Colorectal Cancer Research Results and Study Updates
See Advances in Colorectal Cancer Research for an overview of recent findings and progress, plus ongoing projects supported by NCI.
Results from a new study suggest that the presence of circulating tumor DNA (ctDNA) in blood samples can predict which patients with colorectal cancer should and shouldn’t get chemotherapy after surgery to remove their tumors.
The ENLACE study is the first to use cutting-edge technologies to describe the molecular features of colorectal tumors in Hispanic and Latino people. The study’s goals are to improve treatments and increase Hispanic/Latino engagement in cancer research.
Using computer-aided detection (CAD) during a colonoscopy doesn’t help doctors find the growths most likely to become colorectal cancer, two studies find. Researchers agreed that CAD, which is aided by artificial intelligence technology, needs further refinement.
Radiation may not be needed for people undergoing surgery for rectal cancer, a large clinical trial has shown. A combination of two chemotherapy drugs before surgery appears to be as effective as chemo and radiation and may spare patients from long-term side effects.
When colorectal cancer spreads to the liver, it can be very difficult to treat. Cancer is more likely to invade the liver when patients have fatty liver disease. A recent study places the blame on “message bubbles” called extracellular vesicles that are released by the liver.
Researchers have identified four warning signs that they believe may help identify colorectal cancer early in younger adults. The signs or symptoms are abdominal pain, rectal bleeding, diarrhea, and iron deficiency anemia.
A new treatment regimen may help improve the survival of some people with advanced colorectal cancer, according to results from an international clinical trial. The new regimen includes bevacizumab (Avastin) and the combination of trifluridine and tipiracil (Lonsurf).
FDA approved tucatinib (Tukysa) with trastuzumab (Herceptin) to treat HER2-positive advanced colorectal cancer. The approval was based on the MOUNTAINEER trial, in which nearly 40% of participants’ tumors shrank after receiving the drug combination.
In a study in mice, researchers showed that BHB, a compound produced while eating a ketogenic diet, may slow or stop colorectal cancer from growing. More studies are needed, they warned, to see if BHB has similar effects in humans.
People who had a positive FIT result (signs of blood in the stool) but didn’t get a follow-up colonoscopy were twice as likely to die of colorectal cancer as those who did get a colonoscopy, a new study found.
Excess fructose can promote obesity and colorectal cancer, a new study shows. In mice, the study found that the sweetener, a component of table sugar and high-fructose corn syrup, increased how long normal and cancer cells in the intestines live.
Researchers have discovered a consistent pattern of DNA damage in colorectal tumors that may explain how a diet high in red and processed meat can help cause colorectal cancer.
Screening people for colorectal cancer after age 75 may be beneficial, a new study suggests. The findings provide helpful information for physicians to use in discussing screening choices with their older patients.
Combining text messaging with mailing people free at-home FIT kits helped increase screening for colorectal cancer among a predominantly Black population, a new study has found. It’s part of a larger effort to reduce disparities in cancer screening.
Diagnoses of colorectal cancer continue to increase in younger adults. In September 2020, more than 400 leading scientists and patient advocates participated in an NCI/NIEHS-sponsored symposium to identify research priorities that address important questions about this concerning trend.
The Food and Drug Administration has approved encorafenib (Braftovi) in combination with cetuximab (Erbitux) to treat adults with metastatic colorectal cancer whose tumors have a specific mutation in the BRAF gene, called V600E.
For people with colorectal cancer with a specific mutation in the BRAF gene, a treatment regimen of three targeted drugs can improve how long they live without increasing their risk of serious side effects, results from a new clinical trial show.
Many colorectal cancers likely have spread long before the original tumor is detected, researchers have found. The findings suggest the need for very early detection and could help identify patients who need more aggressive systemic treatments.
Using novel proteogenomic techniques, scientists from NCI’s Clinical Proteomic Tumor Analysis Consortium have uncovered new features of colon cancer that may guide new treatment strategies.
Findings from an NCI-funded study suggest a new vaccine approach may have the potential to prevent colorectal cancer in people with Lynch syndrome, an inherited condition that increases an individual's risk for developing certain types of cancer.
The FDA has approved the combination of the immune checkpoint inhibitors ipilimumab (Yervoy) and nivolumab (Opdivo) for the treatment of patients with metastatic colorectal cancer whose tumor cells have defects that affect their ability to repair DNA.
Two studies examined the impact of more frequent follow-up testing for cancer recurrence in colorectal cancer survivors. Learn whether the studies showed that frequent testing improved survival.
Colorectal cancer screening reduces deaths from the disease, yet about one-third of Americans aren’t up to date with screening. Learn what happened when people waiting for routine checkups used an app that allowed them to order their own screening test.
People with FAP, an inherited condition that greatly increases their risk of gastrointestinal cancer, who took the drugs erlotinib (Tarceva) and sulindac (Aflodac) saw a substantial decrease in the number of precancerous lesions in the colon and rectum.
- U.S. Department of Health & Human Services

- Virtual Tour
- Staff Directory
- En Español
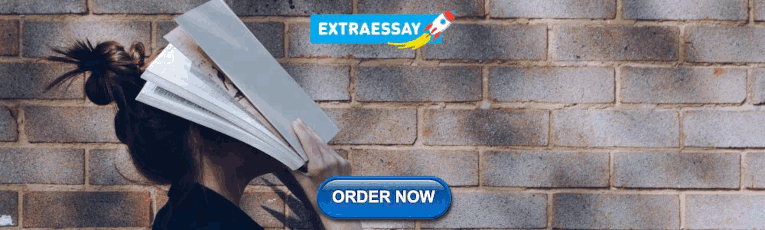
You are here
Research & training, advances in colorectal cancer research.

For an update on what medical science is doing to find colorectal cancer early and to treat it more effectively, read a Parade article by NIH Director Francis S. Collins, The Cancer You Can Beat .
Cancers of the colon and rectum, also known as colorectal cancers, are the third most commonly diagnosed cancers among men and women in the United States and the second leading cause of cancer death in this country. In 2010, it is estimated that more than 140,000 Americans will be diagnosed with colorectal cancer and more than 50,000 will die of the disease. Over the past several decades, researchers have learned a lot about colorectal cancer, but much more research is needed to find ways to prevent the disease and to detect it earlier and treat it more effectively should it occur.
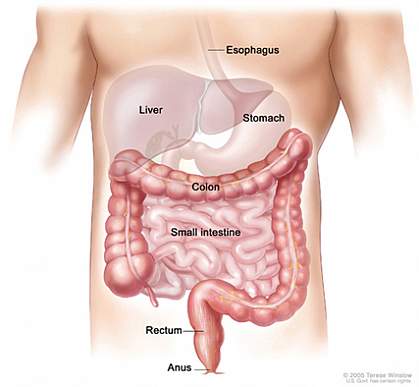
At the National Institutes of Health (NIH), the research effort on colorectal cancer is led by the National Cancer Institute (NCI). A vast network of NCI-supported scientists, located on the NIH campus in Bethesda, Md., and at research centers across the nation and around the world, are exploring new ways to prevent, detect, and treat colorectal cancer. Many other NIH institutes and centers, including the National Institute of Diabetes and Digestive and Kidney Diseases (NIDDK), the National Institute of General Medical Sciences (NIGMS), and the National Human Genome Research Institute (NHGRI), also support colorectal cancer-related research.

Both basic and clinical research scientists are utilizing the latest advances in technology in the effort to reduce the burden and toll of colorectal cancer. In the area of cancer screening and early detection, advances in computer-aided imaging, nanotechnology, and methods of molecular analysis promise to enhance our ability to identify abnormal growths in colorectal tissue that either are or could become cancerous. Meanwhile, other researchers are investigating ways to ensure that people adhere to current colorectal cancer screening recommendations.

In the area of cancer treatment, advances in DNA sequencing and genomic profiling methods should enable the identification of the specific molecular defects in a person’s cancer cells and permit the development of therapies that target or take advantage of those defects. This genetic knowledge might also be exploited to help identify people who have an increased risk of colorectal cancer and to develop effective interventions to prevent the disease.
Additional information about colorectal cancer
- Visit NCI’s colon and rectal cancer home page (also En Español )
- Call NCI’s Cancer Information Service at 1-800-4-CANCER (1-800-422-6237)
- Order NCI publications online
This page last reviewed on August 20, 2015
Connect with Us
- More Social Media from NIH
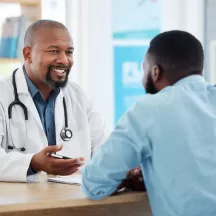
- Screening & prevention: why, when, how?
- Treatment: what are your options?
- Resources & Support
- Research: our efforts to end colon and rectal cancers
- Get involved: help us end colorectal cancer
You don't have to do this alone. Call our toll-free helpline: (877) 422-2030
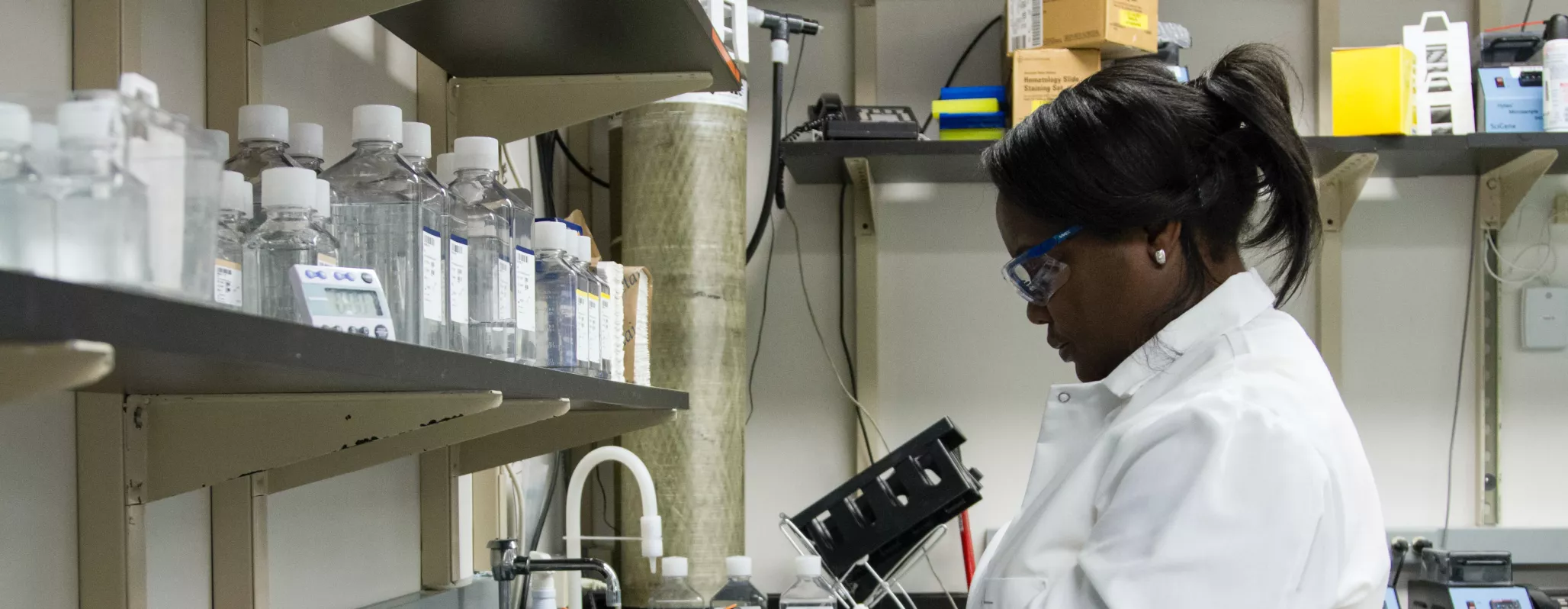
The Alliance’s mission is to end colorectal cancer in our lifetime. By supporting innovative colorectal cancer research by top doctors and scientists, we take important steps toward realizing our vision — a world free of this disease.
By the numbers
We've invested 1,000 hours gaining patient insights.
We've invested nearly $5 million in innovative research.
We work with 75 partner physicians and scientists.
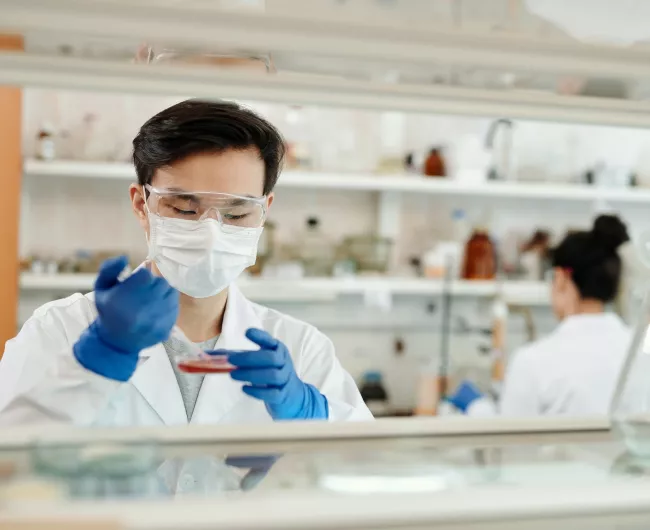
How we fund innovative colorectal cancer research
Our research program is a critical driving force in our mission to end colorectal cancer in our lifetime. We've invested nearly $5 million in colorectal cancer research, including nearly $3 million as early-stage grants to innovative researchers. Learn more about how our funding mechanisms guide our investments in colorectal cancer research.
Funding innovative colorectal cancer research
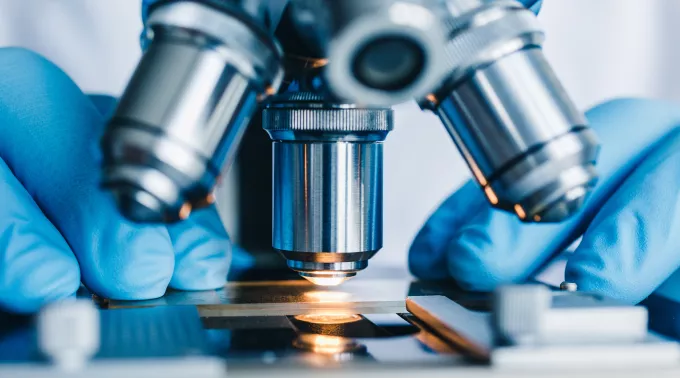
Projects we've funded
By funding the most innovative colorectal cancer research studies, we take important steps toward realizing our vision—a world free of colorectal cancer.
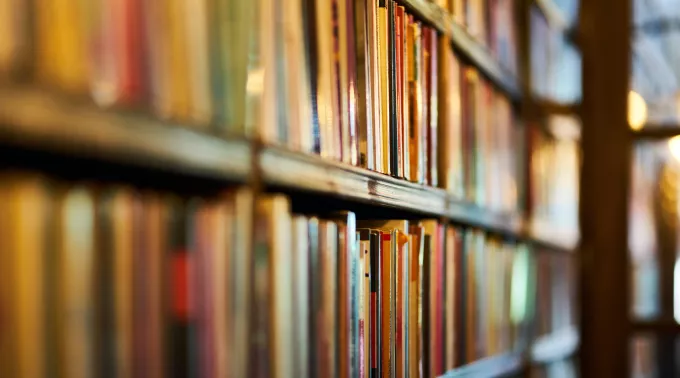
Research library
Our research library is your one-stop source to find Alliance-led progress reports, publications, presentations, and more based on our recent work in colorectal cancer research.
Providing leadership in colorectal cancer research
By convening scientific community leaders and patients together to advance and build knowledge, we are more efficiently addressing critical issues for patients’ direct benefit.
Bringing the Patient Experience to Experts
As a patient-focused organization, the Alliance offers opportunities to connect researchers from across the scientific community with real-time patient insights. We believe in ensuring research studies, clinical trial approaches, and program development all center on the needs of the patient and their family.
Focusing the Conversation
As the largest and most impactful colorectal cancer advocacy community, a core activity of the Alliance’s research efforts center around convening all of the stakeholders who advance science, knowledge, and solutions, as well as those who are influencing community practices, guidance, and policy that improves the research environment. Convening ensures that we all operate collaboratively, enhance efficiency and minimize waste—ultimately getting us to a cure faster.
Our Five-Year Plan
A comprehensive white paper, " The State of the Science in Colorectal Cancer ," details the outcomes of the analysis and summit, along with our five-year scientific strategy.
