiatives in Canada. International Journal of Sustainability in Higher Education, 10 (3), 287–300.
Hopkinson, P. (2011). Developing the campus as a learning resource for student engagement on low carbon futures. In S. Haslett, D. France, & S. Gedye (Eds.), Pedagogy of Climate Change The Higher Education Academy Subject Centre for Geography, Earth and Environmental Sciences pp. 10–24.
Hopkinson, P., Hughes, P., & Layer, G. (2008). Sustainable graduates: linking formal, informal and campus curricula to embed education for sustainable development in the student learning experience. Environmental Education Research, 14 (4), 435–454.
Intergovernmental Panel on Climate Change (2007). Climate Change 2007: Synthesis report summary for policymakers. Contribution of Working Groups I, II and III to the Fourth Assessment Report of the Intergovernmental Panel on Climate Change. Geneva, Switzerland: Core Writing Team. In: R. Pachauri, A. Reisinger (Eds), IPCC pp. 1–104.
Justice, C., Rice, J., Roy, D., Hudspith, B., & Jenkins, H. (2009). Inquiry-based learning in higher education: Administrators’ perspectives on integrating inquiry pedagogy into the curriculum. Higher Education, 58 , 841–855.
Kirwan, W. E. (2010). The 21st Century: The century of the American research university. Innovative Higher Education, 35 , 101–111.
Krizek, K. J., Newport, D., White, J., & Townsend, A. R. (2012). Higher education’s sustainability imperative: How to practically respond? International Journal of Sustainability in Higher Education, 13 (1), 19–33.
Lang, J., Thomas, I., & Wilson, A. (2006). Education for sustainability in Australian universities: Where is the action? Australian Journal of Environmental Education, 22 (2), 45–58.
Levin, K., Cashore, B., Bernstein, S., & Auld, G. (2009). Playing it forward: Path dependency, progressive incrementalism, and the “Super Wicked” problem of global climate change. In IOP Conference Series: Earth and Environmental Science, 6 , 502002.
Lozano, R. (2006). Incorporation and institutionalization of SD into universities: Breaking through barriers to change. Journal of Cleaner Production, 14 (9–11), 787–796.
Lozano, R. (2011). The state of sustainability reporting in universities. International Journal of Sustainability in Higher Education, 12 (1), 67–78.
Merkel, J., Litten, L., & Litten, L. (2007). The sustainabilty challenge. In L. Litten & D. Terkla (Eds.), Advancing Sustainability in Higher Education: New directions for institutional research, no. 134 (pp. 7–26). San Francisco: Wiley Periodicals.
Moore, J. (2005). Seven recommendations for creating sustainability education at the university level. International Journal of Sustainability in Higher Education, 6 (4), 326–339.
Nicolaides, A. (2006). The implementation of environmental management towards sustainable universities and education for sustainable development as an ethical imperative. International Journal of Sustainability in Higher Education, 7 (4), 414–424.
Niu, D., Jiang, D., & Li, F. (2010). Higher education for sustainable development in China. International Journal of Sustainability in Higher Education, 11 (2), 153–162.
Nomura, K., & Abe, O. (2010). Higher education for sustainable development in Japan: Policy and progress. International Journal of Sustainability in Higher Education, 11 (2), 120–129.
Noonan, D., & Thomas, I. (2004). Greening universities in Australia: Progress and possibilities. Australian Journal of Environmental Education, 20 (2), 67–79.
People & Planet (2010). The People & Planet Green League 2010 tables. http://peopleandplanet.org/green-league-2010/table . Accessed 8 December 2011.
Reid, A., & Petocz, P. (2006). University lecturers’ understanding of sustainability. Higher Education, 51 (1), 105–123.
Ryan, A., Tilbury, D., Corcoran, P. B., Abe, O., & Nomura, K. (2010). Sustainability in higher education in the Asia-Pacific: Developments, challenges, and prospects. International Journal of Sustainability in Higher Education, 11 (2), 106–119.
Sammalisto, K., & Lindhqvist, T. (2008). Integration of sustainability in higher education: A study with international perspectives. Innovative Higher Education, 32 (4), 221.
Scott, W., & Gough, S. (2006). Sustainable development within UK higher education: Revealing tendencies and tensions. Journal of Geography in Higher Education, 30 (2), 293–305.
Sharp, L. (2002). Green campuses: The road from little victories to systemic transformation. International Journal of Sustainability in Higher Education, 3 (2), 128–145.
Sherren, K. (2006). Core issues: Reflections on sustainability in Australian university coursework programs. International Journal of Sustainability in Higher Education, 7 (4), 400–413.
Sterling, S., & Scott, W. (2008). Higher education and ESD in England: A critical commentary on recent initiatives. Environmental Education Research, 14 (4), 386–398.
Stern, N. (2006). Stern review on the economics of climate change. http://webarchive.nationalarchives.gov.uk/+/http://www.hm-treasury.gov.uk/independent_reviews/stern_review_economics_climate_change/sternreview_index.cfm . Accessed 21 November 2011.
Sustainable Campus Group (2011). Australian campuses sustainability assessment. Sustainable Campus Group National Reporting Project 2010. http://www.monash.edu/research/sustainability-institute/assets/documents/scg_sustainability-assessment_2010-revised.pdf . Accessed 11 December 2011.
The Australian Research Institute for Environment and Sustainability (2012). ARIES. http://aries.mq.edu.au/ . Accessed 5 February 2012.
Thomas, I. (2004). Sustainability in tertiary curricula: What is stopping it happening? International Journal of Sustainability in Higher Education, 5 (1), 33–47.
Tilbury, D. (2010). Are we learning to change? Mapping global progress in education for sustainable development in the lead up to 'Rio Plus 20'. Global Environmental Research, 14 (2), 101–107.
Tilbury, D. (2011). Sustainability in higher education: A global overview of progress and possibilities. In Higher Education in the World 4. Higher Education’s Commitment to Sustainability: from Understanding to Action . Barcelona: GUNI.
Tilbury, D., & Cooke, K. (2005). A national review of environmental education and its contribution to sustainability in Australia: Frameworks for sustainability . Canberra: Australian Government Department of the Environment and Heritage and Australian Research Institute in Education for Sustainability.
Tilbury, D., Keogh, A., Leighton, A., & Kent, J. (2005). A national review of environmental education and its contribution to sustainability in Australia: Further and higher education . Canberra: Australian Government Department of the Environment and Heritage and Australian Research Institute in Education for Sustainability.
United Nations Educational Scientific and Cultural Organization (2011). Education for sustainable development. http://www.unesco.org/new/en/education/themes/leading-the-international-agenda/education-for-sustainable-development/three-terms-one-goal/ . Accessed 13 July 2011.
University Leaders for a Sustainable Future (2008). Talloires Declaration. http://www.ulsf.org/programs_talloires.html . Accessed 9 August 2010.
van Weenen, H. (2000). Towards a vision of a sustainable university. International Journal of Sustainability in Higher Education, 1 (1), 20–34.
von Oelreich, K. (2004). Environmental certification at Mälardalen University. International Journal of Sustainability in Higher Education, 5 (2), 133–146.
Wals, A., & Blewitt, J. (2010). Third-wave sustainability in higher education: Some (inter)national trends and developments. In P. Jones, D. Selby, & S. Sterling (Eds.), Sustainability Education (pp. 55–74). London: Earthscan.
Wright, T. (2002). Definitions and frameworks for environmental sustainability in higher education. Higher Education Policy, 15 , 105–120.
Wright, T. (2010). University presidents’conceptualizations of sustainability in higher education. International Journal of Sustainability in Higher Education, 11 (1), 61–73.
Yin, R. K. (2009). Case study research: Design and methods (4th ed.). Thousand Oaks, California: Sage Inc.
Download references
Acknowledgments
The authors thank Monash University for financially supporting this research.
Author information
Authors and affiliations.
Environmental Sustainability, Facilities and Services, Monash University, Clayton, Australia
Meredith Ralph
School of Geography and Environmental Science, Monash University, Building 11, Wellington Road, Clayton, VIC, 3800, Australia
Wendy Stubbs
You can also search for this author in PubMed Google Scholar
Corresponding author
Correspondence to Wendy Stubbs .
Rights and permissions
Reprints and permissions
About this article
Ralph, M., Stubbs, W. Integrating environmental sustainability into universities. High Educ 67 , 71–90 (2014). https://doi.org/10.1007/s10734-013-9641-9
Download citation
Published : 05 June 2013
Issue Date : January 2014
DOI : https://doi.org/10.1007/s10734-013-9641-9
Share this article
Anyone you share the following link with will be able to read this content:
Sorry, a shareable link is not currently available for this article.