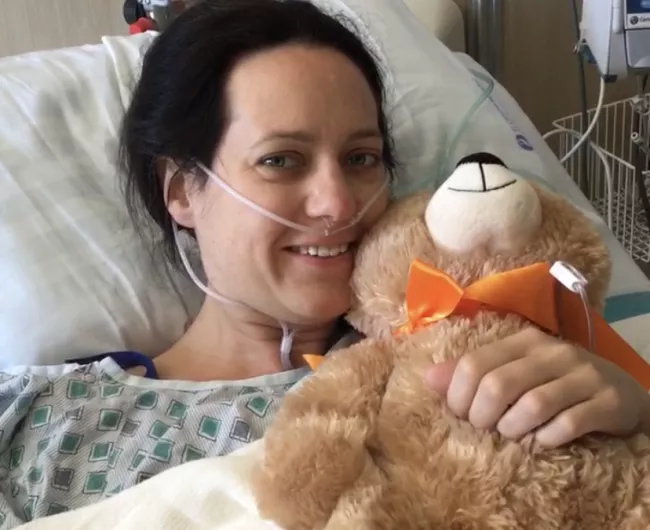
Support colorectal cancer research
Thanks to our generous supporters and the work of researchers all over the world, more survivors are beating colorectal cancer than ever before. However, we still have a long way to go. We need:
- Breakthroughs for advanced colorectal cancer
- Less toxic and more effective cancer treatments,
- Better techniques to diagnose and treat young onset colorectal cancer
- A better understanding of what motivates people to get screened earlier and detect or prevent this unique cancer.
Let’s work together to ensure that more families beat this cancer.
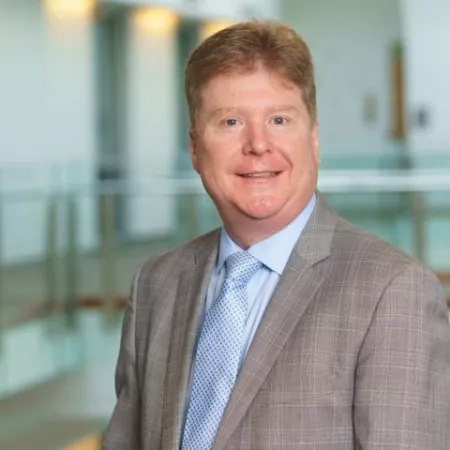
"Colorectal cancer is the second leading cause of cancer death in the U.S., with an increasing rise in early onset disease, or being diagnosed before the age of 50. To change the trajectory of this disease and improve patient outcomes, the Alliance is committed to funding research that leads to new treatments and improved therapies, novel methods in diagnostics, prognostics, and monitoring response to therapies, and studies focused on health equity in screening and care. The Alliance supports studies across the spectrum of basic, translational and clinical research as the promise of managing and curing colorectal cancer is within sight and research funding is desperately needed to realize our goal of eradicating colorectal cancer in our lifetime."
Related Topics Research
Top resources
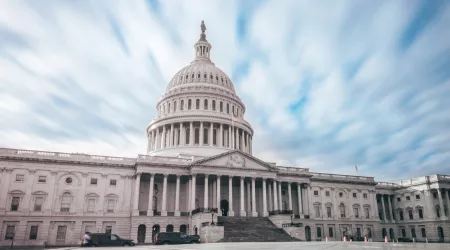
Alliance supports the Clinical Trials Modernization Act
This bipartisan legislation aims to improve participation in clinical trials by addressing major barriers such as non-medical expenses, trial location accessibility, diverse participation, and financial implications for patients.
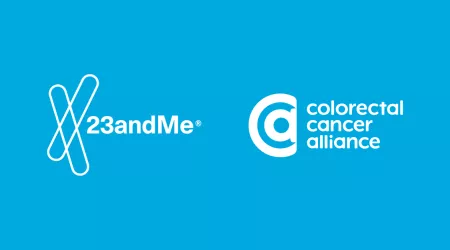
23andMe and the Alliance team up on cancer research
The collaboration aims to raise awareness, encourage screening, and help people better understand their risks.
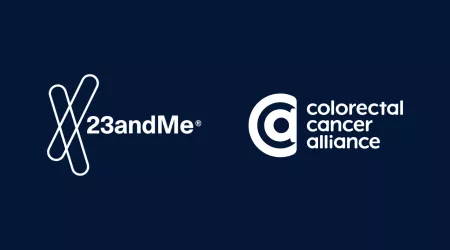
The Alliance and 23andMe Collaborate to Help Advance CRC Research in the Black Community
With 23andMe, the Alliance seeks another avenue to educate Black communities about the importance of knowing their family health history and risk factors to prevent colorectal cancer or find it at an early stage when it is most treatable.
Stanford Cancer Institute
Search stanford cancer institute.

Colorectal cancer (CRC) is the second leading cause of cancer deaths in the United States. The Stanford Cancer Institute has labeled it a priority cancer. In observance of Colorectal Cancer Awareness Month and to learn more about recent advancements in the treatment and knowledge of CRC, we talked to SCI members George A. Fisher Jr., MD , Colleen Haas chair in the Stanford School of Medicine, and Steven M. Corsello, MD , Stanford assistant professor of Medicine and, by courtesy, of Chemical and Systems Biology and principal investigator of the Corsello Laboratory .
Mismatch repair (MMR) deficient CRC
“There is now a proof of concept that the immune system can cure advanced disease,” says Fisher.
Stanford was part of the first trial demonstrating the superiority of immunotherapy to chemotherapy in CRC patients with MMR deficiency, also known as microsatellite instability, which is now the first-line treatment for these patients. Further supporting this, a Memorial Sloan Kettering Cancer Center study showed 37 complete clinical responses to immune checkpoint inhibitors in patients with newly diagnosed mismatch-repair deficient rectal cancer.
“This is remarkable because these are patients who otherwise may be treated with an aggressive combination of chemotherapy, radiation, and surgery,” Corsello says.
While only 5% of metastatic CRC is related to MMR deficiency, nearly 20% of patients with early stage (II or III) disease have MMR deficiency. Fisher says that the challenge is now to find a way to activate the immune system of patients who do not have MMR deficiency, which is a key focus of current research.
HER2-amplified CRC
The accelerated approval of the combination of tucatinib and trastuzumab for advanced HER2-amplified CRC is a notable advancement in treating CRC patients. The drugs work in tandem as an antibody and a small molecule kinase inhibitor against HER2. Corsello notes that screening CRC tumors for HER2 expression is important to identify these tumors..
HER2-positive tumors are present in 3-5% of CRC patients , but finding HER2 amplification can have great implications for patient care. The combination improves progression-free survival compared to chemotherapy with a 38% objective response rate and 12.4 months median duration of response.
Fisher notes that SCI member Edgar Engelman, MD , identified a strategy targeting HER2 with an antibody that could generate an immune response, which is now in clinical trials with SCI members Chris Chen, MD , and Mark Pegram, MD , with Bolt Therapeutics as a sponsor.
RAS mutations
Forty to 50% of CRC tumors harbor RAS mutations with KRAS being a well-established and key culprit oncogene across multiple tumor types. Recent drug discovery advances have enabled the specific inhibition of cancers with the G12C mutation, a point mutation that changes an amino acid from glycine to cysteine at position12 on the KRAS protein. Cysteine residues can react with certain drugs to form covalent adducts and inhibit protein function. These drugs first showed efficacy in non-small cell lung cancer with KRAS G12C mutations, and now researchers are seeing evidence of activity among other cancer types, including CRC, of which up to 3% have KRAS G12C mutations. Specifically, adagrasib has shown anti-tumor activity for CRC patients with the G12C mutation.
“It’s a compound that inhibits the mutant form of KRAS, so it’s highly selective,” Corsello explains.
Further, a recent study in the New England Journal of Medicine demonstrated that the combination of adagrasib and cetuximab, an epidermal growth factor receptor antibody, increased the response rate compared to adagrasib alone.
While the response rates are not as high as lung cancer, Corsello is optimistic about the potential of these medications.
“We’re hopeful that KRAS-targeted therapy will benefit colorectal cancer patients and that we’ll figure out how to use those medications and combinations to further improve the response rate.”
Fisher says that several companies have pipelines with drugs that target G12D and other more common alterations of the KRAS gene that occur in colorectal and pancreas cancers. “We are excited to bring these now KRAS inhibitor to clinical trials for our colorectal and pancreas patients”.
Identifying patients and strategies that could avoid aggressive therapies and surgeries
A challenge in treating CRC patients is identifying who will benefit from adjuvant therapy, which is chemotherapy after surgery, and who can be spared chemotherapy’s risk of toxicity. In the past, providers have relied on the tumor’s clinicopathologic characteristics alone to make this decision, but clinicians are increasingly using the presence of circulating tumor DNA after surgery to identify patients at highest risk who may merit additional treatment.
Circulating tumor DNA can also be used to monitor for recurrence, as tumor DNA may be detectable in a patient before cancer is observable by a CT scan. Clinical trials are now ongoing to see if circulating tumor DNA alone is informative in terms of when to start treatment.
Meta-analyses have also shown that certain colorectal cancer patients, such as those with early stage 3 colon cancer or patients who have a tumor but only one or two positive lymph nodes, may have similar outcomes if they are treated with three months of chemotherapy compared to the standard six months. Shorter exposure to chemotherapy drugs decreases the chance of side effects, such as the risk of sensory neuropathy associated with longer exposure to the chemotherapy drug oxaliplatin
Additionally, a current study being conducted by Stanford professor in radiation oncology Erqi Pollum is examining if a more intensive regimen of radiation and chemotherapy can achieve a complete clinical response potentially allowing patients to avoid an operation with life altering consequence and only undergo surveillance.
Prevention and early detection of colorectal cancer remain key
The US Preventive Services Task Force recommends screening for colorectal cancer in adults starting at age 45. Per the American College of Gastroenterology, earlier screening may also be recommended, especially for individuals with a history of colorectal cancer or advanced polyp in a first-degree relative. Colorectal cancer incidence has increased in younger adults, so close attention to lower gastrointestinal symptoms and low threshold for diagnostic testing is merited, even in younger patients.
Patients who have MMR deficiency are now universally tested to see if the deficiency is from an inherited gene to inform treatment and so that family members are aware of their risk. Lynch syndrome is a genetic defect associated with an increased risk of colorectal cancer, as well as endometrial, ovarian, pancreas, gastric, small intestine, and bile duct cancers. A third of patients with MMR deficiency have inherited Lynch syndrome. Fisher points to the importance of increasing awareness of Lynch syndrome and notes that it’s actually more common than the well-known BRCA mutation associated with breast cancer.
Additionally, tumor molecular profiling is of increasing importance in treatment selection for patients with advanced disease.
Avenues for future breakthroughs
Corsello notes there remains strong interest in combining immune checkpoint inhibitors with additional immunotherapies or targeted therapies to increase response rates in the most common form of colon cancer.
He also believes there are exciting opportunities to use new patient-derived models in cancer research.
“There are ways to take patient tumors and grow them directly in the lab by generating what are called organoids.”
The idea is to make personalized models from patient tumors and use them to understand cancer biology and perform drug discovery experiments. He points to SCI member Calvin Kuo, MD, PhD , as specializing in this field.
Fisher is hopeful about future advancements in colon cancer treatment.
“When I started working as a faculty member at Stanford, there was no treatment that had been proven to prolong survival in colon cancer. Now we know that not only can we improve survival, but in some patients, we can cure what used to be considered incurable.”
March 2023 - By Katie Shumake

- Patient Care
- Clinical Trials
- Health Equity
- Shared Resources
Stanford Medicine
Health care.
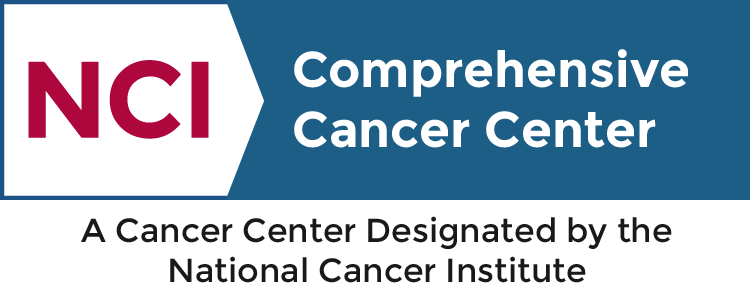
©2024 Stanford Medicine
Select "Patients / Caregivers / Public" or "Researchers / Professionals" to filter your results. To further refine your search, toggle appropriate sections on or off.
Home > Patients, Caregivers, and Advocates > About Cancer > Cancers > Colorectal Cancer
Colorectal Cancer
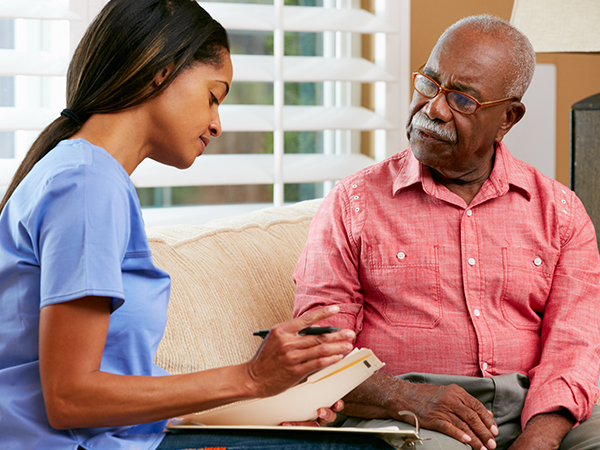
Colon cancer is cancer that forms in the tissues of the first part of the large intestine. Rectal cancer is a malignancy that develops in the rectum, which along with the anal canal, makes up the last 6- to 8-inch part of the large intestine. These cancers are often referred to together as colorectal cancer. Among the most common forms of cancer diagnosed in the United States, an estimated 152,810 people in the United States are expected to be diagnosed with these cancers and 53,010 are expected to die of these diseases in 2024, according to the National Cancer Institute .
Screening for colorectal cancer, the second leading cause of cancer death in the United States, by colonoscopy can both detect these cancers at an early stage, when successful treatment is more likely, and prevent them from developing in the first place, by detecting and removing precancerous polyps during colonoscopy.
If everyone followed colorectal cancer screening guidelines, at least 60 percent of U.S. colorectal cancer deaths could be avoided, according to a major study published in the New England Journal of Medicine in 2012. Unfortunately, it is estimated that one in every three U.S. adults is not getting screened as recommended, according to a U.S. Centers for Disease Control and Prevention analysis. Risk factors for colorectal cancer include family history, certain hereditary conditions, ulcers in the lining of the large intestine, and a personal history of polyps in the colon or rectum.
Source: National Cancer Institute
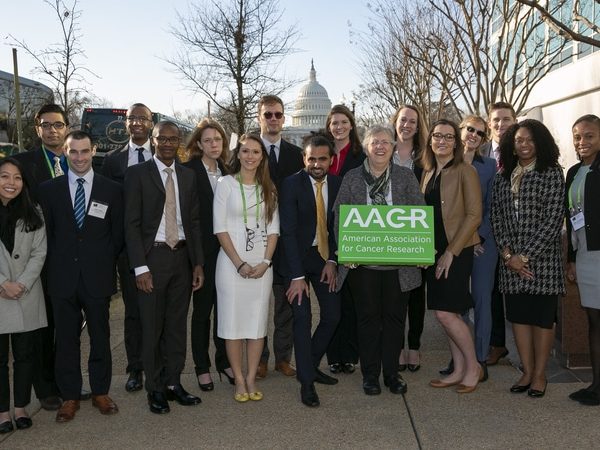
You can support the AACR's mission by contacting your representatives and senators and advocating for increased funding for lifesaving cancer research.

Editors’ Picks, August 2024: ‘Colon Age’ Screening Recs, Stress From Violence, and More
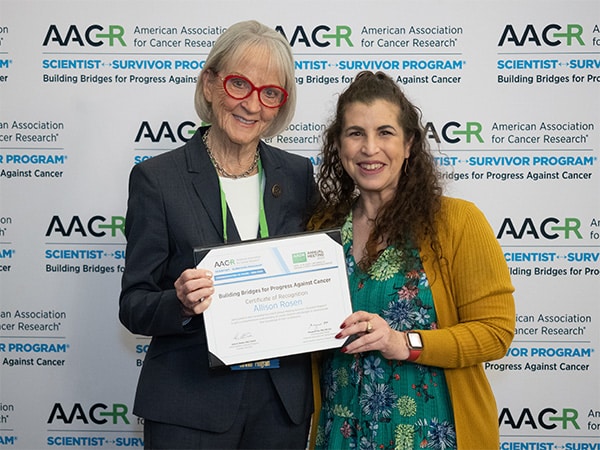
From an Early-Onset Cancer Diagnosis to Patient Advocacy
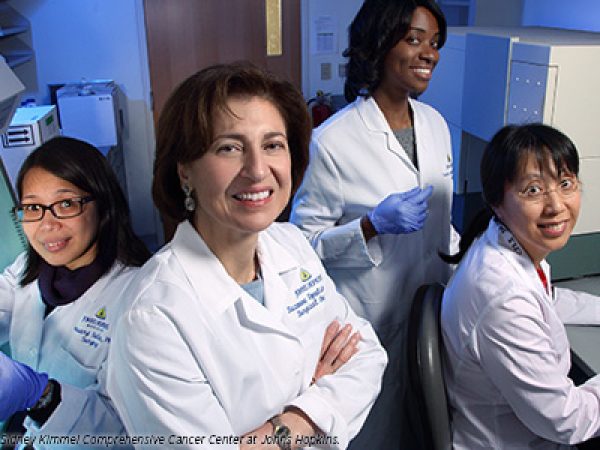
Developing Cancer Immunotherapy
- March is Colorectal Cancer Awareness Month
- Seeking Clues to Early-onset Colorectal Cancer
- Patients With Metastatic Colorectal Cancer May...

An official website of the United States government
The .gov means it’s official. Federal government websites often end in .gov or .mil. Before sharing sensitive information, make sure you’re on a federal government site.
The site is secure. The https:// ensures that you are connecting to the official website and that any information you provide is encrypted and transmitted securely.
- Publications
- Account settings
- My Bibliography
- Collections
- Citation manager
Save citation to file
Email citation, add to collections.
- Create a new collection
- Add to an existing collection
Add to My Bibliography
Your saved search, create a file for external citation management software, your rss feed.
- Search in PubMed
- Search in NLM Catalog
- Add to Search
The mechanisms and drug therapies of colorectal cancer and epigenetics: bibliometrics and visualized analysis
Affiliations.
- 1 School of Clinical Medicine, Chengdu University of TCM, Chengdu, China.
- 2 Hospital of Chengdu University of Traditional Chinese Medicine, Chengdu, China.
- PMID: 39268463
- PMCID: PMC11391208
- DOI: 10.3389/fphar.2024.1466156
Background: Numerous studies have demonstrated a link between epigenetics and CRC. However, there has been no systematic analysis or visualization of relevant publications using bibliometrics.
Methods: 839 publications obtained from the Web of Science Core (WoSCC) were systematically analyzed using CiteSpace and VOSviewer software.
Results: The results show that the countries, institutions, and authors with the most published articles are the United States, Harvard University, and Ogino and Shuji, respectively. SEPT9 is a blood test for the early detection of colorectal cancer. Vitamin D and gut microbiota mediate colorectal cancer and epigenetics, and probiotics may reduce colorectal cancer-related symptoms. We summarize the specific epigenetic mechanisms of CRC and the current existence and potential epigenetic drugs associated with these mechanisms. It is closely integrated with clinical practice, and the possible research directions and challenges in the future are proposed.
Conclusion: This study reviews the current research trends and hotspots in CRC and epigenetics, which can promote the development of this field and provide references for researchers in this field.
Keywords: bibliometrics; colorectal cancer; drug therapies; epigenetics; mechanisms.
Copyright © 2024 Tian and Chen.
PubMed Disclaimer
Conflict of interest statement
The authors declare that the research was conducted in the absence of any commercial or financial relationships that could be construed as a potential conflict of interest.
Document retrieval flow chart.
Published Trend Maps on CRC…
Published Trend Maps on CRC and epigenetics.
Number of publications by countries.
Intermediary centrality of countries.
Collaborative networks of countries.
Number of publications by institution.
Intermediary centrality of institutions.
Collaborative networks of institutions.
Number of publications by authors.
Collaborative networks of authors.
Cluster mapping of highly co-cited…
Cluster mapping of highly co-cited literature.
Keyword co-occurrence map of CRC…
Keyword co-occurrence map of CRC and epigenetics.
Keyword cluster map of CRC…
Keyword cluster map of CRC and epigenetics.
Keyword clustering timeline map of…
Keyword clustering timeline map of CRC and epigenetics.
Keyword brusts map for CRC…
Keyword brusts map for CRC and epigenetics.
- Advani S. M., Advani P. S., Brown D. W., DeSantis S. M., Korphaisarn K., VonVille H. M., et al. (2019). Global differences in the prevalence of the CpG island methylator phenotype of colorectal cancer. BMC Cancer 19 (1), 964. 10.1186/s12885-019-6144-9 - DOI - PMC - PubMed
- Bonasio R., Tu S., Reinberg D. (2010). Molecular signals of epigenetic states. Science 330 (6004), 612–616. 10.1126/science.1191078 - DOI - PMC - PubMed
- Bray F., Ferlay J., Soerjomataram I., Siegel R. L., Torre L. A., Jemal A. (2018). Global cancer statistics 2018: GLOBOCAN estimates of incidence and mortality worldwide for 36 cancers in 185 countries. CA Cancer J. Clin. 68 (6), 394–424. 10.3322/caac.21492 - DOI - PubMed
- Cancer Genome Atlas Network (2012). Comprehensive molecular characterization of human colon and rectal cancer. Nature 487 (7407), 330–337. 10.1038/nature11252 - DOI - PMC - PubMed
- Carding S., Verbeke K., Vipond D. T., Corfe B. M., Owen L. J. (2015). Dysbiosis of the gut microbiota in disease. Microb. Ecol. Health Dis. 26, 26191. 10.3402/mehd.v26.26191 - DOI - PMC - PubMed
Publication types
- Search in MeSH
Grants and funding
Linkout - more resources, full text sources.
- Frontiers Media SA
- PubMed Central
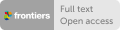
- Citation Manager
NCBI Literature Resources
MeSH PMC Bookshelf Disclaimer
The PubMed wordmark and PubMed logo are registered trademarks of the U.S. Department of Health and Human Services (HHS). Unauthorized use of these marks is strictly prohibited.
Thank you for visiting nature.com. You are using a browser version with limited support for CSS. To obtain the best experience, we recommend you use a more up to date browser (or turn off compatibility mode in Internet Explorer). In the meantime, to ensure continued support, we are displaying the site without styles and JavaScript.
- View all journals
- Explore content
- About the journal
- Publish with us
- Sign up for alerts
- Open access
- Published: 16 September 2024
Systematic prioritization of functional variants and effector genes underlying colorectal cancer risk
- Philip J. Law ORCID: orcid.org/0000-0001-9663-4611 1 ,
- James Studd ORCID: orcid.org/0000-0002-7157-754X 1 ,
- James Smith 1 ,
- Jayaram Vijayakrishnan ORCID: orcid.org/0000-0002-8284-2249 1 ,
- Bradley T. Harris ORCID: orcid.org/0000-0001-9585-1016 2 nAff3 ,
- Maria Mandelia ORCID: orcid.org/0000-0001-7571-6669 1 ,
- Charlie Mills ORCID: orcid.org/0000-0002-4755-7549 1 ,
- Malcolm G. Dunlop ORCID: orcid.org/0000-0002-3033-5851 2 &
- Richard S. Houlston ORCID: orcid.org/0000-0002-5268-0242 1
Nature Genetics ( 2024 ) Cite this article
7 Altmetric
Metrics details
- Colorectal cancer
- Functional genomics
Genome-wide association studies of colorectal cancer (CRC) have identified 170 autosomal risk loci. However, for most of these, the functional variants and their target genes are unknown. Here, we perform statistical fine-mapping incorporating tissue-specific epigenetic annotations and massively parallel reporter assays to systematically prioritize functional variants for each CRC risk locus. We identify plausible causal variants for the 170 risk loci, with a single variant for 40. We link these variants to 208 target genes by analyzing colon-specific quantitative trait loci and implementing the activity-by-contact model, which integrates epigenomic features and Micro-C data, to predict enhancer–gene connections. By deciphering CRC risk loci, we identify direct links between risk variants and target genes, providing further insight into the molecular basis of CRC susceptibility and highlighting potential pharmaceutical targets for prevention and treatment.
Similar content being viewed by others
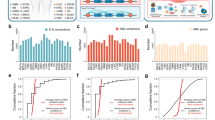
Genome-wide enhancer-gene regulatory maps link causal variants to target genes underlying human cancer risk
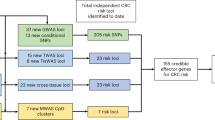
Deciphering colorectal cancer genetics through multi-omic analysis of 100,204 cases and 154,587 controls of European and east Asian ancestries
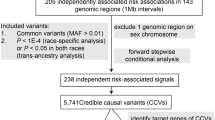
Fine-mapping analysis including over 254,000 East Asian and European descendants identifies 136 putative colorectal cancer susceptibility genes
CRC, which affects around 1.9 million people worldwide annually, has a strong heritable basis 1 . Our recent genome-wide association study 2 (GWAS) of 100,204 CRC cases and 154,587 controls has identified over 200 statistically significant independent risk loci. Deciphering the functional basis of these risk associations has the potential to provide biological insights into the etiology of CRC. However, deconvolution of GWAS risk loci has proven challenging owing to linkage disequilibrium between variants, and because most risk variants localize to noncoding regions of the genome, particularly within enhancer elements. Computational fine-mapping approaches can only predict putative causal variants based on linkage disequilibrium correlations 3 . To definitively identify variants with gene regulatory effects requires experimental validation.
Most noncoding GWAS risk variants are likely to function through cis -regulatory mechanisms that influence target gene expression. By investigating the transcriptional changes associated with different variants, it is possible to link specific alleles to changes in gene expression. Classical reporter assays can only assess the allelic transcriptional activity of individual variants. By contrast, massively parallel reporter assays (MPRAs) provide a scalable approach to characterize the regulatory effects of thousands of variants 4 , and this strategy has recently been successfully exploited in studies to implicate variants associated with multiple disease states 5 , including myeloma 6 and melanoma 7 , 8 .
Although advances in fine-mapping and functional annotation of risk loci have improved the nomination of causal variants, identifying target genes for GWAS signals remains a central challenge. Traditionally, variants have been assigned to the closest gene. However, solely relying on physical proximity for prediction can be unreliable, as causal variants are often regulatory and can affect gene expression through long-range interactions 9 , 10 . Furthermore, it is now recognized that enhancers can have more than one target gene 11 . The analysis of expression quantitative trait locus (eQTL) data generated across multiple cell types has undoubtedly greatly aided target gene identification. However, because published eQTLs capture only 9–13% of the GWAS heritability of cancers 12 , genomic data beyond gene transcription are required to comprehensively decipher the functional basis of associations 13 . Chromatin interactions and their proximity in genomic space are important for the regulation of gene expression. The integration of data from chromatin accessibility 14 , epigenomics histone ChIP-seq (chromatin immunoprecipitation followed by sequencing) 15 and three-dimensional (3D) chromatin interactions 16 , 17 , 18 has been shown to improve the ability to identify causal variants and their likely target gene. The recognition of the limitations of reliance on a single analysis to identify causal variants and gene targets underlying GWAS signals has led to the adoption of data integration approaches 7 , 8 . For example, the INQUISIT pipeline, which scores gene expression, chromatin interactions and ChIP–seq annotations, has frequently been adopted by breast cancer researchers to identify candidate gene targets 19 , 20 . More recently, the computational approach implemented in the activity-by-contact (ABC) model has sought to systematically link regulatory elements to target genes through the combination of enhancer activity with 3D chromatin contact frequencies 21 , 22 .
To provide insight into the functional basis of the CRC risk loci, we integrated data from multiple data modalities. First, we nominated causal variants at each of the risk loci through statistical fine-mapping incorporating tissue-specific epigenetic annotations, and by performing MPRAs in multiple colonic cell lines. Second, by generating and analyzing tissue-specific gene expression data and high-resolution chromatin interaction profiles, we linked nominated variants to target genes (Fig. 1 and Extended Data Fig. 1 ). Our analyses provide a detailed interpretation of CRC risk signals and their underlying basis.
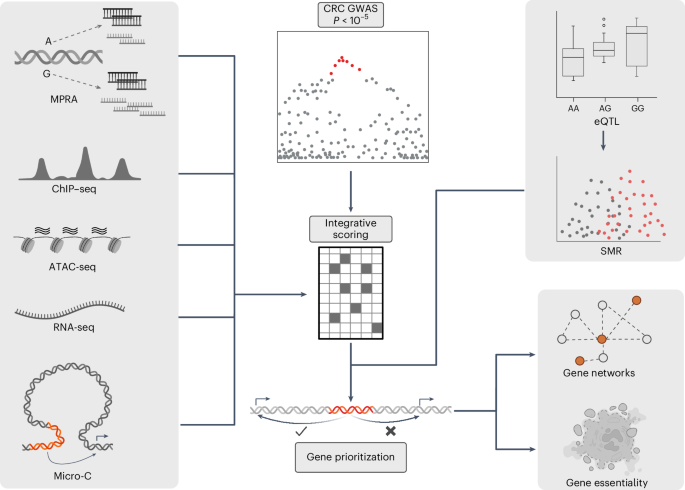
Using data from GWASs for CRC, we identified 170 regions of interest. Data from MPRAs, epigenetic marks (ChIP–seq), chromatin accessibility (ATAC-seq), gene expression (RNA-seq) and long-range chromatin interactions (Micro-C) were combined to derive an integrative score to prioritize the functional variants at each CRC risk locus. These variants were linked to target genes by analyzing colon-specific eQTLs and using SMR. In the GWAS plot, the coloured dots indicate the variants that are above the P value threshold. In the SMR plot, they represent the two different datasets (GWAS and eQTL). The coloured portions of DNA represent the genomic regions of interest that were studied.
Cell specificity and chromatin landscape at risk loci
To identify the cellular contexts of the CRC loci, we analyzed single-cell RNA sequencing (scRNA-seq) profiles across 24 different tissues using the Tabula Sapiens dataset 23 , as well as 11 intestinal regions in the Gut Cell Atlas 24 . We derived single-cell disease relevance scores (scDRSs), which link the scRNA-seq data with polygenic disease risk at single-cell resolution. This score assesses cell-type-specific expression for genes implicated by the GWAS association statistics. Genes whose expression was correlated with scDRSs were strongly enriched in large intestine and epithelial tissue ( P < 10 − 7 ). A specific analysis of intestinal cells showed a strong association of risk variants with BEST4 + enterocytes and colonic epithelial cells ( P < 10 − 7 , Supplementary Fig. 1 ). GWAS variants are generally thought to influence risk through cis -regulatory mechanisms affecting tissue-specific gene expression. We confirmed significant enrichment of enhancer- and promoter-associated histone marks, including histone H3 lysine 4 trimethylation (H3K4me3), H3 lysine 4 monomethylation (H3K4me1) and H3 lysine 27 acetylation (H3K27ac) in colonic and rectal mucosa cells using ChIP–seq data from the National Institutes of Health (NIH) Roadmap Epigenomics Project 25 ( P < 10 − 5 , Supplementary Fig. 2 ).
Statistical fine-mapping of risk loci
We fine-mapped each of the risk loci, including independent signals, incorporating functional annotation using PolyFun 26 and susieR 27 in conjunction with ChIP–seq data on H3K4me1, H3K4me3, H3K27ac, H3K27me3, H3K36me3 and CCCTC-binding factor (CTCF) marks, as well as assay for transposase-accessible chromatin with sequencing (ATAC-seq) data generated from six CRC cell lines (C32, CL11, HT29, SW403, SW480 and SW948) (Supplementary Table 1 ). For each independent risk locus, we extracted variants within a 1-Mb window and calculated the causal probabilities nonparametrically using the established PolyFun protocol, which estimates the per-single nucleotide polymorphism (SNP) heritability weighted by their functional annotations. Credible sets of causal variants were identified by susieR using the probabilities calculated by PolyFun. Posterior inclusion probabilities (PIPs) were ranked, and variants were added to the set until the cumulative PIP reached a value of >0.95, with a minimum individual variant PIP of 0.001. We identified 1–14 credible sets per locus (median, 1), consisting of 1–226 variants (median, 1) (Supplementary Table 2 ).
Functional significance of risk variants
We next assessed the regulatory activity of variants at each of the risk loci using a complementary experimental approach. At each GWAS locus (defined by a 500-kb window spanning the lead variant), we initially identified all variants with a P value within three orders of magnitude of the P value of the lead variant. As this may exclude potentially functional variants at loci where the lead variant has an especially strong association, we also included variants with −log 10 ( P variant ) > 0.7 × (−log 10 ( P lead variant )), stipulating an r 2 of >0.2 for the lead variant and a P variant of <10 − 5 . We performed MPRAs to simultaneously identify functional cis -regulatory variants, testing 8,880 variants (median of 39 variants per locus).
We evaluated the enhancer activity of reference and alternative alleles of the variants by cloning the surrounding 200 bp of genomic sequences. To test variant function in cellular constructs representing tumor and normal states, we transfected primary CRC cell lines (HT29 and SW403) and an immortalized primary colonic cell line (HCEC-1CT). Enhancer activity was quantified by sequencing barcodes in input DNA and mRNA (cDNA). Sequencing statistics and details of the quality control process are shown in Supplementary Table 3 and Supplementary Fig. 3 . A total of 275 unique variants displayed significant allelic transcriptional activity (false discovery rate (FDR) < 10 − 3 ; n = 133 in HT29, n = 102 in SW403 and n = 143 in HCEC-1CT; Supplementary Table 4 ). These ‘MPRA-significant’ variants were more likely to be fine-mapped as the causal variant (chi-square test, P = 4.39 × 10 − 3 ) as well as fine-mapped to enhancer and promoter regions of the colonic epigenome ( P = 3.66 × 10 − 18 ).