Provided by the Springer Nature SharedIt content-sharing initiative
- Universities
- Environmental sustainability
- Education for sustainability
- Integration
- Find a journal
- Publish with us
- Track your research
Global Research in Environment and Sustainability

About the Journal
Global Research in Environment and Sustainability (GRES) is an open access and peer-reviewed international journal. Environmental sustainability has become an increasingly important topic in today's world as the impacts of climate change and human activities on the planet continue to grow. Research in this area focuses on identifying solutions to mitigate these impacts and promote sustainable practices. From renewable energy and waste reduction to conservation and ecosystem management, environmental sustainability research is critical for the preservation of the natural world and the well-being of future generations. This research is multidisciplinary, involving scientists, policymakers, and the general public in the pursuit of a sustainable future.
Current Issue
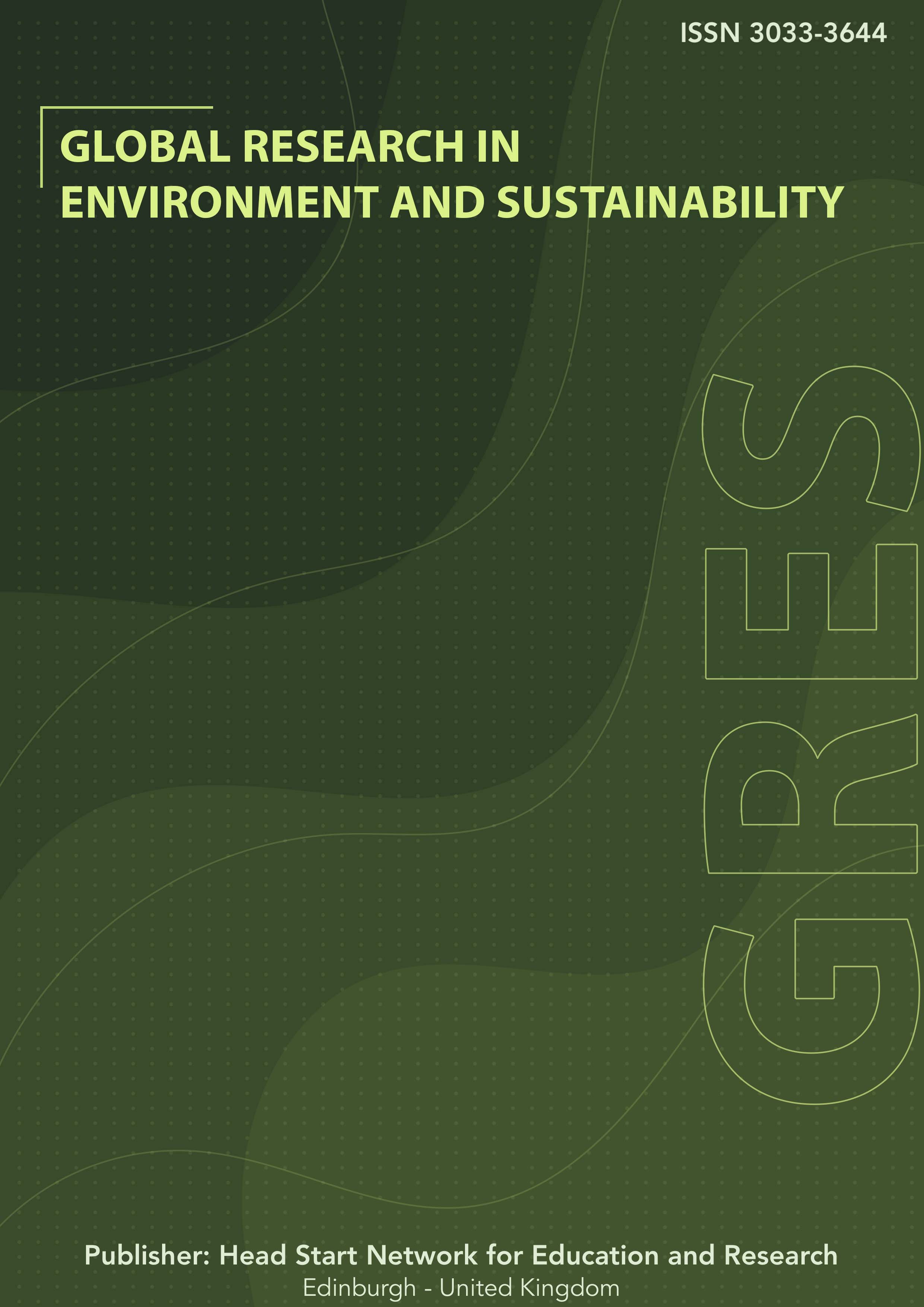
Vol 2, Issue 3, Mar 2024
Estimation of Runoff Using CN Method and Arc GIS for Irrigation Water Accessibility at Kulumsa Watershed, Arsi Zone of Ethiopia
Evaluating substitution of alfalfa hay for concentrate feeds on nutrient intake and growth performance of crossbred dairy heifers, nacl acclimation enhances salt stress tolerance in huckleberry (solanum scabrum mill.) plants, production and chemical assessment of ready to eat snacks produced from cassava, soyabean and cricket composite flour, technical evaluation of low-head drip irrigation system, journal home.
Global Research in Environment and Sustainability (GRES) is an open access and peer-reviewed international journal. This journal is published online on a monthly basis to keep readers up to date with the latest developments regarding environmental sustainability.
Our aim is to provide a platform for researchers, scientists, policymakers, and practitioners to share their latest findings, insights, and innovative solutions in the fields of environmental sustainability and conservation.
With the growing awareness of the urgent need to address climate change and the impact of human activities on the planet, our journal is dedicated to publishing cutting-edge research that addresses the most pressing environmental issues facing our world today. Our articles cover a wide range of topics, including but not limited to renewable energy, sustainable agriculture, water and air pollution, ecosystem management, and biodiversity conservation.
We prioritize rigorous peer-review process to ensure the quality and accuracy of our publications. Our team of experienced editors and reviewers ensures that all submitted papers undergo thorough evaluation and constructive feedback to ensure that the research published in our journal is of the highest standard.
We welcome submissions from researchers and practitioners from all disciplines and regions of the world. Our goal is to foster a diverse and inclusive community of scholars and practitioners who share a common commitment to advancing knowledge and practice in the field of environmental sustainability.
GRES focuses on fundamental and applied environmental science to better understand and provide solutions to global, national, regional, and local environmental challenges. Our multidisciplinary and diverse teams of researchers aim to better understand how Earth systems interact in order to forecast the consequences of those interactions. Our core discipline strengths include:
- Fundamental and applied research in human health
- Ecological risk
- Atmospheric sciences
- Climate science
- Ecological sciences
- Environmental chemistry
- Natural and cultural resources management
- Remote sensing
- Geographical information systems
- Animal behavior
- Biodiversity conservation
- Environmental pollution
- Soil sciences
- Tropical Forest Ecology
- Tropical Wetland Ecology
- Wildlife Ecology and Management.
The subject areas may include, but are not limited to the above domains.
Information
- For Readers
- For Authors
- For Librarians
Global Research in Environment and Sustainability (GRES)
13/5 Whearfield Place, EH11 2PE, Edinburgh
United Kingdom
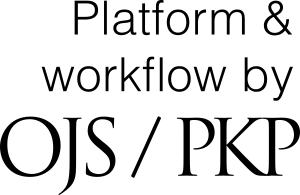

Groundbreaking research in groundwater's role in ecosystem sustainability
Until now, groundwater -- a critical water resource around the globe, especially in dry regions -- has been largely unstudied in its importance and role in sustaining ecosystems. A new groundbreaking research effort led by the SUNY College of Environmental Science and Forestry (ESF) in partnership with University of California Santa Barbara (UCSB), Cardiff University, and Desert Research Institute (DRI) examines the relationship between groundwater and ecosystems across California. Their innovative findings are featured in Nature Water .
Led by Dr. Melissa Rohde, who completed the study as part of her doctoral research in Dr. John Stella's Riparian Echohydrology Lab at ESF, the team used satellite imagery and groundwater monitoring data to identify thresholds of groundwater depth and seasonal change that can support sensitive ecosystems throughout California under the state's Sustainable Groundwater Management Act framework.
"A vast majority of our planet's freshwater is groundwater, but we don't acknowledge or manage it sustainably, resulting in serious consequences for humans and natural ecosystems," said Dr. Rohde, now Principal at Rohde Environmental Consulting, LLC. "Groundwater is critical for many ecosystems, but their water requirements are rarely accounted for by water agencies and conservationists. To reconcile that, our study provides a simple and practical approach to detect ecological thresholds and targets that can be used by practitioners to allocate and manage water resources."
Utilizing 38 years of Landsat satellite images (1985-2022) and statewide groundwater well data, the study examined impacts on key plant communities. A major challenge was to develop standardized metrics that can be applied across diverse ecosystems with site-specific water conditions. The team applied a common data transformation method in a new way to identify thresholds of vegetation greenness and groundwater depth over time that can determine groundwater needs for ecosystems, helping to inform decisions about water use and planning.
"Groundwater-dependent ecosystems such as wetlands, floodplains, and riparian zones have a very outsized importance on biodiversity. Upwards of 80 to 90 percent of species in a general region may be dependent on these ecosystems in some form or another," said Dr. Stella, study co-author and Vice President for Research at ESF. "We applied a simple statistical approach to very large data sets to identify warning signs and conservation targets for a great diversity of ecosystem types."
The vast geographic scope and long timeline covered by the study, allowed the team to evaluate how large-scale systems respond to major climate shocks such as the historical California drought that occurred from 2012 -- 2016, as well as where individual groundwater-dependent ecosystems can serve as resilient drought refugia.
"This type of study, covering the entire state of California over close to 40 years, has really only become possible in the past few years and shows the promise for similar studies over a much larger geographic area using the approach pioneered by Dr. Rohde," remarked co-author, Dr. Dar Roberts from UCSB.
It was discovered that during drought events, groundwater-dependent vegetation that maintains a connection to groundwater can serve as critical drought refugia for associated species, such as riparian birds or fish. However, when groundwater levels deepen beyond plants' rooting zones during drought, these safe havens can be lost.
"A key takeaway from this study is that we can use what we know about how deep the roots of different types of plants tend to be to approximate what groundwater levels are needed to maintain ecosystem health," said co-author Dr. Christine Albano from DRI. "We found that vegetation was healthier where groundwater levels were within about 1 meter of maximum root depth, as compared to where groundwater was deeper."
The research team is hopeful that their approach and findings can help inform water management decisions in California and beyond.
"This study arms groundwater managers with an intuitive, site-specific measure that can provide a data-driven foundation to guide water allocation and ecosystem restoration efforts," said co-author and professor Kelly Caylor from UCSB.
"Globally, there are increasing efforts to manage groundwater resources for multiple purposes, not only to support drinking water needs or high-value agriculture. Our work provides a sound basis on which to develop clear guidelines for how to manage groundwater to support a wide range of needs within drainage basins in California and beyond," said co-author Prof. Michael Singer from Cardiff University.
- Ecology Research
- Endangered Plants
- Drought Research
- Marine conservation
- Groundwater
- Remediation
- Hydrogeology
- Eutrophication
Story Source:
Materials provided by SUNY College of Environmental Science and Forestry . Note: Content may be edited for style and length.
Journal Reference :
- Melissa M. Rohde, John C. Stella, Michael Bliss Singer, Dar A. Roberts, Kelly K. Caylor, Christine M. Albano. Establishing ecological thresholds and targets for groundwater management . Nature Water , 2024; DOI: 10.1038/s44221-024-00221-w
Cite This Page :
Explore More
- Ozone Removes Mating Barriers Between Fly ...
- Parkinson's: New Theory On Origins and Spread
- Clash of Stars Solves Stellar Mystery
- Secure Quantum Computing at Home
- Ocean Currents: Collapse of Antarctic Ice ...
- Pacific Cities Much Older Than Previously ...
- The Milky Way in Ancient Egyptian Mythology
- Physical Activity Best in the Evening
- How the Body Switches out of 'Fight' Mode
- New Drug Prevents Flu-Related Lung Damage
Trending Topics
Strange & offbeat.
DISCOVER THE INNOVATIVE WORK WE ARE DOING ON:
- Air, Food & Water
- Art & Culture
- Cities & Towns
- Climate Change
- Energy & Technology
- Environmental Justice
- Law & Policy
- Nature & Conservation
- Sustainable Business
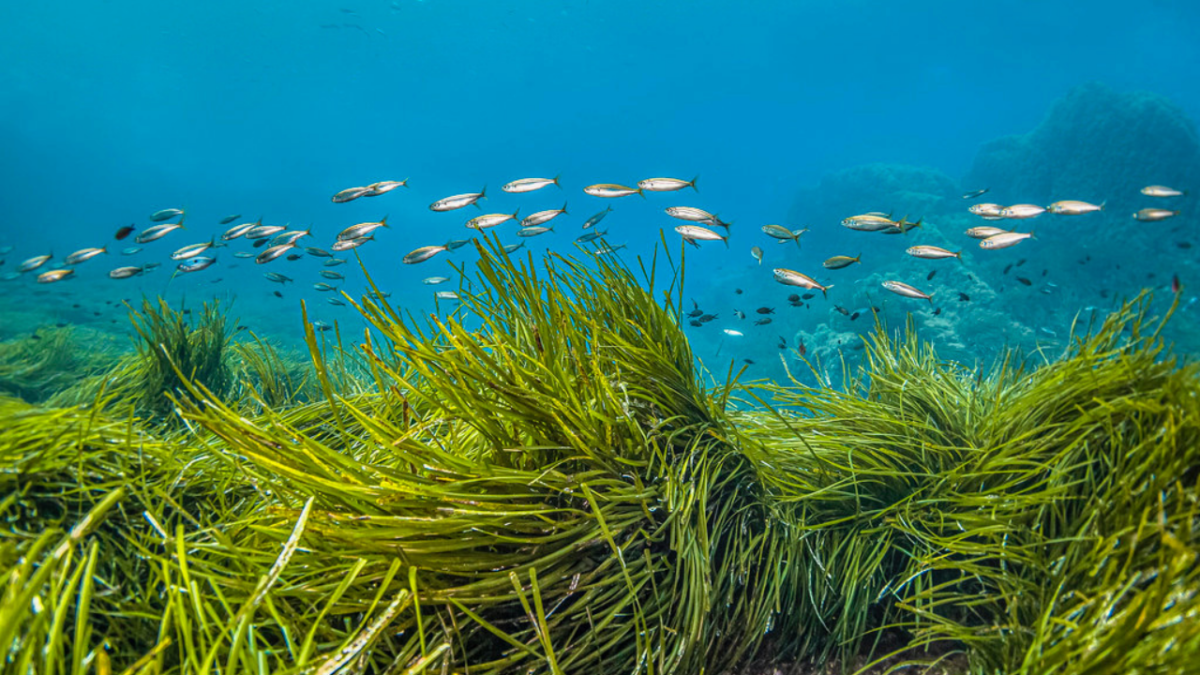
eDNA reveals unique fish communities in Southern California seagrass meadows
nature & conservation
Commonly mistaken for seaweed or kelp, seagrass is a type of marine flowering plant that grows in shallow waters.
The patches it forms are among the most productive ecosystems in the world. Fed by nutrient-rich currents, these underwater meadows support a staggering array of marine life.
A UCLA-led study published in the journal PLOS One uncovered distinct fish communities associated with different geographically located seagrass beds, and revealed the hidden biodiversity of these habitats.
“We saw that seagrass beds, even between coastal mainland and coastal island beds, support largely different communities of fish across the seasons,” said Tanner Waters, the paper’s lead author and a Ph.D. graduate from UCLA Institute of the Environment and Sustainability.
Researchers collected environmental DNA (eDNA) from seagrass sites across Southern California’s coast. Seagrass meadows in protected bays and inlets were found to host species that live in wetlands, estuaries and bays. In contrast, seagrass meadows along exposed coastlines — open to ocean waves — supported species that live in the water column and on rocky reefs.
The research identified 78 species of fish thriving in these seagrass habitats. The structural components formed by the shoots and leaves of the seagrass serve as a nursery for juvenile fish and shelter for various species. Commercially and recreationally important fish species, such as California halibut, kelp bass and barred sand bass, significantly benefit from these habitats as well.
Southern California’s coast is home to two types of seagrasses — surfgrass and eelgrass. They play a crucial role in cleaning surrounding water and sequestering carbon dioxide from the atmosphere. Since seagrass health is directly linked to the health of the entire coastal ecosystem, these underwater meadows serve as early warning signs of environmental problems. By monitoring seagrass health, scientists can gain valuable insights into the status of our coastal waters.
The use of eDNA — a technique that collects genetic material shed by organisms in their environment — allowed researchers to detect a greater variety of species than traditional visual fish sampling methods, including rare or ecologically important ones. While visual surveys provide valuable information on abundance and fish length, eDNA sampling is more comprehensive, revealing uncommon visitors such as tuna, which rarely inhabit shallow and nearshore environments.