We focused on the underlying biological mechanisms through which genetic variants at CRC risk loci shape the regulatory environment around putative target genes. First, because risk variants can mediate their effects through altered transcription factor binding, we assessed transcription factor binding in chromatin-accessible regions using the JASPAR 2022 transcription factor motif database 28 in concert with the ATAC-seq data. The most common transcription factors predicted to bind at the loci included ZNF460 (found at ten loci), CTCF ( n = 7), PRDM9 ( n = 7), SP1 ( n = 7) and KLF5 ( n = 3), and these transcription factor binding sites were enriched at the GWAS loci ( P < 10 − 4 , Supplementary Fig. 4 ). Of note is KLF5, which was associated with the 13q22.1 risk locus, and PRDM9, a histone methyltransferase, which catalyzes H3K4 methylation. Second, we predicted enhancer–gene connections across risk loci from ultra-high-resolution Micro-C chromatin interaction profiles generated in CL11, HT29, SW403, SW403 and SW498 cell lines. The MPRA-significant variants preferentially localized to open chromatin ( P = 7.32 × 10 − 35 ) and mapped to regions that interacted with the transcription start site (TSS) of genes through a Micro-C chromatin interaction ( P = 7.28 × 10 − 4 ). In addition to confirming the interaction between rs6983267 at the 8q24.21 locus and the MYC TSS 18 , 29 , 30 , chromatin looping interactions implicate several other genes with established roles in CRC biology, including LAMC1 , TGFB1 and KLF5 . Using Akita 31 , a convolutional neural network based model for predicting 3D chromatin structure, 20% (1,798 out of 8,880) of the tested variants were predicted to affect 3D genome folding; 244 variants mapped to a CTCF motif, and approximately half of these ( n = 121) were predicted to severely affect the 3D chromatin structure.
Nominating causal variants using an integrative scoring system
To prioritize plausible causal variants at each locus, we integrated the multiple levels of functional annotations and fine-mapping data for all 8,880 variants. We adopted a scoring approach similar to that of ref. 8 , assigning a value between 0 and 2 for each variant and each annotation: 0 represented no hit, 1 represented a hit and 2 represented a strong hit (see Methods for the designation of each annotation-specific score). For each locus, the annotation scores were summed, and the variants ranked. The variants with scores in the top 20% were designated as Tier 1 variants, those with scores in the bottom 50% as Tier 3, and the remainder as Tier 2 (Fig. 2 ). We identified 2,406 Tier 1 variants, 42 of which were also the top hit in the GWAS meta-analysis. Forty-nine of the GWAS loci did not have any Tier 1 variants, and 16 of these also did not have any Tier 2 SNPs, with the 16 corresponding to regions with little to no functional data (Supplementary Fig. 5 and Supplementary Table 2 ).
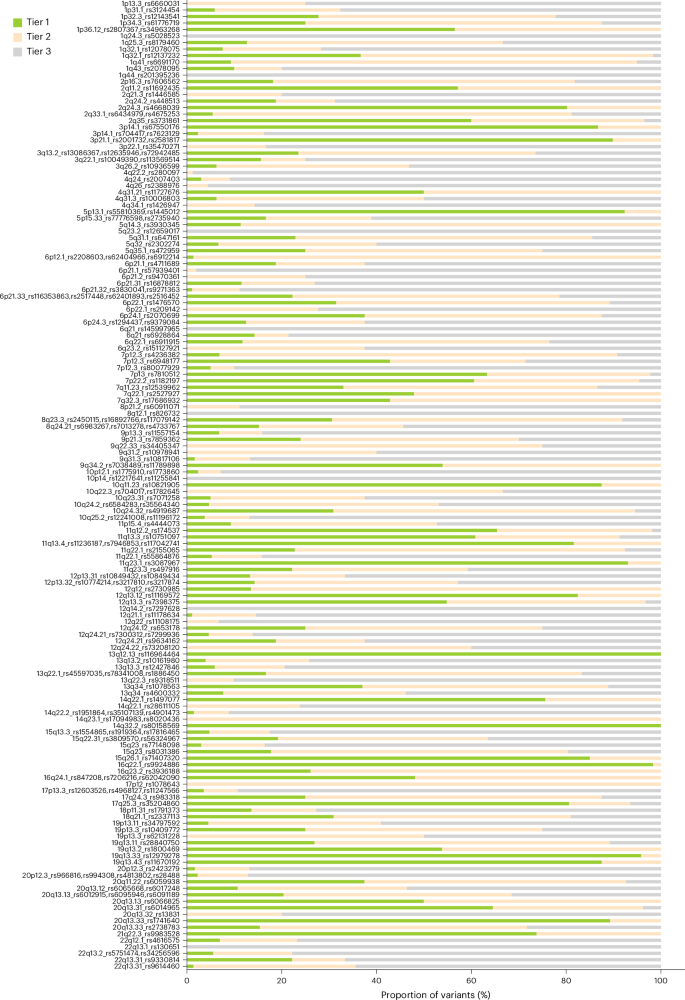
Scores were calculated as the sum of the annotations for each variant. Loci are labeled with the cytoband and the top GWAS SNPs in each region. The variants with scores in the top 20% were designated as Tier 1 variants, those with scores in the bottom 50% as Tier 3 and the remainder as Tier 2.
Source data
Linking nominated variants to target genes.
To link variants at each locus to respective target susceptibility genes, we analyzed eQTL data from normal colon (SOCCS (Study of Colorectal Cancer in Scotland) colon or rectum epithelium, n = 213; Genotype-Tissue Expression (GTEx) transverse colonic mucosa, n = 367) and CRC tissues (The Cancer Genome Atlas Colon Adenocarcinoma (TCGA COAD), n = 286; Rectum Adenocarcinoma (READ), n = 94). Of the 275 MPRA-significant variants, 113 had a significant eQTL ( P eQTL < 7.51 × 10 −5 ; Bonferroni-corrected P value for the 665 unique genes tested in the eQTL analysis), and 79 of these displayed a consistent direction of effect between MPRAs and eQTLs (that is, a direction of gene expression that is concordant with MPRA-allelic transcriptional levels) (Supplementary Table 2 ). By performing a summary-data-based Mendelian randomization (SMR) analysis 32 , we identified 94 candidate target genes for 54 risk loci ( P SMR-adjusted < 0.05; a median of one gene per locus) in the normal data, and 14 candidate target genes for 12 of the risk loci in the tumor data (Supplementary Table 5 ).
Following on, we evaluated the quantitative effect of enhancer–gene regulation by analyzing Micro-C data in conjunction with ATAC-seq, H3K27ac ChIP–seq and RNA-seq data using the ABC tool 22 . ABC interactions typically regulated two to three genes within 15–54 kb, and 62 of the risk loci fell within predicted enhancer regions that regulate genes.
Focusing on the Tier 1 variants at each locus (1–5 variants per locus; median, 1), 94 of the GWAS loci were linked to genes predicted by at least two sources of evidence (SMR normal, SMR tumor, ABC and Micro-C), and 10 had one source of evidence (Supplementary Table 6 ). Forty-two loci could not be associated with a gene, with the majority of these falling in expression-inactive regions (B-compartments). For 82 of the nominated loci, the closest gene was predicted to be the target gene, with 61 of these falling within introns. Approximately 70% of the nominated variants fell within the same topologically associating domain (TAD) as their target gene.
In addition to validating rs6983267 , which mediates its effect through a long-range interaction with MYC 18 , 29 , 30 , as the basis of the 8q24.21 association (Fig. 3a and Extended Data Fig. 2 ), our analysis provides evidence for the functional basis of the 170 risk loci and implicates 208 target genes. Although many of the risk loci have not previously been the subject of detailed scrutiny, several of the target genes have either well-documented roles in CRC or are strong a priori candidates for having a role in tumor biology. For example, we identify rs1248418 as the basis of the 10p12.1 association (top GWAS variant rs1773860 ; r 2 = 0.91, D ′ = 0.98). The enhancer region to which rs1248418 localizes shows a long-range interaction with the TSS of the gene encoding BAMBI, a negative regulator of transforming growth factor-β (TGFβ) signaling (Fig. 3b and Extended Data Fig. 3 ). Similarly, through functional annotation, we identify rs67631072 as the basis of the 1p34.3 locus (top GWAS variant rs61776719 ; r 2 = 1.0, D ′ = 1.0), with evidence from SMR and Micro-C implicating FHL3 (Fig. 3c and Extended Data Fig. 4 ). The C-risk allele ( P variant = 1.59 × 10 −16 ) is associated with increased expression of FHL3 ( P eQTL = 7.69 × 10 −16 ), which has been shown to have oncogenic functions through interactions with SMAD2, SMAD3 and SMAD4, key mediators of TGFβ signaling 33 , 34 , 35 . Our analysis also implicates rs9547700 (top GWAS variant rs12427846 ; r 2 = 0.96, D ′ = 0.98) as the functional basis of the 13q13.3 locus, and the risk allele is associated with reduced transcriptional activity and decreased SMAD9 expression, further emphasizing the central role of genetic variation in TGFβ signaling pathways as a determinant of genetic susceptibility (Supplementary Fig. 6 ).
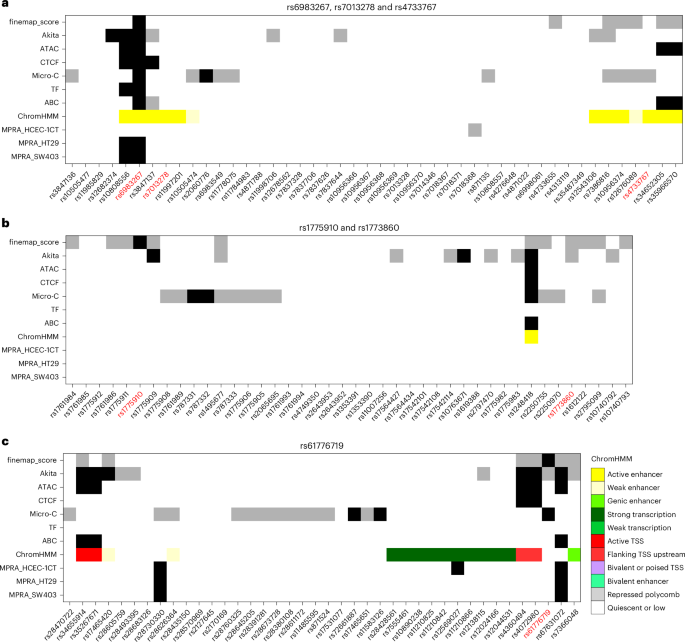
a , At the 8q24.21 locus, the GWAS identified rs6983267 , rs7013278 and rs4733767 , highlighted in red, as risk loci. rs6983267 and rs7013278 are within 1.5 kb of each other, but rs6983267 is better annotated, with strong hits for MPRAs, transcription factor binding, open chromatin (ATAC-seq) and Micro-C. rs4733767 is over 150 kb away from rs6983267 and rs7013278 and has separate annotations, so it is probably a true independent locus. b , At the 10p12.1 locus, rs1773860 was the lead GWAS variant at this locus, but rs1248418 ( r 2 = 0.91, D ′ = 0.98) was better annotated. This variant is located in open chromatin and is predicted to be in an enhancer region. In addition, this variant showed a long-range interaction with the TSS of BAMBI . c , Functional annotation of rs61776719 at the 1p34.3 locus identified rs67631072 ( r 2 = 1.0, D ′ = 1.0) as the top annotated variant, which shows enhancer activity in open chromatin regions and is predicted by the ABC model to affect gene expression. Detailed figures of the annotations of the regions are shown in Extended Data Figs. 2 – 4 . In all figure panels, gray blocks correspond to an annotation, and black blocks correspond to a strong annotation. ATAC denotes the presence of an ATAC-seq peak, CTCF denotes the presence of a CTCF peak from the ChIP–seq analysis and Akita denotes evidence of disruption of 3D chromatin structure. TF denotes that a transcription factor was predicted to bind.
Gene list analysis
By performing this integrated analysis, we identified a set of 208 genes from the GWAS loci (Fig. 4 and Supplementary Table 6 ) and showed here a direct link between the risk variant and an implicated gene. To determine which of the target genes that we identified are already known to have a role in CRC, and more broadly cancer, we used the text mining tool OncoScore 36 , which examines text from all available studies in the biomedical literature. To complement this analysis, we queried semantic predications within the Semantic MEDLINE Database 37 using MELODI Presto 38 . An integration of the results from these searches revealed that 142 of the 208 candidate target genes that we identified appear to have no documented role in CRC, and 47 of these presently have no established role in any cancer (Supplementary Tables 7 and 8 ).
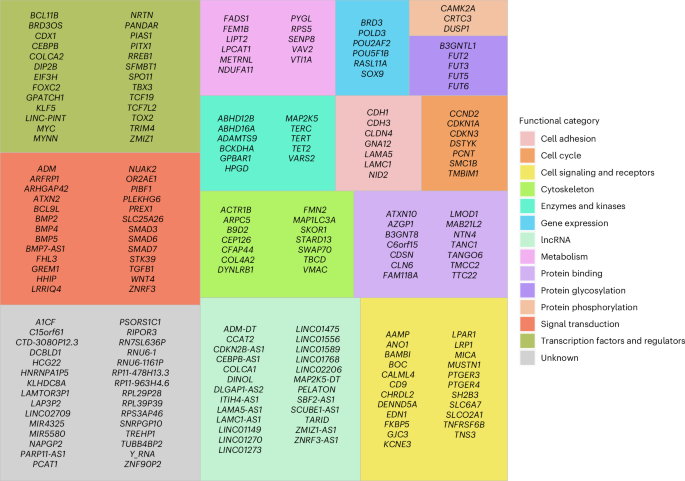
Genes that were identified in the integrated analysis were classified according to their biological or cellular function. The size of the box is proportional to the number of genes in the category.
One of the aspirations of GWASs is to inform therapeutics. To investigate the potential clinical utility of the CRC target genes identified at risk loci, we used oncoEnrichR 39 to explore multiple sources of functional and drug curation, including Open Targets 40 , 41 and DepMap 42 . For ten of the genes, there are already approved drugs that provide an opportunity for repurposing (Supplementary Table 9 ). These include crofelemer and misoprostol. Crofelemer inhibits ANO1, a calcium-activated chloride channel, which has a role in epithelial fluid secretion, and the gene is overexpressed in CRC. Misoprostol is a PTGER3 (prostaglandin receptor) agonist, potentially capable of addressing the downregulation of this receptor in tumors. In addition to these, several of the target genes identified are attractive drug targets, with 44 having clinical or discovery precedence, and a further 31 are likely to be tractable (Supplementary Table 10 ). Based on CRISPR knockout data, genomic biomarkers and patient data 43 , TBCD , KLF5 and SOX9 are also predicted to be promising therapeutic targets in CRC, as are CCND1 , CDH1 , MYC and POU5F1B in many different types of cancer (Supplementary Table 11 ).
After investigating regulatory networks in the gene list, we identified transcription factor regulatory interactions in cancer and normal cells. It was possible to observe sets of ‘hub’ genes, including MYC , MYNN , EGR1 , ZNF263 , CTCF and SP1 (Supplementary Fig. 7 ). Formally testing for molecular pathways enriched in the target genes revealed that the genes were enriched in TGFβ-related pathways (TGFβ signaling pathway, Kyoto Encyclopedia of Genes and Genomes (KEGG), P adjusted ( P adj ) = 4.31 × 10 −6 ; TGFβ signaling activation by blocking of tumor suppressors, Elsevier Pathway Collection, P adj = 4.4 × 10 −5 ; Hippo signaling pathway, KEGG, P adj = 6.50 × 10 −5 ; Wnt signaling pathway, KEGG, P adj = 9.38 × 10 −3 ), as well as in cancer-related pathways (pathways in cancer, KEGG, P adj = 1.43 × 10 −6 ). Given the central role of these pathways in CRC development, these findings expand opportunities for indirect targeting; for example, the use of porcupine inhibitors to indirectly target Wnt pathway activity 44 . Hence, adapted forms or modified dosing regimens of these drugs may offer alternative treatment options.
To prioritize functional variants for the identification of CRC susceptibility genes at risk loci, we systematically scored multiple genetic and functional features as well as assayed allelic transcriptional activity. Integration of these data nominated 208 variants at 170 risk loci, few of which have previously been formally investigated.