While the study highlights how seagrass beds support a staggering array of marine life, it also underscores the need for tailored conservation strategies based on location, Waters said. “Future efforts must consider geographic diversity of seagrass beds in order to ensure the greatest net benefit for Southern California’s fish populations,” he said.
As Southern California’s oceans face escalating threats, including coastal development and climate change, safeguarding these vital habitats is critical to protecting biodiversity and fisheries. Recognizing the many species that visit or live in seagrass beds can help implement effective conservation strategies for these habitats.
MEET THE AUTHOR
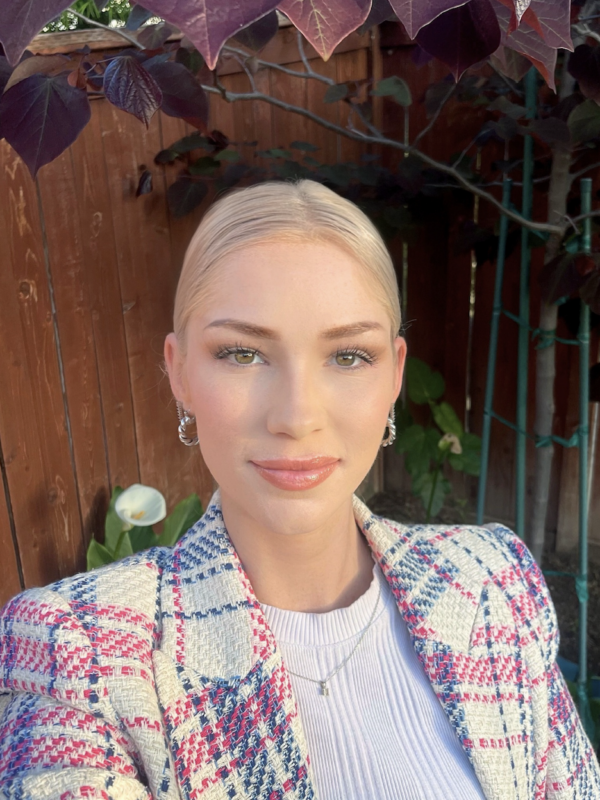
Claire Griffiths
RELATED ARTICLES
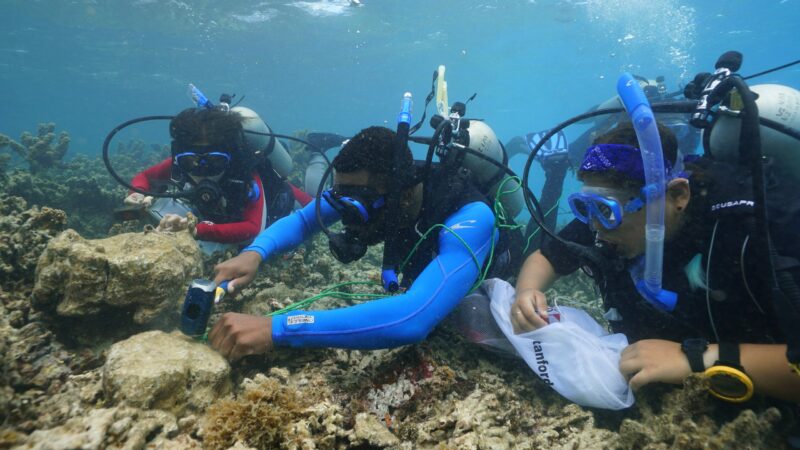
Sea urchins help tropical coral reefs recover, study finds
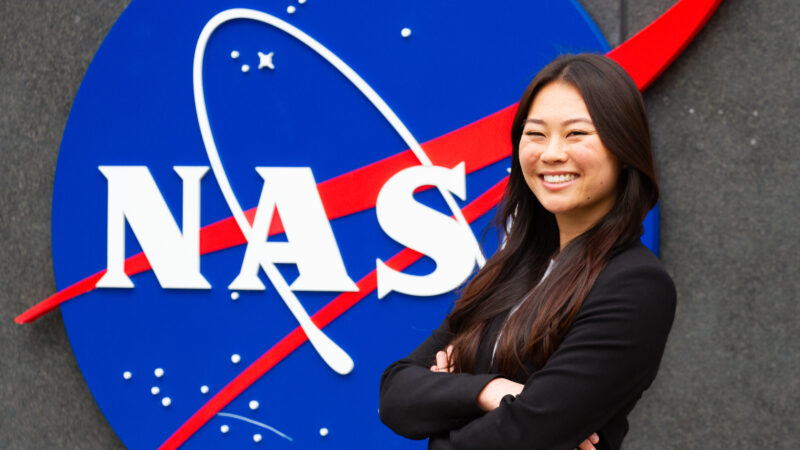
Alumni Spotlight — From UCLA to NASA, Danielle Sonobe ’23
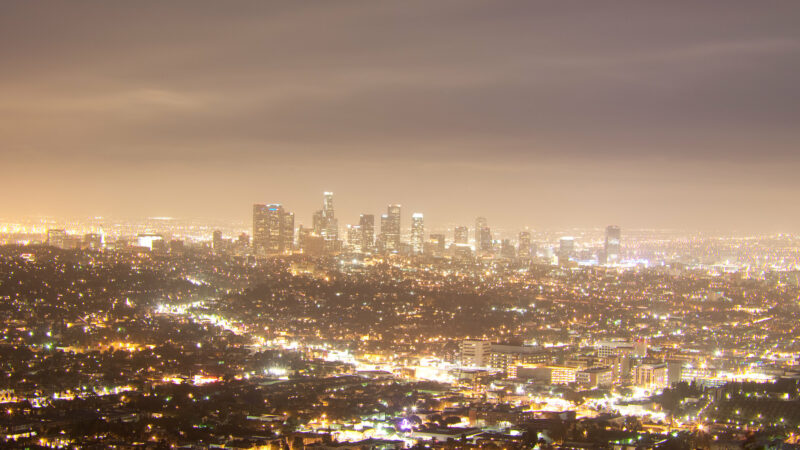
How to dim the consequences of global light pollution
Voice of the US farmer 2023–24: Farmers seek path to scale sustainably
Since 2018, McKinsey has gone into the field to better understand farmers’ mindsets. Following our publication of “Global Farmer Insights 2022,” 1 “ Global Farmer Insights 2022 ,” McKinsey, accessed March 29, 2024. we surveyed nearly 500 US farmers in 2023 to learn about their profitability, outlook on purchasing decisions, and adoption of sustainability practices and agtech solutions. 2 Survey conducted in fourth quarter 2023. There were 485 responses through computer-assisted web interviewing. Farmers surveyed included row- and specialty-crop operations with a farm-size distribution of 60 percent with less than 1,000 acres, 33 percent with 1,000 to 5,000 acres, and 7 percent with more than 5,000 acres. The 2023 survey found that despite recent supply chain disruptions, price volatility, and inflation, many farmers are investing in adopting sustainable-farming practices and technology solutions.
The sustainable-farming environment is rapidly evolving
Sustainable-farming practices are necessary to meet decarbonization goals and broader environmental targets. 3 The US Department of Agriculture (USDA) defines sustainable agriculture as “farming in such a way to protect the environment, aid and expand natural resources, and to make the best use of nonrenewable resources.” “Sustainability agriculture,” National Agricultural Library, accessed March 29, 2024. Agriculture accounts for nearly a quarter of global emissions, 4 Climate change 2022: Mitigation of climate change: Summary for policymakers , Intergovernmental Panel on Climate Change, 2022. and it was identified as the industry that contributes the most to exceeding planetary boundaries in McKinsey’s 2022 report Nature in the balance . 5 Nature in the balance: What companies can do to restore natural capital , McKinsey, December 5, 2022.
About the authors
Governments, investors, and companies are taking action to promote change. For example, the US Department of Agriculture’s mandatory budget in 2023 included an estimated $7 billion dedicated to conservation, with an additional $17 billion in conservation funding mandated by the Inflation Reduction Act through 2031. 6 “CBO’s February 2023 baseline for farm programs,” Congressional Budget Office, February 2023; FY 2024 budget summary , US Department of Agriculture, 2024. Furthermore, many agriculture players and consumer goods companies have committed to regenerative farming and deforestation-free supply chains.
However, average 2021–22 global finance flows for agriculture were only $43 billion, compared with $515 billion for energy systems and $336 billion for transport. 7 Barbara Buchner et al., “Global landscape of climate finance 2023,” Climate Policy Initiative, November 2, 2023. And adoption of sustainable-farming practices is not increasing fast enough to meet the sustainability goals of food processors and consumer goods companies.
In 2022, we released The agricultural transition: Building a sustainable future , 8 The agricultural transition: Building a sustainable future , McKinsey, June 27, 2023. a report that examined on-farm decarbonization measures and laid out a path to meet decarbonization targets. As a part of our fourth quarter 2023 survey, we explored a selection of these and other practices to better understand farmer adoption and opportunities to scale it in the United States.
Adoption of sustainable-farming practices is growing, but penetration remains low
Although 90 percent of farmers expressed awareness of the selected sustainable-farming practices, holistic adoption remains low. While more than 68 percent of farmers surveyed have adopted reduced- or no-till practices, only about half are using variable-rate fertilizer application and 35 percent are using controlled-irrigation practices.
Sustainable-farming practices that require behavioral changes in agriculture lead the way in adoption (for example, reducing or eliminating tillage), followed by practices that require product changes, such as nitrogen stabilizers or inhibitors. Practices that require changes in equipment tend to have the lowest adoption levels (Exhibit 1).
Many farmers are adopting sustainable-farming practices, but they are implementing them on only a small share of their acreage—generally less than 30 percent. There is an opportunity to increase acreage penetration among current users, especially for practices such as cover cropping and use of biologicals.
There are further opportunities to increase overall adoption by educating farmers on newer practices and convincing farmers who have heard of but not used the practices to give them a try. For example, 74 percent of farmers said they had heard of on-farm renewable energy, but only 13 percent have adopted it and only 7 percent are planning to adopt it in the next two years. Furthermore, 16 percent of farmers said they had not heard of biologicals, and 46 percent had not heard of biochar as a fertilizer.
Specialty-crop farmers are leading the way on adoption of most sustainability practices (Exhibit 2). Adoption is at least 20 percent greater among specialty-crop farmers for half of the practices surveyed. For example, biologicals adoption is 11 percentage points higher for specialty crops than for row crops; 20 percent of specialty farmers said they have adopted on-farm renewable-energy generation compared with 11 percent of row-crop farmers. Moreover, a greater percentage of specialty-crop farmers said they plan to implement sustainability practices in the next two years compared with row-crop farmers.
Practice adoption is correlated with perceived ROI
Farmers adopting sustainable-farming practices say they expect a positive ROI. Adoption of practices is highly correlated with farmers’ perceived ROI. Practices with the highest perceived ROI, such as applying fertilizer based on soil sampling, reducing or eliminating tillage, and implementing variable-rate fertilization, also have the highest rates of adoption (Exhibit 3).
Farmers expect many of the practices to have positive long-term benefits, such as a 3 to 5 percent yield rise and higher land value. However, farmers said they expect costs to remain 1 to 3 percent higher for most practices after more than five years of adoption. The ROI on adoption of sustainable-farming practices is complex and depends on a combination of factors including crop yield, crop prices, land value, and input, labor, and equipment costs. Although farmers said they generally expect the use of sustainable-farming practices to raise their costs, they also expect this increase to pay dividends in higher crop yield, land value appreciation, and better crop pricing.
For example, surveyed farmers who grow cover crops said they are experiencing or expect to experience a 3 percent increase in crop yield, a 2 percent increase in crop prices, and a 3 percent increase in land value on average. They are also experiencing or expect to experience a 2 percent increase in input, labor, and equipment costs on average, but they expect the benefits to outweigh these costs. Notably, for some practices, such as reducing or eliminating tillage, farmers expect both revenue increases (through increased yields) and cost reductions.
There are a variety of barriers to adoption
Small and large farms face different barriers in adopting sustainable-farming practices. However, compensation is a major obstacle to implementation for farms of all sizes (Exhibit 4). For example, 51 percent of medium-size and large farms with more than 1,000 acres and 39 percent of small farms identified obtaining a market premium (higher price) for sustainably grown crops or commodities as a top barrier to adoption. Moreover, 45 percent of medium-size and large farms see generating additional revenue from sustainability assets (for example, carbon credits) as their biggest barrier to adoption. Farmers with less than 1,000 acres are far more likely than those with bigger holdings to face challenges in implementing sustainable-farming practices (for example, operational barriers).
The most attractive incentives to unlock the transition to sustainable-farming practices vary by farm size (Exhibit 5). Representatives of medium-size and large farms with more than 1,000 acres said that certainty on operational benefits and reliable information on expected ROI are key. On the other hand, small farms with less than 1,000 acres highlighted the availability of financial incentives and assurance of a green premium (higher price) as the top two factors necessary to promote adoption. Overall, farmers are focused on gaining confidence in the economic benefits of sustainable-farming practices before making the full transition.
Across the board, both small and large farmers highlighted crop and commodity premiums from buyers of sustainable crops as the most attractive financial lever to increase adoption of sustainable-farming practices. Generating long-term and reliable sources of economic benefit may be key to driving additional adoption.
Government programs have seen greater participation than industry programs
Government-led programs designed to boost adoption of sustainable practices have substantially greater participation among farmers than industry programs do. These initiatives, such as the Environmental Quality Incentives Program (EQIP), are helping to spur adoption among farmers.
Of surveyed farmers, 57 percent said they were participating in a government program, while only 4 percent were participating in an industry-sponsored program, including the more than 15 carbon programs launched since 2016. 9 Lisa Moore et al., Agricultural carbon programs: From program chaos to systems change , American Farmland Trust, August 19, 2023. Adoption of sustainable-farming practices was at least 20 percent greater among government program participants than among those not enrolled in any program for ten of the 13 surveyed practices (Exhibit 6). This could indicate that government programs are driving adoption and that continued programmatic support from governments and industry players may encourage more farmers to transition to sustainable-farming practices.
Industry players can take steps to support farmers
As the importance of sustainable-farming grows, winning players will partner with farmers to support them in growth and innovation. To do so, industry participants could consider the following:
- reassessing the share of value captured by the farmer and bringing transparency to consumers about the “true cost of food”
- building sustainability programs and making them accessible; practice adoption is higher among participants in programs, but most of these are small scale today
- investing in education to help farmers overcome operational challenges and generate further data points on yield and cost benefits, especially for less-adopted practices
- collaborating with smaller and specialty-crop farmers in the near term, as these farmers are more willing to adopt most practices in the next two years
- continuing to evolve and grow nutrient-related programs, such as applying variable-rate fertilizer; most farmers view these practices as ROI positive, but only about half have adopted them and only in a part of their acreage
Many agriculture players are making commitments to sustainable farming in response to the sector’s large emissions footprint. But the transition to sustainably produced food depends on changing farmers’ behaviors and operational decisions. Our survey suggests that agriculture ecosystem players should consider how they can meet farmers’ expressed need for compensation for investments in sustainable-farming practices and provide reliable information about practice implementation and operational benefits.
David Fiocco is a senior partner in McKinsey’s Minneapolis office, Vasanth Ganesan is a partner in the New York office, Maria Garcia de la Serrana Lozano is an associate partner in the San Francisco office, and Julia Kalanik and Wilson Roen are consultants in the Chicago office.
The authors wish to thank Rui Chen, Raissa Dantas, Emma Galeucia, David Greenawalt, Emmanuel Mwaka, and Asha Sharma for their contributions to this article.
Explore a career with us
Related articles.
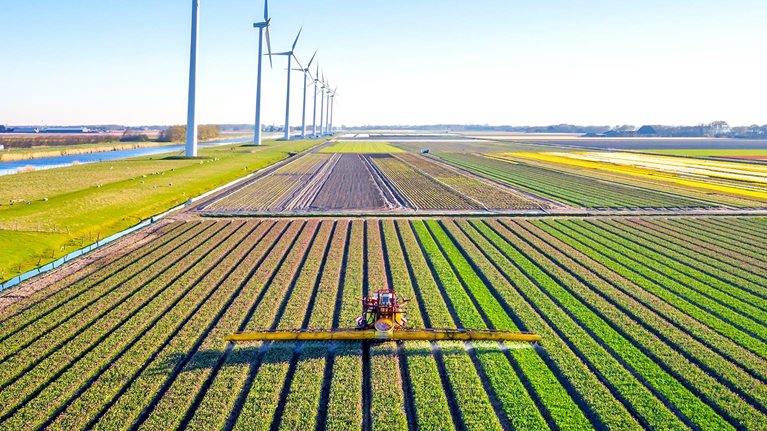
From green ammonia to lower-carbon foods

Building food and agriculture businesses for a green future
EDITORIAL article
This article is part of the research topic.
Women in Biogeochemical Dynamics Research: 2022
Editorial Frontiers in Environmental Science "Women in Biogeochemical Dynamics Research" Provisionally Accepted
- 1 Université de Reims Champagne-Ardenne, France
- 2 Institute of Marine Sciences, Spanish National Research Council (CSIC), Spain
The final, formatted version of the article will be published soon.