Our data support tissue-specific transcriptional regulation as a major mechanism through which GWAS variants influence CRC risk. Although 24% (40 out of 170) of the loci had a single Tier 1 variant, 58% (98 out of 170) featured more than one equally plausible functional variant. The potential of multiple functional variants at some loci to underscore CRC risk and plausibly target more than one gene is consistent with a study reporting that multiple causal regulatory variants in high linkage disequilibrium are responsible for a subset of lymphoblastoid cell eQTLs 5 . In 48% of the GWAS risk loci, the candidate target gene was the closest to the gene or intronic, often localizing within the same TAD. This is in line with the Open Targets gold standard dataset 40 , and this proximity effect has previously been noted and proposed to reflect evolutionary conservation 13 . For an appreciable proportion of risk loci, we found no obvious candidate genes, largely due to a paucity of functional data in these regions. This may be indicative of alternative mechanisms of action that were not explored here. For example, it has recently been proposed that the mechanistic basis of the 8q23.3 risk locus is a consequence of variable number tandem repeats 45 .
We acknowledge that this study has some limitations. First, MPRA-significant variants were not identified for 36% of the GWAS loci. The functional basis of these risk loci might operate through mechanisms that cannot be tested by MPRAs. However, we cannot exclude technical issues or simply lack of statistical power to demonstrate a difference in allelic transcriptional activity. Second, for 96 loci, we could not assign a target gene using eQTLs. We have sought to address the cellular context of eQTLs, analyzing both normal and tumor data, although failure to demonstrate a relationship may reflect a lack of statistical power, especially for lower-frequency variants. Therefore, rather than rely solely on eQTLs, we performed an ABC-model-based analysis utilizing epigenomic features and Micro-C data to predict the enhancer–gene connections.
Accepting these caveats, we performed a multilayered analysis that enabled us to nominate the probable causal variants for the CRC risk loci and implicate 208 gene targets as the biological basis of associations. Only six of the genes we identified ( BCL9L , CDH1 , SMAD3 , SOX9 , TBX3 and TCF7L2 ) are established CRC driver genes 46 (that is, genes with recurrent nonsynonymous somatic mutations in CRC under positive selection). This suggests a model by which genetic predisposition indirectly affects oncogenesis. In addition to emphasizing the role of genetic variation in established CRC genes and pathways, we identify candidate target genes with hitherto no previously well-established role. Notably, these include components of the calmodulin superfamily, CALML4 and CAMK2A . The calmodulin pathway is the principal calcium sensor regulating a myriad of vital biological processes, including cell proliferation, programmed cell death and autophagy, and is increasingly viewed as an attractive therapeutic target 47 . SLCO2A1 , which has a role in the synthesis and clearance of prostaglandins, along with FADS1 , also highlights the importance of inflammation and the immune response in CRC development. The identification of ATXN10 and ATXN2 as candidate target genes provides support for the involvement of the Ras–MAP kinase pathway and EGFR trafficking in CRC development. BCKDHA catalyzes the breakdown of branched-chain amino acids, the dysregulation of which is recognized to have a role in the progression of a range of cancers 48 .
In summary, we provide further insight into the functional basis of risk loci, implicating novel genes in the development of CRC, which expands the potential for therapeutic targeting. Our analysis provides an outline for a generalized strategy to profile disease-associated GWAS loci using high-throughput variant screening in concert with multilayered functional annotation.
For the eQTL data, all participants provided informed written consent, and the research was approved by local research ethics committees (SOCCS 11/SS/0109 and 01/0/05) and National Health Service management (SOCCS 2013/0014, 2003/W/GEN/05).
GWAS statistics and definition of risk loci
GWAS summary association statistics were obtained from the recently published GWAS meta-analysis of 100,204 CRC cases and 154,587 controls 2 . Risk loci were defined as variants with P < 5 × 10 −8 and that were at least 500 kb apart. To identify secondary signals inside this window, a conditional analysis was performed on the meta-analysis summary statistics using genome-wide complex trait analysis with conditional and joint analysis 49 . As the GWAS data were based on east Asian and European individuals, we used genotyping data from 6,684 unrelated individuals of east Asian ancestry and 4,284 individuals of European ancestry from the UK10K project 50 and the 1000 Genomes Project 51 , respectively, as a reference for an estimation of linkage disequilibrium. The conditional analysis was performed on each population separately, and the data were combined using a meta-analysis, retaining associations where P conditional < 5 × 10 −8 . In total, there were 204 autosomal variants identified, which mapped to 170 loci.
Cell lines and cell culture
CRC cell lines were cultured in 5% CO 2 at 37 °C, with SW403 (ACC294, DSMZ), SW480 (ACC313, DSMZ) and SW948 (91030714, ECACC) grown in DMEM (Gibco), HT29 (ACC299, DSMZ) in McCoy’s 5A (Modified) Medium (Gibco) with GlutaMAX Supplement (Gibco), CL11 (ACC467, DSMZ) in DMEM/F-12 (Gibco), and C32 (ECACC) in Iscove's Modified Dulbecco's Medium (Gibco). Media were supplemented with 10% (20% for CL11) heat-inactivated FBS (Sigma). The normal colon crypt cell line HCEC-1CT (CkHT039-0229, Evercyte) was cultured in a 4:1 ratio of DMEM and Medium 199 (Gibco) supplemented with ColoUp medium (Evercyte) at 37 °C, with 3% O 2 and 5% CO 2 . All cell lines were cultured until they reached 90% confluency and then passaged using TrypLE (Gibco).
Variant selection
We used MPRAs to identify variants exhibiting transcriptional differences. The nature of the assay requires that the variants to be tested are predefined, and the number of variants tested were constrained by oligonucleotide synthesis chip capacity. In light of this, using data from the CRC GWAS, we selected variants for MPRA testing by first considering all variants in a 500-kb window spanning each primary or conditional association (that is, ±250 kb around each lead variant) whose P values were within three orders of magnitude of that of the lead variant. As this might not capture functional variants that remain highly significant at some loci (that is, where the lead variant has an extremely strong association), we also considered variants having −log 10 ( P variant ) > 0.7 × (−log 10 ( P lead variant )), stipulating an r 2 of >0.2 for the lead variant and a P variant of <10 −5 in the GWAS. A total of 100 control variants were also evaluated: 50 were derived from common variants (minor allele frequency > 0.05) that mapped to repressive regions (greater than fourfold enriched versus input) as defined by the NIH Roadmap Epigenomics Project colonic (E-075) H3K27me3 mark, and the remaining 50 were randomly generated.
Variant oligonucleotide library design
For each variant, 100-bp flanking sequences were added, yielding genomic probe sequences of 201 bp (100 + 1 + 100). Oligonucleotides containing an SceI restriction site, which was used for cloning, were excluded. During library synthesis, probe orientation was determined by the addition of two adapter sequences (AGGACCGGATCAACT and CATTGCGTGAACCGA) at either the 5′ and 3′ ends or the 3′ and 5′ ends relative to the probe sequence. Each variant had four probes: one for each combination of forward and reverse strands and one for each reference and alternative allele. Library synthesis was performed by Twist Bioscience.
Library construction, transfection and sequencing
A lentiviral MPRA was carried out as previously described 52 . In brief, the MPRA library was amplified using 12 cycles of PCR, with adapter sequences as primers. All PCR reactions were performed using Q5 High-Fidelity 2X Master Mix (NEB). Subsequent rounds of PCR incorporated a random 15-base polymer barcode sequence for probe identification. Barcoded probes were incorporated into a pLS-SceI vector (a gift from N. Ahituv; Addgene plasmid no. 137725) by Gibson assembly using NEBuilder HiFi DNA Assembly Cloning Kit (NEB). After ligation, 100 ng of plasmid was transfected into NEB Stable Competent E. coli (High Efficiency) (NEB) using an Eppendorf Eporator at 1.8 kV. Bacteria were plated on carbenicillin (500 µg ml −1 ) agar plates. A total of 2 × 10 6 colonies, sufficient for 100 unique barcodes per probe, were collected, and plasmid DNA was purified using ZymoPURE II Plasmid Maxiprep Kits (Zymo Research) before Illumina-based library preparation. Barcode-to-probe association was carried out by sequencing 4 nM of the pLS-SceI library on an Illumina MiSeq using a MiSeq Reagent Kit v2 (300 cycles) with three custom primers. Primer sequences are provided in Supplementary Table 9 . Custom primers were diluted to a final concentration of 0.5 μM and added as follows: pLSmP-ass-seq-R1 (forward probe) read 1 (146 cycles), pLSmP-ass-seq-R2 (reverse probe) read 2 (146 cycles) and pLSmP-ass-seq-ind1 (forward barcode) index read 1 (15 cycles). The sample index read 2 (10 cycles) was performed using the default Illumina P5 primer.
Lentivirus particles were produced in HEK239T cells (CRL-11268, ATCC). For one T175 flask, 10 µg pLS-SceI, 6.5 µg psPAX2 and 3.5 µg pMD2.G were diluted in 2 ml of Opti-MEM (Gibco) and 40 µl of TurboFect (Thermo Fisher Scientific) and added according to the manufacturer’s guidelines. Other virus preparation steps were carried out as previously described 52 . HT29, SW403 and HCEC-1CT cells were used for enhancer quantitation. Cells were transduced with a viral moiety of infection of 80 based on cell-line-specific or batch-specific viral transduction efficiencies using 8 µM polybrene (Sigma). SW403 cells were transduced before attachment (reverse transduction), and the other cell lines were allowed at least 24 h to attach. After 24 h, the medium was removed, and the cells were incubated for an additional 48 h. The cells were lysed, and the RNA and DNA were purified using an AllPrep DNA/RNA Kit (Qiagen). DNA and RNA library preparation and sequencing were performed as previously described 52 . DNA and RNA samples were uniquely indexed, and a 16-bp random molecular identifier was added using PCR to eliminate optical duplicates. For each cell line, three DNA and three RNA replicate libraries were combined in equimolar amounts. DNA and RNA libraries from each cell line were mixed at a 1:3 ratio and diluted to 7.89 nM for sequencing. MPRA libraries were sequenced using a NovaSeq 6000 (Illumina) using the following primers: pLSmP-ass-seq-ind1 (forward barcode) read 1 (15 cycles), pLSmP-bc-seq (reverse barcode) read 2 (15 cycles) and pLSmP-UMI-seq (forward unique molecular identifier) index read 1 (16 cycles) (Supplementary Table 12 ). Sample indexes (index read 2, 10 cycles) were sequenced using the default Illumina P5 primer.
Data analyses
Raw sequencing data were converted to FASTQ format using bcl2fastq (Illumina). The MPRAflow 52 pipeline v2.3.5 was used to associate and count the number of barcodes associated with each probe sequence. To identify the different alleles for each variant, the FASTQ files were modified to include the forward library adapter (AGGACCGGATCAACT). This sequence was also added to the design FASTA file used by MPRAflow for alignment. For a sequence to be associated with a given barcode, it had to be a perfect match to the library sequence, which was enforced using a CIGAR string of 230M. For statistical analysis of the MPRA data, we used MPRAnalyze 53 v1.12.0, which uses a nested pair of generalized linear models designed to estimate noise in the DNA and RNA libraries. We filtered the barcodes and collected those that contained all four allele-specific probes (that is, fwd_ref, fwd_alt, rev_ref and rev_alt), and we only retained a barcode if there was a DNA read present with a corresponding RNA read in the same replicate. Library size correction factors were estimated according to the replicate number, allele type (alternative or reference) and stand (forward or reverse) using the upper quantile of nonzero values for depth estimation. Owing to the large number of barcodes, we used the ‘scaled’ option, which uses the DNA counts directly as estimates rather than generating a DNA model. There was a strong correlation between the scaled analysis and the full model in a downsampled dataset (Supplementary Fig. 8 ). A likelihood test was performed to test the effect of the allele using the direction and replicate as covariates.
ChIPmentation
ChIPmentation was performed on histone marks H3K4me1 (C15410194, Diagenode), H3K4me3 (C15410003-50, Diagenode), H3K27ac (C15410196, Diagenode), H3K27me3 (C15410195, Diagenode), H3K36me3 (C15410192, Diagenode) and CTCF (C15410210-50, Diagenode) for the C32, CL11, HT29, SW403, SW480, SW948 cell lines using a published protocol 54 , with minor modifications, as detailed in the Supplementary Note. Data processing was performed using the Nextflow nf-core chipseq pipeline v1.2.1 (ref. 55 ) with default parameters.
ATAC-seq was performed on the C32, CACO2, CL11, HT29, SW403, SW480, SW948 and HCEC-1CT cell lines as previously described 56 . Experimental protocols are detailed in the Supplementary Note. Data processing was performed using the Nextflow nf-core atacseq pipeline v1.2.1 (ref. 57 ) with default parameters. Peaks for both the ChIP–seq and ATAC-seq data were consistent across all cell lines (Supplementary Fig. 9 ).
We generated Micro-C chromatin interaction maps of the CL11, HT29, SW403, SW480 and SW948 cell lines as previously described 58 , 59 . Experimental protocols are detailed in the Supplementary Note. The data were analyzed using JuicerTools v1.22 (ref. 60 ) to count valid interactions. We required valid interactions of >90% for classification as cis -contacts, of which 60–70% had to be short-range cis -contacts. If the metrics were satisfactory, the pooled library was sequenced on a NovaSeq 6000 (Illumina) to a depth of at least 300 million reads per library, using 100 bp paired-end sequencing.
We used the nf-distiller pipeline 61 v0.3.4 to generate the interaction maps from the raw FASTQ files, using matrix balancing normalization and binning at 1 kb. FitHiC2 (ref. 62 ) was used to call significant interactions, merging adjoining bins with significant interactions. TADs and compartments were identified using cooltools 63 v0.5.4 with 30-kb and 100-kb windows, respectively, and binning at 10 kb. Compartments were determined using an eigendecomposition of the contact matrix. The GC content of each bin was used as a phasing track. Active and inactive compartments are defined as having a positive and negative value for the first eigenvector, respectively.
RNA extraction and library sequencing
RNA sequencing of the C32, CL11, HT29, SW403, SW948 and HCEC-1CT cell lines was performed. The experimental protocols are detailed in the Supplementary Note. Analysis of the RNA-seq data was performed using the RNAflow pipeline 64 v1.4.1 with default parameters.
Cell-type specificity of risk variants
To identify the cell types through which CRC risk variants exert their effects, we analyzed single-cell gene-expression profiles across different tissues using the Tabula Sapiens v4 dataset 23 (~500,000 cells from 24 organs from 15 normal human subjects) and across different intestinal regions using the Gut Cell Atlas 24 (~125,000 cells from 86 healthy adults from 11 distinct locations in the gut). We used scDRSs 65 v1.0.1 to link the scRNA-seq data with polygenic disease risk at a single-cell resolution, independent of cell type. In brief, using the CRC GWAS association summary statistics, MAGMA 66 v1.10 defined a putative set of disease genes. Using the top 1,000 putative genes, a disease score was calculated as a function of the GWAS z-scores and the scRNA-seq expression values. Cell-specific association P values were calculated by comparing normalized disease scores to an empirical distribution of normalized scores across all control gene sets and all cells.
Histone mark enrichment analysis
To examine enrichment in specific histone marks across the risk loci, we adapted the variant set enrichment method described previously 67 , 68 . In brief, for each risk locus, a region of strong linkage disequilibrium (defined as r 2 ≥ 0.8 and D ′ ≥ 0.8) was determined, and variants mapping to these regions were termed the associated variant set (AVS). ChIP–seq data for the H3K4me3, H3K27ac, H3K4me1, H3K27me3, H3K9ac, H3K9me3 and H3K36me3 chromatin marks from up to 128 cell types were obtained from the NIH Roadmap Epigenomics Project data 25 . For each mark, the overlap of the positions of variants in the AVS and the ChIP–seq peaks was determined to produce a mapping tally. A null distribution was generated by randomly selecting variants with the same linkage disequilibrium characteristics as the risk-associated variants, and a null mapping tally was calculated. This process was repeated 50,000 times, and approximate P values were calculated as the proportion of permutations for which the null mapping tally was greater or equal to the AVS mapping tally.
We used ChromHMM 69 v1.24 to predict chromatin states using the H3K4me1, H3K4me3, H3K27ac, H3K27me3 and H3K36me3 histone marks. The BAM files from the nf-core chipseq pipeline described above were binarized, and a 15-state model was predicted (Supplementary Fig. 10 ). States were annotated using previously published annotations 69 , 70 , 71 .
Fine-mapping of risk loci
Using summary data from the CRC GWAS, we defined flanking regions 500 kb on either side of the most significant variant at each risk locus. We performed statistical fine-mapping of these CRC risk loci using PolyFun 26 v1.4.1 and susieR 27 v0.11.92. We calculated the previous causal probabilities nonparametrically using the established PolyFun protocol, which estimates the per-SNP heritability for each variant, weighted by the functional annotations. Annotation data were gathered from the baseline-LF v2.2 annotation data 26 , 72 provided by the A. Price group ( https://alkesgroup.broadinstitute.org/LDSCORE ) using the CRC-specific ChIP–seq and open chromatin data that were generated in-house (Supplementary Table 1 ). Linkage disequilibrium scores were calculated using data from 45,498 disease-free European individuals in the Genomics England dataset ( https://re-docs.genomicsengland.co.uk/aggv2 ). Using the probabilities estimated by PolyFun, we fine-mapped loci across a 500-kb window using the Sum of Single Effects model, which was implemented in susieR. For loci with one independent variant, we set the maximum number of causal variants to two, as susieR is unable to use linkage disequilibrium information for a single variant. For loci with multiple independent variants, we performed fine-mapping of the region including all independent variants, and set the maximum number of causal variants equal to the number of independent variants. The output from susieR included a posterior inclusion probability (PIP) for each variant and the 95% credible set that the variant belongs to. Variants with PIPs of >0.001 and that cumulatively reached a probability of 0.95 were included in a credible set.