At present, less than 30% of researchers worldwide are women. Long-standing biases and gender stereotypes are discouraging girls and women away from science-related fields, and STEM research in particular. Science and gender equality are, however, essential to ensure sustainable development as highlighted by UNESCO. In order to change traditional mindsets, gender equality must be promoted, stereotypes defeated, and girls and women should be encouraged to pursue STEM careers.Therefore, we are proud to offer this platform in Frontiers in Environmental Science to promote the work of women scientists. To be considered for this collection, the first, last, or corresponding author should be a researcher who identifies as a woman The papers presented here highlight the diversity of research performed across the entire breadth of Biogeochemical Dynamics research and the advances in theory, experiment, and methodology with applications to compelling problems. Contributions to this journal showcase the breadth and depth of investigations aimed at understanding and mitigating human impacts on our planet.One significant study by Chua and Fulweiler (2023) highlights the importance of hightemporal-resolution gas concentration measurements in capturing the rapid response of sediments to low-oxygen conditions. This research sheds light on the dynamic nature of sedimentary processes and underscores the necessity of precise monitoring techniques in studying environmental phenomena.In another investigation, Forsyth et al. (2023) delve into the bioconcentration and translocation of rare earth elements in plants from legacy mine sites in Portugal. Their findings not only contribute to our understanding of plant-metal interactions but also have implications for environmental management strategies in areas affected by mining activities.Meanwhile, Ismail and Al-Shehhi (2023) offer a comprehensive review of biogeochemical models' applications in various marine environments. By synthesizing existing knowledge, the authors provide valuable insights into the role of these models in elucidating complex biogeochemical processes and guiding sustainable marine resource management practices. Lavergne et al. (2024) draw attention to the importance of conserving microorganisms in terrestrial ecosystems, focusing on soil microbial diversity in Chile and the Antarctic Peninsula. Their work underscores the need for heightened awareness and proactive conservation efforts to safeguard these essential components of ecosystem functioning.In a study with implications for land management practices, Rissanen et al. (2023) investigate the vegetation impacts on methane emissions from boreal forestry-drained peatlands. Their findings highlight the role of moss cover in modulating emissions and emphasize the importance of considering vegetation dynamics in peatland management strategies.Addressing the pervasive issue of plastic pollution in marine environments, Romera-Castillo et al. ( 2022) quantify the leaching of dissolved organic matter from aged plastic and its impact on microbial activity. This research underscores the urgent need to mitigate plastic pollution and its cascading effects on marine ecosystems. Furthermore, Santos et al. (2022) employ leaf wax biomarkers to reconstruct environmental conditions in a high-mountain lake area in western Iberia. Their work illustrates the utility of biomarker analysis in paleoenvironmental reconstructions and contributes to our understanding of past environmental changes. Sun et al. (2023) investigate the release of inorganic mercury and subsequent methylmercury production in boreal peatlands due to ground warming. Their findings underscore the complex interactions between climate change and mercury cycling in peatland ecosystems, highlighting the need for integrated approaches to mitigate mercury pollution.Lastly, Vila-Costa et al. (2023) data represent a significant step forward in unraveling the intricate interactions between pelagic marine bacteria and organic pollutants. By conducting comprehensive field studies in the tropical Pacific and subtropical Atlantic Oceans, the researchers provide invaluable insights into the responses of these bacteria to various pollutants.Collectively, these studies exemplify the diverse and interdisciplinary nature of research featured by women in Environmental Science. By advancing our understanding of environmental processes and informing evidence-based management strategies, these contributions play a crucial role in addressing the myriad challenges facing our planet. As we navigate the complexities of the Anthropocene, women scientists are at the forefront of fostering dialogue and innovation to promote environmental sustainability and stewardship. In the realm of biogeochemical dynamics research, women scientists have long been making significant contributions, yet their achievements and perspectives are sometimes overlooked or underrepresented. As we strive for inclusivity and diversity in the scientific community, it's imperative to shine a spotlight on the invaluable work of women researchers in this field. It's essential to amplify their voices, recognize their contributions, and support their career advancement. By fostering an inclusive and supportive environment that values diversity, we can harness the full potential of women scientists and unlock new insights into the complex dynamics of our planet's biogeochemical cycles. In conclusion, women scientists are driving innovation, discovery, and progress in biogeochemical dynamics research. Their expertise, leadership, and dedication are indispensable assets in tackling the environmental challenges of the 21st century. Let us continue to celebrate and uplift the contributions of women in science, paving the way for a more equitable and sustainable future.
Keywords: women in STEM, environmental science, Biogeochemical Dynamics Research, Gender Equality, Diversity in Science
Received: 09 Apr 2024; Accepted: 11 Apr 2024.
Copyright: © 2024 Cosio, Bravo and Sebastian. This is an open-access article distributed under the terms of the Creative Commons Attribution License (CC BY) . The use, distribution or reproduction in other forums is permitted, provided the original author(s) or licensor are credited and that the original publication in this journal is cited, in accordance with accepted academic practice. No use, distribution or reproduction is permitted which does not comply with these terms.
* Correspondence: Mx. Claudia Cosio, Université de Reims Champagne-Ardenne, Reims, France
People also looked at
Fellowships support student research on compostable cutlery, sustainable solutions, and shorebird conservation
Undergraduate fellowships in the College of Natural Resources and Environment allow students to design and pursue their own research projects.
- David Fleming
10 Apr 2024
- Share on Facebook
- Share on Twitter
- Copy address link to clipboard
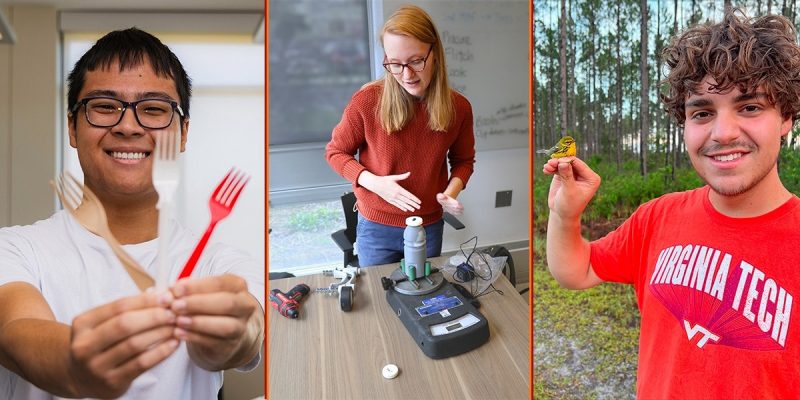
For Brian Tea, research starts at the back of Dining Services' Southgate Center. Lifting the lid of a container of food waste already starting to spoil, Tea scoops out enough to feed the composting tumblers that he is using to conduct his project, supported by an Undergraduate Research Fellowship from the College of Natural Resource and Environment (CNRE).
“My research is on compostable cutlery,” said Tea, a junior majoring in sustainable biomaterials in the Department of Sustainable Biomaterials . “I’m interested in understanding the life cycle of single-use materials and how sustainable options can provide a circular solution to waste.”
Tea tested the ability to compost cutlery made from three materials: the biodegradable polymer cellulose diacetate; polylactic acid, which is a renewable bioplastic; and the commonplace polystyrene plastics readily available at fast food restaurants.
Working under the guidance of Associate Professor Maren Roman , Tea measured the degradation processes of the various materials using an at-home composter that grinded and heated the composted materials to determine how efficiently the cutlery was able to break down.
Tea was one of eight recipients of a CNRE fellowship this past academic year. The program aims to give undergraduates both the financial resources and instructional support to conduct hands-on research.
“The CNRE Undergraduate Research Fellowship program provides students with an incredible opportunity to work side by side with our faculty to address local, state, national, and global challenges,” said Associate Dean Keith Goyne , who supervises the program. “Our undergraduate research fellows are tackling a wide range of topics in social and natural sciences, and they truly are using the fellowships to understand and improve the world.”
Applicants are eligible to receive up to $2,500 to support a research project of their own design with the sponsorship of a faculty mentor. Funding for fellowships comes in part from the generous gifts of donors to the college, whose contributions allow students the opportunity to take their education in their own hands. These grants are part of Virginia Tech Advantage , the university’s commitment to providing transformational educational opportunities to all students, regardless of income.
From materials science to making a difference
The Department of Sustainable Biomaterials focuses on training students to take on careers utilizing natural and synthetic materials to better the world.
For junior Justin Brandt, that challenge meant using one of the oldest building materials – bamboo – to enhance the use of cross-laminated timber (CLT), an engineered wood product that has become an increasingly popular material for the construction of homes and buildings.
“I think CLT is a genius building idea. It’s feasible and easy to implement, and it really blows my mind that it still isn’t used very often,” said Brandt, who switched majors on the recommendation of his roommate , junior Sage Smith. “One of the major problems is that since it is such a solid material, it’s difficult to hide wiring or piping inside of it.”
Brandt’s solution is to incorporate hollow lengths of bamboo in the solid wood timbers to provide builders with a handy, strong, and environmentally sustainable solution for hiding the plumbing and wiring that designers would prefer to have out of sight.
Brandt, who likes the challenge of tackling projects on his own, said he’s grateful to have had the chance to conduct his own research.
“I reached out to Daniel Hindman and told him my idea, and we sat down and talked about it,” Brandt said of connecting with the associate professor. “The whole experience made me feel very heard, and having the chance to express my passions in such an experiential and scientific way has been a great opportunity for me.”
Junior Rosa Williams’s dive into the subject of materials science will focus on everyday items: the small plastic bottles that hold medicine and the safety caps that keep children – and sometimes the elderly – from accessing the medicines inside.
Williams, who did a summer internship learning how plastic closure design can impact day-to-day-lives, said her curiosity about how we determine standards for products started much earlier.
“As a kid growing up, I was always super curious about who the specific person was who could open every peanut butter jar,” said Williams, who is majoring in packaging systems and design. “Those kinds of questions led to me thinking about how we make decisions to standardize certain things.”
Williams – a recipient of the Geza Ifju Scholarship and a scholarship from the Virginia Forestry Educational Foundation – will be conducting research on how to better understand packaging challenges as they relate to children and the elderly so that designers can make choices that allow for more user-friendly packaging options.
“There are testing requirements for child-resistant packages to be elderly-friendly, but those requirements exclude people with certain disabilities, including arthritis, which is extremely common for people over the age of 60,” said Williams, who is minoring in disability studies. “I want to do an exploratory study that considers the experiences of children and seniors to better understand the physical capabilities of both groups.”
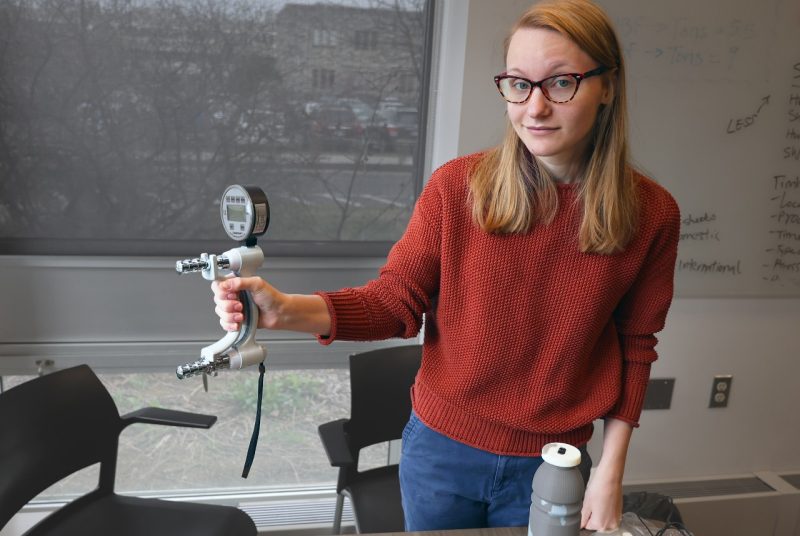
Using a hand dynamometer, which measures grip strength, and a torquemeter, to measure wrist twisting capabilities, Williams will compare the grip strengths of volunteer children and seniors against existing data to better understand the specific capacities of these two populations underrepresented in safety and accessibility measures for plastic bottles.
“My long-term career goal is to design packaging with an understanding of disabilities,” said Williams. “Improvements to plastic closures, with their vast uses across the food, beverage, and pharmaceutical industries, can make the world a more user-friendly place.”
Conservationists of a feather conference together
When Desraeli McBride showed up to present at the Wildlife Society’s annual conference in Louisville, Ketucky, this past fall, she could count on at least one familiar face: She and Max Nootbaar, both seniors majoring in wildlife conservation in the Department of Fish and Wildlife Conservation , were on hand to present research posters at the conference.
“The poster session was only two hours,” said McBride, who presented on her work with oystercatchers, a threatened bird species found along the barrier islands of Virginia. “But getting to stand there and feel like an expert with so many people coming by to ask about the research I was doing was honestly so much fun.”
McBride, a first-generation college student who graduated in December with degrees in wildlife conservation and biology, received a CNRE research fellowship to conduct her study on oystercatchers under the guidance of Professor Sarah Karpanty .
“I conducted research on Fisherman Island, which is one of the 14 barrier islands we have in Virginia,” said McBride. “I worked with American oystercatchers, which I think are the best birds in the world. For my project, I helped monitor nests and broods to determine the survival of chicks and investigate the overall habitat and cover selections of these birds.”
McBride, who aspires to be a wildlife field biologist, was the recipient of the Camp-Younts Foundation Scholarship in Wildlife. Funded by the Atkinson family, this scholarship supports the education of students studying animal conservation.
“I’ve been very supported in my time at the college, from the scholarships I’ve received, to the advising that is amazing here, to the general support from professors and students,” said McBride. “All of that has definitely made this place feel like home.”
For Nootbaar, an interest in piping plovers – those small shorebirds that race along ebbing waves hunting for food – started when he noted a small, numbered band on a bird he was watching.
“I wrote down the band number and reported the sighting, and I received a response that this individual hatched in Round Bay, Nova Scotia, in 2017,” said Nootbaar, a Charlottesville native who is graduating this spring. “I was thrilled by my small contribution to the research of this threatened species.”
That experience – and the encouragement of Logan Anderson ’22 – led Nootbaar to the Department of Fish and Wildlife Conservation, where he received an Undergraduate Student Research Fellowship to use breeding data to assess the demographic rates and population shifts of plovers, as part of a broader effort to model the species population dynamics along the Atlantic coast.
“I’m working with a data set from a banding study that was conducted on the Virginia barrier islands in 2018 and 2019,” said Nootbaar, who is also sponsored by Karpanty. “The program banded 111 adult and chicks, and several conservation organizations and wildlife agencies have been monitoring where those birds have ended up breeding over the past several years. I’m using that data to see how the Virginia population is connected to other populations in the mid-Atlantic region.”
Nootbaar, who was recently selected as the recipient of the college's David William Smith Leadership Award, also received a Dean’s International Study Abroad scholarship to travel to the Galápagos Islands for the spring semester study abroad course Darwin’s Galápagos: Evolution in the Anthropocene . He said his experiences in the college have motivated him to pursue a career in conservation research.
“My involvement in the Virginia Tech Shorebird Program has exposed me to the plight of shorebirds affected by climate change, habitat loss, and other anthropogenic drivers,” said Nootbaar. “Learning about these threats has strengthened my desire to conduct research relevant to the conservation and management of threatened species.”
From hands-on learners to future scientists
From improving everyday materials such as disposable cutlery and plastic pill bottles, to conserving species or designing new ways to imagine the future of housing, research fellowships are providing undergraduate students in the college with the chance to take the lead in directing their educational journeys.
“The experiential learning gained by our undergraduate research fellows is invaluable for their development as scientists,” said Goyne. “The opportunity to choose and craft their projects under the mentorship of globally-recognized experts provides students with great motivation to engage in the research and to complete the research with precision and accuracy.”
The application window for CNRE Undergraduate Research Fellowships for the 2024-25 academic year is open, and interested students in the college can learn more about the program and how to apply by visiting the undergraduate student research page . The deadline for applications is May 1.
Krista Timney
540-231-6157
- Blacksburg, Va.