Transcription factor binding
We used TOBIAS 73 v0.14.0 to predict transcription factor binding using the BINDetect method. Using the ATAC-seq data from the C32, CACO2, CL11, HT29, SW403, SW480, SW948 and HCEC-1CT cell lines in conjunction with the JASPAR 2022 core nonredundant transcription factor motif database 28 , which was filtered to motifs found in humans, we predicted whether there were any potential transcription factors bound in open chromatin. The TOBIAS scores indicate how well the transcription factor motif matches the genomic sequence.
We performed an enrichment analysis of bound transcription factors according to the NIH Roadmap Epigenomics Project histone analysis. The number of transcription factors predicted to bind to each of the GWAS regions (based on the selected variants) were counted. A null distribution was generated by randomly selecting variants with P variant > 0.95; a window of a size equivalent to that of the GWAS data was formed, and a null transcription factor count was calculated. This process was repeated 50,000 times, and approximate P values were calculated as the proportion of permutations for which the null transcription factor count was greater or equal to the GWAS transcription factor count.
ABC model for prediction of enhancer–gene interactions
To predict enhancer–gene connections in each cell line, we used ABC 22 v0.2.2 in conjunction with data from ATAC-seq, H3K27ac ChIP–seq, Micro-C and RNA-seq. The analysis was performed as previously described 22 using default parameters. In brief, we investigated the 150,000 gene–enhancer interactions with the highest ABC scores for all enhancer regions within 5 Mb of the TSS of a gene. Enhancer regions were filtered such that those overlapping the GWAS risk loci were retained.
eQTLs and SMR
Comprehensive details about the RNA-seq and whole-genome sequencing data from the SOCCS and GTEx datasets are described in ref. 74 . In brief, the GTEx data were derived from 367 postmortem transverse colon samples, and the SOCCS data were derived from the normal colon or rectum mucosa of 223 healthy individuals. eQTL analysis was performed using Matrix eQTL v2.3 (ref. 75 ) on probabilistic estimation of expression residuals-adjusted residuals 76 , and age, sex, batch and a number of hidden covariates equal to one quarter of the sample size in both datasets were taken into account. The variants tested were limited to those within 0.5 Mb of lead variants, with a minor allele frequency of >0.01 and associations with genes within 1 Mb. Per-dataset results underwent a meta-analysis using a fixed-effects inverse variance-weighted model in META 77 v1.7. SMR 32 v1.3.1 analysis was performed using the eQTL results from the meta-analysis and GWAS summary statistics from ref. 2 using default parameters. As SMR is performed only on the top eQTLs for each gene, P SMR values were Bonferroni-corrected for multiple testing based on the number of genes within each risk locus. We retained results with P SMR-corrected < 0.05 and P HEIDI > 0.05. To analyze the preservation of CRC risk-associated eQTL effects in tumors, eQTL summary statistics from TCGA COAD ( n = 286) and READ ( n = 94) samples were obtained from PancanQTL 78 , underwent a meta-analysis and subjected to SMR as described above.
3D chromatin structure disruption
To predict the effect of variants on the 3D structure of DNA, we used Akita 31 v0.6, which uses a deep learning framework. The Micro-C data were binned into 1,024 (2 10 )-bp sets. Data were preprocessed using default parameters, except for a sequence length of 1,048,576 (2 20 ) bp and a crop length of 65,536 (2 16 ) bp. The model was trained using default parameters, with 10% of the data used for testing and 10% used for validation. We performed in silico mutagenesis on a nucleotide level on 200-bp regions centered on each tested variant. Disruption scores were calculated as the L2 norm of the predicted differences between the contact maps for each allele.
Scoring of variants
To prioritize the variants in each of the risk loci, we adopted the following scoring scheme:
MPRA: variants with an FDR of ≤10 −3 were given a score of 2, and those with an FDR of ≤0.05 were given a score of 1. Each cell line was considered separately.
Statistical fine-mapping: variants with a PIP of >0 were given a score of 1 (that is, the variant was part of a credible set), and those with a PIP of >0.5 were given a score of 2.
Chromatin annotation: based on ChromHMM annotation, variants that fell within either a promoter or an enhancer region were given a score of 2, and those that fell within regions with weak predicted states (that is, with lower emission parameters) were given a score of 1.
Open chromatin: if the variants fell within an ATAC-seq peak, then they were given a score of 2.
SMR: for both the normal and tumor samples, if a variant was associated with a gene identified using SMR, then it was given a score of 2.
Akita: if >25% of the variants within 100bp of the tested variant had a disruption score in the top 10% of all disruption scores, then it was given a score of 2. If >25% of the variants within 100bp of the tested variant had a disruption score in the top 20% of all disruption scores, then it was given a score of 1.
CTCF: if the variants fell within a CTCF peak, then they were given a score of 2.
Long-range interaction: using the output from FitHiC (filtered using −log 10 ( P ) ≥ 2), if the variant fell within one end of a Micro-C contact and the other end was within a gene body, then it was given a score of 1. If the other end of the interaction contained a TSS of a gene, then the variant was given a score of 2.
As many of these analyses were performed on multiple cell lines, it was necessary to find a scoring consensus across cell lines for collation of the scores. For the ATAC-seq, CTCF, Micro-C and ChromHMM data, this consensus was that the annotation had to be present in >50% of the cell lines. For the ABC model and the transcription factor binding prediction, we performed a binomial analysis of the scores from the respective analysis tools. For each analysis, we identified the number of times that the score was in the 90th percentile. We calculated the probability of the occurrence of this score using the binomial distribution survival function. If P < 0.05, the tested variant was assigned a score of 1, and if P < 0.01, it was given a score of 2.
The annotation scores for each variant were summed, and the scores were ranked. The variants with scores in the top 20% of all scores were designated as Tier 1 variants, those with scores in the bottom 50% as Tier 3 and the remainder as Tier 2.
Gene prioritization
To link the variants with genes, we used the data from the Micro-C TSSs, the ABC model and SMR of the tumor and normal samples. We focused on the Tier 1 variants, as they provided the most information regarding annotations. A gene annotation had to be present in at least two of the annotation sources to be suggested as a putative target gene. If no genes reached this threshold, we included the interactions related to the Micro-C data within a gene body and included these as weak predictions. For any genes that did not have any Tier 1 variants, we used the Tier 2 variants instead, and any genes that were found were labeled as weak predictions. Finally, if no genes were identified throughout the process and the variant was intronic to a gene, that gene was used as a weak prediction.
Gene evidence
To formally examine whether target genes were known to be associated with cancer (and specifically CRC), we used OncoScore 36 v1.30.0, a text mining tool that ranks genes by their association with cancer based on the available biomedical literature. We used an OncoScore of 21.09 as the threshold to define novelty. To complement this analysis, we also performed a literature survey in MELODI Presto (accessed 3 April 2024) 38 using semantic predications in the Semantic MEDLINE Database 37 , which is based on all citations in PubMed. Within the Semantic MEDLINE Database, pairs of terms were connected by a predicate, which are collectively known as ‘literature triples’ (that is, ‘subject term’–predicate–‘object term’). We performed the analysis using the gene list as the subject and ‘colorectal cancer’ as the object. Driver genes were determined using intOGen (released 1 February 2020) 46 and restricted to those from colorectal cohorts (COADREAD). Gene distance information was obtained from HaploReg v4.1.
We used oncoEnrichR 39 v1.4.2.1 to analyze the gene sets. This tool provides a suite of analyses, including cancer associations, drug associations, synthetic lethality, gene fitness and protein–protein interactions.
Regulatory interaction data were obtained from the DoRothEA and OmniPath resources 79 , 80 . These datasets contains a list of previously identified transcription factor–target interactions that are scored based on multiple lines of evidence (namely, literature-curated resources, ChIP–seq peaks, transcription factor binding site motifs and gene-expression-inferred interactions). Regulatory interactions were inferred using gene expression in tumor samples (from TCGA) or normal tissues (from GTex).
Cell viability and gene essentiality data were obtained from the Cancer Dependency Map (DepMap, 2020_Q2 release), which provides information on a systematic genome-scale CRISPR–Cas9 drop-out screen in 912 cancer cell lines 42 . We restricted the analysis to the CRC cell lines from primary tumors (that is, nonmetastatic; n = 37). To identify putative therapeutic targets, we used the results from the Project Score database (2021_Q2 release) 42 , 43 in DepMap. This generates target priority scores based on the integration of CRISPR knockout gene fitness effects with genomic biomarker and patient data (accounts for 30% of the score and is based on evidence of a genetic biomarker associated with a target dependency, as well as tumor prevalence), and cell line fitness effects (accounts for 70% of the score and is based on gene fitness, genes expressed and genes not homozygously deleted). All genes are assigned a target priority score between zero and 100 from lowest to highest priority. A threshold score of 40 was established based on scores calculated for targets with approved or preclinical cancer compounds.
Drug tractability information was based on data from the Open Targets Platform 41 , and pathway enrichment was performed using Enrichr (released 8 June 2023) 81 .
Reporting summary
Further information on research design is available in the Nature Portfolio Reporting Summary linked to this article.
Data availability
GWAS data are available from GWAS Catalog (accession no. GCST90129505 ). Cell line data have been deposited in the European Genome-phenome Archive under the following accessions: EGAD50000000596 (MPRA), EGAD50000000294 (Micro-C), EGAD50000000295 (ChIP–seq, all marks), EGAD50000000296 (ATAC-seq), EGAD50000000297 (RNA-seq). Annotation data for all the GWAS regions are available on the University of California, Santa Cruz (UCSC) Genome Browser ( https://genome.ucsc.edu/s/philip.law%40icr.ac.uk/CRC%20GWAS%20annotation ).
Single cell RNA-seq data were obtained from the Gut Cell Atlas ( https://www.gutcellatlas.org ) and the Tabula Sapiens project ( https://tabula-sapiens-portal.ds.czbiohub.org ). Transcription factor binding was based on data from JASPAR ( https://jaspar.genereg.net ). Functional annotations for the fine-mapping were obtained from the A. Price group ( https://alkesgroup.broadinstitute.org/LDSCORE ). Histone marks in different tissues were obtained from the NIH Roadmap Epigenomics Project ( https://egg2.wustl.edu/roadmap/web_portal ). eQTL data were obtained from PancanQTL ( http://bioinfo.life.hust.edu.cn/PancanQTL ) and GTEx ( https://gtexportal.org ). Literature mining was performed in MELODI Presto ( https://melodi-presto.mrcieu.ac.uk ) using data from the Semantic MEDLINE Database ( https://lhncbc.nlm.nih.gov/ii/tools/SemRep_SemMedDB_SKR.html ). Gene annotation data were obtained from OmniPath ( https://omnipathdb.org ), DoRothEA ( https://saezlab.github.io/dorothea ), DepMap ( https://depmap.org ) and Open Targets ( https://www.opentargets.org ), and analyzed in oncoEnrichR ( https://oncotools.elixir.no ). Source data are provided with this paper.
Code availability
No custom code was generated. Publicly available code was used for all aspects of data processing and analysis and is cited in the appropriate section of the Methods.
Lichtenstein, P. et al. Environmental and heritable factors in the causation of cancer–analyses of cohorts of twins from Sweden, Denmark, and Finland. N. Engl. J. Med. 343 , 78–85 (2000).
Article CAS PubMed Google Scholar
Fernandez-Rozadilla, C. et al. Deciphering colorectal cancer genetics through multi-omic analysis of 100,204 cases and 154,587 controls of European and east Asian ancestries. Nat. Genet. 55 , 89–99 (2023).
Schaid, D. J., Chen, W. & Larson, N. B. From genome-wide associations to candidate causal variants by statistical fine-mapping. Nat. Rev. Genet. 19 , 491–504 (2018).
Article CAS PubMed PubMed Central Google Scholar
Tewhey, R. et al. Direct identification of hundreds of expression-modulating variants using a multiplexed reporter assay. Cell 165 , 1519–1529 (2016).
Abell, N. S. et al. Multiple causal variants underlie genetic associations in humans. Science 375 , 1247–1254 (2022).
Ajore, R. et al. Functional dissection of inherited non-coding variation influencing multiple myeloma risk. Nat. Commun. 13 , 151 (2022).
Choi, J. et al. Massively parallel reporter assays of melanoma risk variants identify MX2 as a gene promoting melanoma. Nat. Commun. 11 , 2718 (2020).
Long, E. et al. Massively parallel reporter assays and variant scoring identified functional variants and target genes for melanoma loci and highlighted cell-type specificity. Am. J. Hum. Genet. 109 , 2210–2229 (2022).
Karnuta, J. M. & Scacheri, P. C. Enhancers: bridging the gap between gene control and human disease. Hum. Mol. Genet 27 , R219–R227 (2018).
Ying, P. et al. Genome-wide enhancer-gene regulatory maps link causal variants to target genes underlying human cancer risk. Nat. Commun. 14 , 5958 (2023).
Gschwind, A. R. et al. An encyclopedia of enhancer-gene regulatory interactions in the human genome. Preprint at bioRxiv https://doi.org/10.1101/2023.11.09.563812 (2023).
Yao, D. W., O’Connor, L. J., Price, A. L. & Gusev, A. Quantifying genetic effects on disease mediated by assayed gene expression levels. Nat. Genet. 52 , 626–633 (2020).
Mostafavi, H., Spence, J. P., Naqvi, S. & Pritchard, J. K. Systematic differences in discovery of genetic effects on gene expression and complex traits. Nat. Genet. 55 , 1866–1875 (2023).
Soskic, B. et al. Chromatin activity at GWAS loci identifies T cell states driving complex immune diseases. Nat. Genet. 51 , 1486–1493 (2019).
Trynka, G. et al. Chromatin marks identify critical cell types for fine mapping complex trait variants. Nat. Genet. 45 , 124–130 (2013).
Downes, D. J. et al. Identification of LZTFL1 as a candidate effector gene at a COVID-19 risk locus. Nat. Genet. 53 , 1606–1615 (2021).
Orozco, G., Schoenfelder, S., Walker, N., Eyre, S. & Fraser, P. 3D genome organization links non-coding disease-associated variants to genes. Front. Cell Dev. Biol. 10 , 995388 (2022).
Article PubMed PubMed Central Google Scholar
Jäger, R. et al. Capture Hi-C identifies the chromatin interactome of colorectal cancer risk loci. Nat. Commun. 6 , 6178 (2015).
Article PubMed Google Scholar
Fachal, L. et al. Fine-mapping of 150 breast cancer risk regions identifies 191 likely target genes. Nat. Genet. 52 , 56–73 (2020).
Michailidou, K. et al. Association analysis identifies 65 new breast cancer risk loci. Nature 551 , 92–94 (2017).
Fulco, C. P. et al. Activity-by-contact model of enhancer-promoter regulation from thousands of CRISPR perturbations. Nat. Genet. 51 , 1664–1669 (2019).
Nasser, J. et al. Genome-wide enhancer maps link risk variants to disease genes. Nature 593 , 238–243 (2021).
Tabula Sapiens, C. et al. The Tabula Sapiens: a multiple-organ, single-cell transcriptomic atlas of humans. Science 376 , eabl4896 (2022).
Article Google Scholar
Elmentaite, R. et al. Cells of the human intestinal tract mapped across space and time. Nature 597 , 250–255 (2021).
Kundaje, A. et al. Integrative analysis of 111 reference human epigenomes. Nature 518 , 317–330 (2015).
Weissbrod, O. et al. Functionally informed fine-mapping and polygenic localization of complex trait heritability. Nat. Genet. 52 , 1355–1363 (2020).
Wang, G., Sarkar, A., Carbonetto, P. & Stephens, M. A simple new approach to variable selection in regression, with application to genetic fine mapping. J. R. Stat. Soc. Series B Stat. Methodol. 82 , 1273–1300 (2020).
Castro-Mondragon, J. A. et al. JASPAR 2022: the 9th release of the open-access database of transcription factor binding profiles. Nucleic Acids Res. 50 , D165–D173 (2022).
Pomerantz, M. M. et al. The 8q24 cancer risk variant rs6983267 shows long-range interaction with MYC in colorectal cancer. Nat. Genet. 41 , 882–884 (2009).
Tuupanen, S. et al. The common colorectal cancer predisposition SNP rs6983267 at chromosome 8q24 confers potential to enhanced Wnt signaling. Nat. Genet. 41 , 885–890 (2009).