- Career Development
- Climate Change
- Coastal Research
- College of Natural Resources and Environment
- Complex Problems
- Economical and Sustainable Materials
- Environment
- Experiential Learning
- Fellowships
- First Generation Students
- Fish and Wildlife Conservation
- Global Education
- Internships
- Outreach and International Affairs
- Philanthropy
- Research Participation
- Sustainability
- Threatened and Endangered Species
- Undergraduate Education
- Undergraduate Research
- Virginia Tech Advantage
- Young Alumni
Related Content
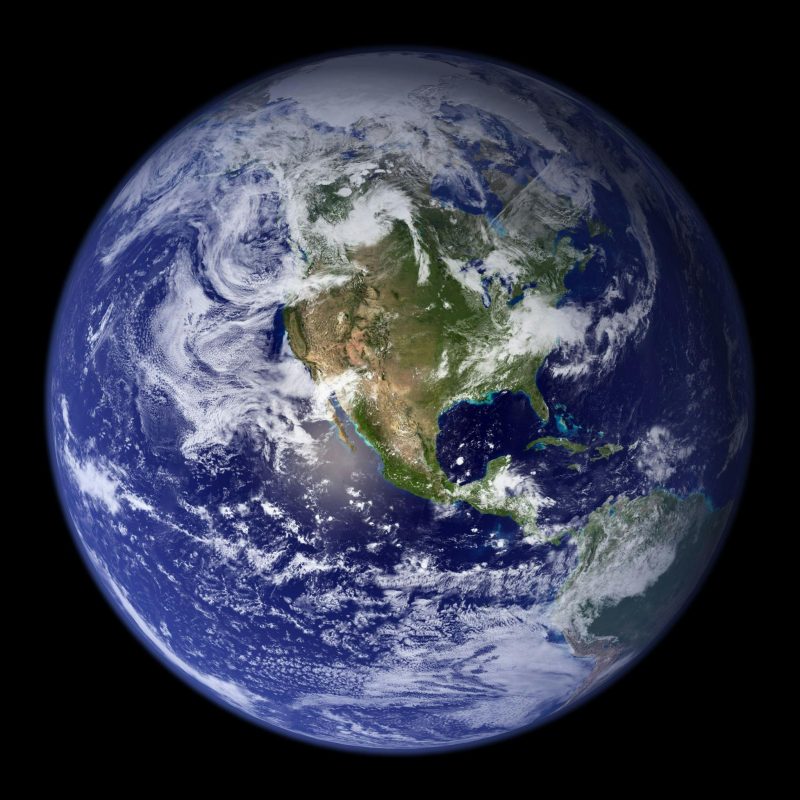
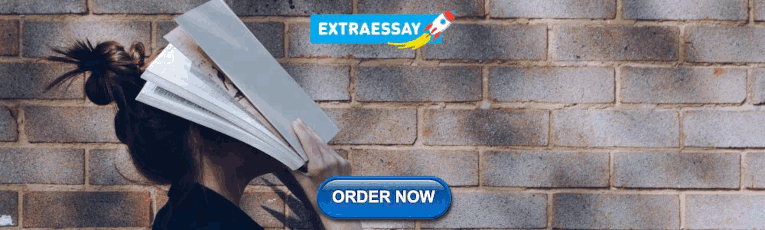
IMAGES
VIDEO
COMMENTS
7.2 Impact Factor. Current Opinion in Environmental Sustainability (COSUST) builds on Elsevier's reputation for excellence in scientific publishing and long-standing commitment to communicating high quality reproducible research. Established in 2010 as part of the Current Opinion and Research (CO+RE) suite of …. View full aims & scope.
The primary goal of Sustainable Environment Research (SER) is to publish high quality research articles associated with sustainable environmental science and technology and to contribute to improving environmental practice. The scope of SER includes issues of environmental science, technology, management and related fields, especially in ...
New research on environmental sustainability from Harvard Business School faculty on issues including the role of companies to mitigate climate change, corporate social responsibility, reporting to stakeholders, government relations and development of Chief Sustainability Officers.
Accordingly, we pursue three interrelated goals in this conceptual paper. After a brief review of key insights from extant theoretical and empirical research on the topic, our first conceptual contribution is to explain the process of leadership in the context of environmental sustainability and to define the construct of leader green behavior based on an integration of the behavioral approach ...
Current Research in Environmental Sustainability is a gold open access (OA) journal, which means articles are permanently and freely available. It is a companion to the highly regarded review journal Current Opinion in Environmental Sustainability (COSUST) and is part of the Current Opinion and Research (CO+RE) suite of journals. ...
Sustainability is essentially the ability to endure, for example by exploiting resources in a way that does not deplete their future availability or unduly damage the wider environment. This ...
S. Nikhil. R. S. Ajin. Original Article 17 January 2023 Pages: 59 - 72. 1. 2. …. 7. Next. Environmental Sustainability is a multi-disciplinary, quarterly, peer reviewed journal focused at maintaining and sustaining the quality of our planet through ...
Abstract. Environmental sustainability is key to allow for human development without compromising natural resources. Environmental indicators provide crucial information about the state of the ...
There is a compelling need to consider the environmental impacts of DDAI technologies. 17 This includes, for example, who is, and who should be responsible for these impacts and how these responsibilities should be enacted and distributed. Given that concern about environmental sustainability in the health research sector is relatively recent, 2 this paper provides a scoping review to explore ...
Indeed, given the breadth of the topic, it is difficult to find papers within the Journal of Environmental Planning and Management (JEPM) that do not contribute to sustainable development and sustainability. However, even after over thirty years of research, many papers in this Journal still regularly feature the terms "sustainable ...
Environmental sustainability touches many: community food systems, green space & urban tree canopy, and access to clean, drinkable water among them. Sometimes oversimplified to an issue of choice, the health status and lifestyle choices of community members are often a byproduct of the systems — political, economic, social — in which they ...
In this paper we argue the need to reimagine research ethics frameworks to include notions of environmental sustainability. While there have long been calls for health care ethics frameworks and decision-making to include aspects of sustainability, less attention has focused on how research ethics frameworks could address this. To do this, we first describe the traditional approach to research ...
The National Research Council Committee on Computing Research for Environmental and Societal Sustainability has suggested that "[s]marter energy grids, sustainable agriculture, and resilient infrastructure provide three concrete and important examples of the potential role of IT innovation and CS research in sustainability." ... However, the ...
Environmental sustainability is a significant research area at Microsoft. We are working on a wide portfolio of research projects that push the state of the art in science, engineering and economics to make Microsoft, our customers, and the world more sustainable. We are building on our long-standing relationships with the academic community ...
Sustainability Blog. Read our latest research, articles, and reports on Sustainability on the changes that matter most for the challenges and opportunities ahead.
Sustainability: A Comprehensive Literature. January 2016. DOI: 10.4018/978-1-4666-9639-6.ch015. In book: Handbook of Research on Global Supply Chain Management (pp.248-268) Chapter: 15. Publisher ...
Figure 1 summarises the environmental sustainability activities across operations, teaching and research areas. The scores achieved by each university are summarised in Fig. 2, expressed as a percentage of the maximum possible score from Table 2 (Operations = 32, Teaching = 6, Research = 7). The data showed similar levels of integration in Australia and in England, particularly in the ...
Global Research in Environment and Sustainability (GRES) is an open access and peer-reviewed international journal. Environmental sustainability has become an increasingly important topic in today's world as the impacts of climate change and human activities on the planet continue to grow. Research in this area focuses on identifying solutions ...
Abstract and Figures. This paper reviews the existing research on sustainability and sustainable development around the world. It begins by defining the sustainability and sustainable development ...
Groundbreaking research in groundwater's role in ecosystem sustainability. ScienceDaily . Retrieved April 8, 2024 from www.sciencedaily.com / releases / 2024 / 04 / 240403130524.htm
"We saw that seagrass beds, even between coastal mainland and coastal island beds, support largely different communities of fish across the seasons," said Tanner Waters, the paper's lead author and a Ph.D. graduate from UCLA Institute of the Environment and Sustainability.
Sustainable-farming practices are necessary to meet decarbonization goals and broader environmental targets. 3 The US Department of Agriculture (USDA) defines sustainable agriculture as "farming in such a way to protect the environment, aid and expand natural resources, and to make the best use of nonrenewable resources." "Sustainability ...
To address a rapidly changing space operating environment and ensure its preservation for generations to come, NASA released the first part of its integrated Space Sustainability Strategy, on Tuesday advancing the agency's role as a global leader on this crucial issue. "The release of this strategy marks true progress for NASA on space sustainability," said NASA Deputy Administrator Pam ...
At present, less than 30% of researchers worldwide are women. Long-standing biases and gender stereotypes are discouraging girls and women away from science-related fields, and STEM research in particular. Science and gender equality are, however, essential to ensure sustainable development as highlighted by UNESCO. In order to change traditional mindsets, gender equality must be promoted ...
Since the sustainable development theory was proposed, it has been applied by many scholars in ESG-related research . Social and environmental sustainability is highly compatible with the social and environmental pillar of ESG . In addition, enterprises can formulate ESG implementation strategies through internal governance mechanisms to deal ...
For Brian Tea, research starts at the back of Dining Services' Southgate Center. Lifting the lid of a container of food waste already starting to spoil, Tea scoops out enough to feed the composting tumblers that he is using to conduct his project, supported by an Undergraduate Research Fellowship from the College of Natural Resource and Environment (CNRE).