Fudenberg, G., Kelley, D. R. & Pollard, K. S. Predicting 3D genome folding from DNA sequence with Akita. Nat. Methods 17 , 1111–1117 (2020).
Zhu, Z. et al. Integration of summary data from GWAS and eQTL studies predicts complex trait gene targets. Nat. Genet. 48 , 481–487 (2016).
Cao, G. et al. FHL3 contributes to EMT and chemotherapy resistance through up-regulation of Slug and activation of TGFβ/Smad-independent pathways in gastric cancer. Front. Oncol. 11 , 649029 (2021).
Hou, Y. et al. The circular RNA circ_GRHPR promotes NSCLC cell proliferation and invasion via interactions with the RNA-binding protein PCBP2. Clin. Exp. Pharmacol. Physiol. 48 , 1171–1181 (2021).
Huang, Z., Yu, C., Yu, L., Shu, H. & Zhu, X. The roles of FHL3 in cancer. Front. Oncol. 12 , 887828 (2022).
Piazza, R. et al. OncoScore: a novel, Internet-based tool to assess the oncogenic potential of genes. Sci. Rep. 7 , 46290 (2017).
Kilicoglu, H., Shin, D., Fiszman, M., Rosemblat, G. & Rindflesch, T. C. SemMedDB: a PubMed-scale repository of biomedical semantic predications. Bioinformatics 28 , 3158–3160 (2012).
Elsworth, B. & Gaunt, T. R. MELODI Presto: a fast and agile tool to explore semantic triples derived from biomedical literature. Bioinformatics 37 , 583–585 (2021).
Nakken, S. et al. Comprehensive interrogation of gene lists from genome-scale cancer screens with oncoEnrichR. Int. J. Cancer 153 , 1819–1828 (2023).
Mountjoy, E. et al. An open approach to systematically prioritize causal variants and genes at all published human GWAS trait-associated loci. Nat. Genet. 53 , 1527–1533 (2021).
Ochoa, D. et al. The next-generation Open Targets Platform: reimagined, redesigned, rebuilt. Nucleic Acids Res. 51 , D1353–D1359 (2023).
Pacini, C. et al. Integrated cross-study datasets of genetic dependencies in cancer. Nat. Commun. 12 , 1661 (2021).
Behan, F. M. et al. Prioritization of cancer therapeutic targets using CRISPR-Cas9 screens. Nature 568 , 511–516 (2019).
Ho, S. Y. & Keller, T. H. The use of porcupine inhibitors to target Wnt-driven cancers. Bioorg. Med. Chem. Lett. 25 , 5472–5476 (2015).
Mukamel, R. E. et al. Repeat polymorphisms underlie top genetic risk loci for glaucoma and colorectal cancer. Cell 186 , 3659–3673.e23 (2023).
Martinez-Jimenez, F. et al. A compendium of mutational cancer driver genes. Nat. Rev. Cancer 20 , 555–572 (2020).
Villalobo, A. & Berchtold, M. W. The role of calmodulin in tumor cell migration, invasiveness, and metastasis. Int. J. Mol. Sci. 21 , 765 (2020).
Yang, D. et al. Branched-chain amino acid catabolism breaks glutamine addiction to sustain hepatocellular carcinoma progression. Cell Rep. 41 , 111691 (2022).
Yang, J., Lee, S. H., Goddard, M. E. & Visscher, P. M. GCTA: a tool for genome-wide complex trait analysis. Am. J. Hum. Genet. 88 , 76–82 (2011).
Consortium, U. K. et al. The UK10K project identifies rare variants in health and disease. Nature 526 , 82–90 (2015).
Genomes Project, C. et al. A global reference for human genetic variation. Nature 526 , 68–74 (2015).
Gordon, M. G. et al. lentiMPRA and MPRAflow for high-throughput functional characterization of gene regulatory elements. Nat. Protoc. 15 , 2387–2412 (2020).
Ashuach, T. et al. MPRAnalyze: statistical framework for massively parallel reporter assays. Genome Biol. 20 , 183 (2019).
Schmidl, C., Rendeiro, A. F., Sheffield, N. C. & Bock, C. ChIPmentation: fast, robust, low-input ChIP-seq for histones and transcription factors. Nat. Methods 12 , 963–965 (2015).
Patel H, et al. nf-core/chipseq: nf-core/chipseq v1.2.1 – Platinum Mole (1.2.1). Zenodo https://doi.org/10.5281/zenodo.3966161 (2020).
Corces, M. R. et al. An improved ATAC-seq protocol reduces background and enables interrogation of frozen tissues. Nat. Methods 14 , 959–962 (2017).
Patel, H. et al. nf-core/atacseq: nf-core/atacseq v1.2.1 – Iron Centipede (1.2.1). Zenodo https://doi.org/10.5281/zenodo.3965985 (2020).
Krietenstein, N. et al. Ultrastructural details of mammalian chromosome architecture. Mol. Cell 78 , 554–565.e7 (2020).
Hsieh, T.-H. S. et al. Resolving the 3D landscape of transcription-linked mammalian chromatin folding. Mol. Cell 78 , 539–553.e8 (2020).
Durand, N. C. et al. Juicer provides a one-click system for analyzing loop-resolution Hi-C experiments. Cell Syst. 3 , 95–98 (2016).
Nora, E. P. et al. Molecular basis of CTCF binding polarity in genome folding. Nat. Commun. 11 , 5612 (2020).
Kaul, A., Bhattacharyya, S. & Ay, F. Identifying statistically significant chromatin contacts from Hi-C data with FitHiC2. Nat. Protoc. 15 , 991–1012 (2020).
Open2C, Abdennur, N. et al. Cooltools: enabling high-resolution Hi-C analysis in Python. PLoS Comput. Biol. 20 , e1012067 (2024).
Lataretu, M. & Hölzer, M. RNAflow: an effective and simple RNA-seq differential gene expression pipeline using Nextflow. Genes 11 , 1487 (2020).
Zhang, M. J. et al. Polygenic enrichment distinguishes disease associations of individual cells in single-cell RNA-seq data. Nat. Genet. 54 , 1572–1580 (2022).
de Leeuw, C. A., Mooij, J. M., Heskes, T. & Posthuma, D. MAGMA: generalized gene-set analysis of GWAS data. PLoS Comput. Biol. 11 , e1004219 (2015).
Cowper-Sal lari, R. et al. Breast cancer risk-associated SNPs modulate the affinity of chromatin for FOXA1 and alter gene expression. Nat. Genet. 44 , 1191–1198 (2012).
Law, P. J. et al. Genome-wide association analysis implicates dysregulation of immunity genes in chronic lymphocytic leukaemia. Nat. Commun. 8 , 14175 (2017).
Ernst, J. & Kellis, M. ChromHMM: automating chromatin-state discovery and characterization. Nat. Methods 9 , 215–216 (2012).
Ernst, J. & Kellis, M. Chromatin-state discovery and genome annotation with ChromHMM. Nat. Protoc. 12 , 2478–2492 (2017).
van der Velde, A. et al. Annotation of chromatin states in 66 complete mouse epigenomes during development. Commun. Biol. 4 , 239 (2021).
Gazal, S. et al. Functional architecture of low-frequency variants highlights strength of negative selection across coding and non-coding annotations. Nat. Genet. 50 , 1600–1607 (2018).
Bentsen, M. et al. ATAC-seq footprinting unravels kinetics of transcription factor binding during zygotic genome activation. Nat. Commun. 11 , 4267 (2020).
Rajasekaran, V. et al. Genetic variation at 11q23.1 confers colorectal cancer risk by dysregulation of colonic tuft cell transcriptional activator POU2AF2. Preprint at bioRxiv https://doi.org/10.1101/2023.08.24.554659 (2023).
Shabalin, A. A. Matrix eQTL: ultra fast eQTL analysis via large matrix operations. Bioinformatics 28 , 1353–1358 (2012).
Stegle, O., Parts, L., Piipari, M., Winn, J. & Durbin, R. Using probabilistic estimation of expression residuals (PEER) to obtain increased power and interpretability of gene expression analyses. Nat. Protoc. 7 , 500–507 (2012).
Liu, J. Z. et al. Meta-analysis and imputation refines the association of 15q25 with smoking quantity. Nat. Genet. 42 , 436–440 (2010).
Gong, J. et al. PancanQTL: systematic identification of cis-eQTLs and trans-eQTLs in 33 cancer types. Nucleic Acids Res. 46 , D971–D976 (2017).
Article PubMed Central Google Scholar
Garcia-Alonso, L., Holland, C. H., Ibrahim, M. M., Turei, D. & Saez-Rodriguez, J. Benchmark and integration of resources for the estimation of human transcription factor activities. Genome Res. 29 , 1363–1375 (2019).
Türei, D., Korcsmáros, T. & Saez-Rodriguez, J. OmniPath: guidelines and gateway for literature-curated signaling pathway resources. Nat. Methods 13 , 966–967 (2016).
Chen, E. Y. et al. Enrichr: interactive and collaborative HTML5 gene list enrichment analysis tool. BMC Bioinformatics 14 , 128 (2013).
Download references
Acknowledgements
At the Institute of Cancer Research (ICR), this work was supported by Cancer Research UK (CRUK) (C1298/A25514 to R.S.H.), the Wellcome Trust (214388) and the ICR Genomics Facility, which performed the sequencing. In Edinburgh, this work was supported by funding from CRUK (DRCPGM/100012 and C348/A12076 to M.G.D.), as well as the Cancer Research UK Scotland Centre in Edinburgh (CTRQQR‑2021/100006 to M.G.D.), which provided infrastructure and staffing. B.T.H. was supported by a CRUK Ph.D. studentship, which was supervised by S. Farrington, at the Edinburgh CRUK Cancer Research Centre. We thank M. Schubach and M. Kircher for assistance in adapting the MPRAflow pipeline. We also thank M. Went, A. Gunnell and A. Everall for technical and statistical input. Figure 1 was created using BioRender.com .
Author information
Bradley T. Harris
Present address: Wellcome Sanger Institute, Hinxton, UK
Authors and Affiliations
Division of Genetics and Epidemiology, The Institute of Cancer Research, Sutton, UK
Philip J. Law, James Studd, James Smith, Jayaram Vijayakrishnan, Maria Mandelia, Charlie Mills & Richard S. Houlston
Colon Cancer Genetics Group, Edinburgh Cancer Research Centre, Institute of Genetics and Cancer, University of Edinburgh, Edinburgh, UK
Bradley T. Harris & Malcolm G. Dunlop
You can also search for this author in PubMed Google Scholar
Contributions
P.J.L. and R.S.H. designed and planned the study. P.J.L., C.M. and B.T.H. performed bioinformatic analysis. J. Studd, J. Smith, J.V. and M.M. performed experiments and analyzed data. P.J.L. integrated analyses and interpreted data. P.J.L. and R.S.H. wrote the manuscript. R.S.H. and M.G.D. provided supervision. All authors have read and approved the final version of the manuscript.
Corresponding author
Correspondence to Richard S. Houlston .
Ethics declarations
Competing interests.
The authors declare no competing interests.
Peer review
Peer review information.
Nature Genetics thanks Luke Jostins-Dean and the other, anonymous, reviewer(s) for their contribution to the peer review of this work. Peer reviewer reports are available.
Additional information
Publisher’s note Springer Nature remains neutral with regard to jurisdictional claims in published maps and institutional affiliations.
Extended data
Extended data fig. 1 detailed schematic of the analysis..
Detailed schematic of the analysis performed. Using the loci identified by the CRC GWAS, we annotated the regions using multiple functional modalities including massively parallel reporter assays (MPRA) to observe allelic effects on transcription, epigenetic marks (ChIP-seq), chromatin accessibility (ATAC-seq), gene expression (RNA-seq) and long-range chromatin interactions (Micro-C). ABC: Activity By Contact.
Extended Data Fig. 2 Detailed annotation for the variants in 8q24 locus.
Detailed functional annotation for the variants in 8q24 locus from UCSC Genome Browser, showing the Micro-C, chromHMM, ATAC-seq, and ChIP-seq data across the various cell lines. The putative variant, rs6983267 , is highlighted in light blue (left). A secondary signal at rs4733767 is also shown (middle blue line).
Extended Data Fig. 3 Detailed annotation for the variants in 10p12 locus.
Detailed annotation for the variants in 10p12 locus from UCSC Genome Browser. The putative variant, rs1248418 , is highlighted in light blue.
Extended Data Fig. 4 Detailed annotation for the variants in 1p34 locus.
Detailed annotation for the variants in 1p34 locus from UCSC Genome Browser. The putative variant, rs67631072 , is highlighted in light blue.
Supplementary information
Supplementary information.
Supplementary Methods and Supplementary Figs. 1–10
Reporting Summary
Peer review file, supplementary tables.
Supplementary Tables 1–12
Source Data Fig. 2
List of each tested SNP and their assigned tier
Source Data Fig. 3
List of each SNP in the example regions with their functional annotations
Source Data Fig. 4
List of genes and their assigned functional category
Rights and permissions
Open Access This article is licensed under a Creative Commons Attribution 4.0 International License, which permits use, sharing, adaptation, distribution and reproduction in any medium or format, as long as you give appropriate credit to the original author(s) and the source, provide a link to the Creative Commons licence, and indicate if changes were made. The images or other third party material in this article are included in the article’s Creative Commons licence, unless indicated otherwise in a credit line to the material. If material is not included in the article’s Creative Commons licence and your intended use is not permitted by statutory regulation or exceeds the permitted use, you will need to obtain permission directly from the copyright holder. To view a copy of this licence, visit http://creativecommons.org/licenses/by/4.0/ .
Reprints and permissions
About this article
Cite this article.
Law, P.J., Studd, J., Smith, J. et al. Systematic prioritization of functional variants and effector genes underlying colorectal cancer risk. Nat Genet (2024). https://doi.org/10.1038/s41588-024-01900-w
Download citation
Received : 09 October 2023
Accepted : 07 August 2024
Published : 16 September 2024
DOI : https://doi.org/10.1038/s41588-024-01900-w
Share this article
Anyone you share the following link with will be able to read this content:
Sorry, a shareable link is not currently available for this article.
Provided by the Springer Nature SharedIt content-sharing initiative
Quick links
- Explore articles by subject
- Guide to authors
- Editorial policies
Sign up for the Nature Briefing: Translational Research newsletter — top stories in biotechnology, drug discovery and pharma.

For the best browsing experience please enable JavaScript. Instructions for Microsoft Edge and Internet Explorer , other browsers
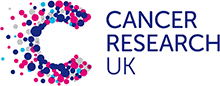
- About cancer
- Get involved
- Our research
- Funding for researchers
- Cancer types
- Cancer in general
- Causes of cancer
- Coping with cancer
- Health professionals
- Do your own fundraising
- By cancer type
- By cancer subject
- Our funding schemes
- Applying for funding
- Managing your research grant
- How we deliver our research
- Find a shop
- Shop online
- Our eBay shop
- Our organisation
- Current jobs
- Cancer news
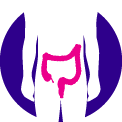
Bowel cancer
Bowel cancer means cancer that starts in the large bowel (colon) and the back passage (rectum). It is also known as colorectal cancer. Your treatment depends on where the cancer starts in your bowel.
The bowel is split into 2 parts - the small bowel and the large bowel. The large bowel includes the colon, the rectum and the bowel opening (anus).
This section is about colon and rectal cancer. We have separate sections about anal cancer and small bowel cancer.
- Find out about anal cancer
- Find out about small bowel cancer
What is bowel cancer?
Bowel cancer is also called colorectal cancer. It affects the large bowel, which is made up of the colon and rectum.
Symptoms of bowel cancer
Symptoms of bowel (colorectal) cancer can include bleeding from the back passage, blood in your poo or a change in your normal bowel habit.
Risks and causes of bowel cancer
The risk factors for bowel (colorectal) cancer include your age, family history, lifestyle factors and other medical conditions.
Getting diagnosed
You usually start by seeing your GP and they might refer you to a specialist and organise tests. Find out more, and read about screening for bowel cancer.
Stages, types and grades of bowel cancer
The stage of a cancer tells you how far it has grown through the bowel wall and whether it has spread to nearby lymph nodes or other organs. It helps your doctor to know which treatment you need.
Treatment depends on whether you have colon or rectal cancer, as well as your cancer stage. You might have surgery, radiotherapy or chemotherapy or a combination of these.
Survival for bowel cancer
Survival depends on many factors including the stage of your bowel cancer when you are diagnosed and how it has responded to treatment. The figures for bowel cancer survival can only be used as a general guide.
Research and clinical trials
All treatments must be fully researched before they can be adopted as standard treatment for everyone. Find out about research into bowel (colorectal) cancer, as well as how to take part in clinical trials.
Living with bowel cancer
Getting practical and emotional support can help you cope with your diagnosis, and life during and after treatment.
Advanced bowel cancer
Advanced bowel (colorectal) cancer means cancer that has spread outside your bowel to other parts of your body. Treatment includes chemotherapy, surgery, targeted cancer drugs (biological therapies) and radiotherapy.
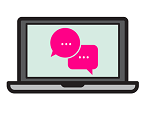
It’s a worrying time for many people and we want to be there for you whenever - and wherever - you need us. Cancer Chat is our fully moderated forum where you can talk to others affected by cancer, share experiences, and get support. Cancer Chat is free to join and available 24 hours a day.
Visit the Cancer Chat forum

About Cancer generously supported by Dangoor Education since 2010.
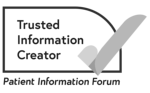
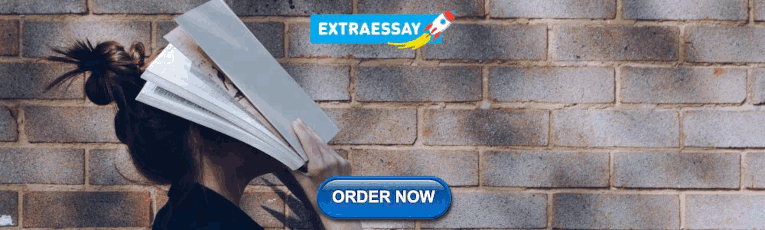
Find a clinical trial
Search our clinical trials database for all cancer trials and studies recruiting in the UK

Cancer Chat forum
Talk to other people affected by cancer

Nurse helpline 0808 800 4040
Questions about cancer? Call freephone 9 to 5 Monday to Friday or email us

An official website of the United States government
The .gov means it’s official. Federal government websites often end in .gov or .mil. Before sharing sensitive information, make sure you’re on a federal government site.
The site is secure. The https:// ensures that you are connecting to the official website and that any information you provide is encrypted and transmitted securely.
- Publications
- Account settings
The PMC website is updating on October 15, 2024. Learn More or Try it out now .
- Advanced Search
- Journal List
- Front Oncol
- PMC11387156
Editorial: Emerging mutations in colorectal cancer development and progression
Colorectal cancer (CRC) is one of the most common malignancies and causes of cancer-related deaths, being the third most common cancer and the second most common cause of cancer death worldwide. GLOBOCAN estimated that in 2022 CRC constituted 9.6% of the approximately 2.5 million cancers diagnosed worldwide, as well as 9.3% of the approximately 1.8 million cancer deaths worldwide ( 1 ). CRC is primarily sporadic in nature although about 3-4% of patients have germline mutations as part of the Lynch syndrome (previously known as hereditary non-polyposis coli) or familial polyposis coli (APC). Sporadic CRC may be in part related to lifestyle risk factors, especially diets high in fat and low in fiber, as well as lack of exercise, obesity, alcohol consumption, and tobacco smoking. It is estimated that by the year 2035, the total number of deaths from colon and rectal cancer will increase by 71.5% and 60%, respectively. Overall survival (OS) at 5 years after primary diagnosis in stage I–II patients is about 87-90%, decreasing to about 68–72% in stage III patients, and further dropping to about 11–14% in stage IV metastatic CRC (mCRC). Although CRC has primarily been a disease seen in high-income countries (HICs), it is rapidly increasing in low-middle-income countries (LMICs) possibly related to dietary changes to so-called Western diets, high in fat and low in fiber, and yet unknown gene mutations. Indeed, CRC also seems to be being diagnosed at younger ages in LMICs with mean ages of about 10 years younger than in HICs, especially in patients of African descent ( 2 , 3 ).
CRC is a clinical and etiologically heterogeneous disease that is characterized by clinical, diagnostic, and therapeutic differences based on tumor-sidedness probably related to its embryologic origin, from either the midgut or the hindgut, as well as various germline and somatic molecular mutations in global gene expression. Studies have found that the cumulative impact of genetic and epigenetic mutations in tumor suppressor genes, oncogenes, and DNA mismatch-repair (MMR) genes may be a potential precursor to the onset of the disease, the response to treatment, as well as its progression and outcome ( 4 – 6 ).
Germline mutations and deletions in the APC gene, and DNA MMR genes including MLH1, MSH2, MSH6, and PMS2, which result in increased microsatellite instability (MSI-H) have been highlighted to play a key role in the etiology of CRC and result in a predisposition to the disease in some families, particularly those with the Lynch syndrome. Somatic mutations of DNA MMR genes also result in increased (MSI-H) in about 12% of CRC tumors. The remaining 85% of CRCs are however microsatellite stable (MSS) as their DNA repair genes are proficient. Genetic alterations have been found to influence the dysregulation of various signaling pathways which lead to drug resistance, inhibition of apoptosis, and progression of tumor invasion and proliferation leading to colorectal tumor growth and metastasis, as well as resistance to both chemotherapeutic and targeted treatments.
Somatic mutations of EGFR-1 signal transduction pathway genes, especially of the RAS family kinases, especially KRAS and NRAS, as well as BRAF kinases, as well as of MEK and ERK/MAPK, appear to be important in tumorigenesis and cancer progression. In addition, alterations in the PI3K/PTEN/AKT/mTOR pathway, especially of PI3K and PTEN, also appear to play an important role in CRC development and progression, associated with resistance to treatment.
Today therapeutic algorithms for CRC contain endoscopic and surgical resection, systemic adjuvant chemotherapy, radiation therapy, palliative chemotherapy, targeted therapy, including monoclonal antibodies and signal-transduction kinase inhibitors, and immunotherapy. Due to the poor outcomes of numerous colorectal patients to existing therapeutic approaches and since CRC survival is highly dependent on primary diagnosis, staging, and early treatment, known significant biomarkers that can predict beneficial responses as early as possible are critical. In addition to biomarkers guiding early diagnosis and treatment, monitoring of these biomarkers during the course of the disease is essential in evaluating the response to treatment and helping treatment decision-making.
In patients with metastatic CRC (mCRC), 5-fluorouracil (5FU)/irinotecan/oxaliplatin-based chemotherapy (FOLFIRI or FOLFOX) in combination with either EGFR-1 or VEGF targeted therapy has improved median overall survival (OS) from less than 1 year before the year 2000 to 2 years or more today.
The addition of cetuximab, a chimeric IgG1 anti-EGFR-1 monoclonal antibody, to 5FU/irinotecan-based chemotherapy (FOLFIRI) in newly diagnosed mCRC patients increased the median progression-free survival from 8.4 months to 9.9 months (HR 0.70; 95% CI 0.56-0.87; p=0.0012) and the median overall survival from 20 months to 23.5 months (HR 0.80; 95% CI 0.67-0.95; p=0.0094) in KRAS exon 2 wild-type patients (~45% of patients) but showed no benefit in the KRAS mutant patients ( 4 ).
The addition of panitumumab, a fully human IgG2 anti-EGFR-1 monoclonal antibody to 5FU/oxaliplatin-based chemotherapy (FOLFOX) in newly diagnosed mCRC increased the median progression-free survival (PFS) from 7.9 months to 10.1 months (HR 0.72; 95% CI 0.58-0.90; p=0.004) and the median overall survival (OS) from 20.2 months to 26 months (HR 0.78; 95% CI 0.62-0.99; p=0.04) in RAS wild-type patients, with 17% of the KRAS exon 2 wild-type patients having other RAS mutations. The addition of panitumumab to FOLFOX chemotherapy in RAS mutant patients however showed a negative benefit in both PFS and OS ( 5 ).
The addition of pembrolizumab, a humanized anti-PD1 monoclonal antibody to chemotherapy in mCRC showed ( 6 ).
Until recently it has been very difficult to follow the development and implications of such mutations as well as their relationship to disease outcomes, due to the difficulties and high risks of repeated biopsies to obtain new tumor tissue. The advent of “liquid biopsies” with the ability to analyze nanogram amounts of circulating cell-free tumor DNA (cfDNA/ctDNA) has made it now possible to follow patients’ disease course and outcomes and correlate them with molecular changes ( 7 ).
Recent studies have shown a correlation between CRC disease outcomes and the emergence of new mutations of EGFR-1 signaling pathway genes with a relationship between higher mutant allele frequency and poorer patient outcomes. In addition, emergent mutations of the extracellular ligand-binding domain may negatively affect the binding of anti-EGFR-1 monoclonal antibodies to specific epitopes in this domain ( 8 , 9 ).
This Research Topic aims to generate a discussion regarding the emergence of mutations of various components of the EGFR-1 and other signaling pathways in the field of colorectal cancer, how it influences the disease, and the impact it has on patient outcomes.
Author contributions
PR: Conceptualization, Visualization, Writing – original draft, Writing – review & editing.
Conflict of interest
The author declare that the research was conducted in the absence of any commercial or financial relationships that could be construed as a potential conflict of interest.
The author(s) declared that they were an editorial board member of Frontiers, at the time of submission. This had no impact on the peer review process and the final decision.
Publisher’s note
All claims expressed in this article are solely those of the authors and do not necessarily represent those of their affiliated organizations, or those of the publisher, the editors and the reviewers. Any product that may be evaluated in this article, or claim that may be made by its manufacturer, is not guaranteed or endorsed by the publisher.
- Open access
- Published: 13 September 2024
Discovery of vitexin as a novel VDR agonist that mitigates the transition from chronic intestinal inflammation to colorectal cancer
- Yonger Chen 1 , 2 na1 ,
- Jian Liang 2 na1 ,
- Shuxian Chen 3 na1 ,
- Nan Lin 3 ,
- Shuoxi Xu 2 ,
- Jindian Miao 2 ,
- Jing Zhang 2 ,
- Chen Chen 2 ,
- Xin Yuan 4 ,
- Zhuoya Xie 5 ,
- Enlin Zhu 6 ,
- Mingsheng Cai 1 ,
- Xiaoli Wei 5 ,
- Shaozhen Hou 2 &
- Hailin Tang 5
Molecular Cancer volume 23 , Article number: 196 ( 2024 ) Cite this article
Metrics details
Colitis-associated colorectal cancer (CAC) frequently develops in patients with inflammatory bowel disease (IBD) who have been exposed to a prolonged state of chronic inflammation. The investigation of pharmacological agents and their mechanisms to prevent precancerous lesions and inhibit their progression remains a significant focus and challenge in CAC research. Previous studies have demonstrated that vitexin effectively mitigates CAC, however, its precise mechanism of action warrants further exploration. This study reveals that the absence of the Vitamin D receptor (VDR) accelerates the progression from chronic colitis to colorectal cancer. Our findings indicate that vitexin can specifically target the VDR protein, facilitating its translocation into the cell nucleus to exert transcriptional activity. Additionally, through a co-culture model of macrophages and cancer cells, we observed that vitexin promotes the polarization of macrophages towards the M1 phenotype, a process that is dependent on VDR. Furthermore, ChIP-seq analysis revealed that vitexin regulates the transcriptional activation of phenazine biosynthesis-like domain protein (PBLD) via VDR. ChIP assays and dual luciferase reporter assays were employed to identify the functional PBLD regulatory region, confirming that the VDR/PBLD pathway is critical for vitexin-mediated regulation of macrophage polarization. Finally, in a mouse model with myeloid VDR gene knockout, we found that the protective effects of vitexin were abolished in mid-stage CAC. In summary, our study establishes that vitexin targets VDR and modulates macrophage polarization through the VDR/PBLD pathway, thereby alleviating the transition from chronic colitis to colorectal cancer.
• Deficiency in VDR accelerates the progression from chronic colitis to colorectal cancer.
• Vitexin regulates transcription by targeting VDR and upregulating its nuclear expression.
• Vitexin induces M1 polarization of macrophages in the tumor microenvironment via the VDR/PBLD signaling pathway, mitigating the progression from chronic colitis to colorectal cancer in mice.
Introduction
Colorectal cancer (CRC) is one of the leading causes of cancer-related mortality globally, with over 20% of cases linked to chronic inflammation [ 1 ]. Colitis-associated colorectal cancer (CAC) represents a particularly aggressive subtype of CRC that occurs in patients with inflammatory bowel disease (IBD) who have been in a chronic inflammatory environment for a long time [ 2 , 3 ]. The largest cohort study in China showed that homologous recombination pathway gene mutations are another major genetic risk factor for heterogeneous clinical phenotypes of colorectal cancer [ 4 ]. Unlike sporadic CRC, which typically progresses through the adenoma-dysplasia-carcinoma sequence, CAC is characterized by the accumulation of somatic mutations that facilitate the progress transition from inflammatory mucosa to dysplasia to carcinoma [ 5 ], and patients with CAC are often diagnosed at an advanced stage and with a poor prognosis [ 6 ]. Thus far, the management of colorectal cancer has encountered substantial challenges, particularly due to the development of drug resistance in treatments targeting the disease [ 7 , 8 , 9 , 10 ]. Studies have shown that mitochondria in colorectal cancer stem cells are a target for drug resistance [ 11 ]. Inflammatory damage accelerates the onset and progression of CAC [ 12 ]. This inflammatory signaling is mediated through dynamic crosstalk between cancer cells and tumor microenvironment (TME) cells. Therefore, exploring the intrinsic connection between cancer cells and TME cells is also crucial for a better understanding of carcinogenesis.
The TME consists of various types of non-malignant stromal cells, including macrophages, neutrophils, lymphocytes, endothelial cells, and cancer-associated fibroblasts (CAFs). Tumor-associated macrophages (TAMs) play a pivotal role in tumor progression, metastasis, and recurrence following treatment [ 13 , 14 ]. The plasticity and heterogeneity of macrophages allow them to be classified along the M1-M2 polarization axis [ 15 , 16 , 17 ]. Tumor-associated macrophages usually exhibit an M2-like phenotype with pro-tumor functions, whereas M1 macrophages have anti-tumor functions. Consequently, reprogramming TAMs to adopt an M1-like phenotype represents a promising strategy to promote tumor regression [ 18 , 19 ]. Various approaches exist for selecting the M1 phenotype from TAMs or reprogramming TAMs from an M2 to an M1 phenotype, including TLR agonists, monoclonal antibodies targeting M1 phenotype suppressor proteins, and other compounds [ 20 ]. Targeted therapy usies utilizing small molecule compounds can specifically target genes in tumor cells, precisely acting on cancer cells to inhibit proliferation and reduce damage to normal cells, providing new insights for cancer treatment. Numerous studies have demonstrated that small molecule monomers derived from traditional Chinese medicine can exert therapeutic effects by targeting specific proteins. Vitexin, a flavonoid compound, has been reported to possess protective effects on the intestines. Our previous research indicated that vitexin significantly modulated macrophage polarization in the intestines of azoxymethane (AOM)/ dextran sodium sulfate (DSS) mice and exhibited protective effects on the intestines [ 21 ]. Despite significant advancements in our understanding of tumorigenesis, the complexities underlying the interactions between cancer cells and macrophages following vitexin exposure, particularly within the immune microenvironment, remain inadequately elucidated. The role of vitamin D receptors (VDR) in the pathogenesis of colorectal cancer and colitis has been well established. Notably, patients suffering from both inflammatory bowel disease and colorectal cancer often exhibit vitamin D/VDR deficiencies. Early downregulation of VDR has been observed in the onset of colitis, correlating with the development of larger and more numerous tumors in VDR-deficient models of CAC [ 22 , 23 ]. Recent investigations have indicated that vitamin D activity becomes dysregulated in advanced cancer stages, although it is known to modulate the interactions between immune and cancer cells, thereby inhibiting the production of pro-inflammatory cytokines [ 24 ]. Despite extensive research on vitamin D, critical questions regarding the biological role of intestinal VDR during the transition from colitis to CAC remain unresolved. Therefore, it is important to search for novel VDR agonists and thus explore their therapeutic role and mechanisms in inflammatory cancers. Similarly, the changes occurring in macrophages during the transition from inflammatory bowel disease to CAC are complex. Within the tumor microenvironment, both VDR and macrophages are implicated in the progression of CAC, but whether they are closely linked or functionally independent remains unclear. Considering the multiple functional roles of the VDR in the development of CAC, the cellular and molecular mechanisms by which the VDR regulates macrophages and thereby protects the host are important.
In this study, we established a mouse model of chronic colitis progressing to colorectal cancer (mid-term CAC) and demonstrated that myeloid-specific VDR knockout mice exhibit low VDR expression in the colon, which negatively correlates with the presence of M2-type macrophages. We confirmed that vitexin can target VDR and promote its transcriptional functions. We used a co-culture of macrophages and cancer cells to simulate the vitexin regulation of macrophages in the tumor microenvironment in a VDR-dependent manner and found that this regulation is achieved through the VDR/phenazine biosynthesis-like domain-containing protein (PBLD) pathway. In addition, we used a mouse model with myeloid-specific VDR knockout to study the protective effect of vitexin on VDR during inflammation and tumorigenesis. Our findings elucidate novel pathways and candidate therapeutic agents for addressing the transformation of chronic intestinal inflammation into colorectal cancer by regulating the polarization of macrophages in the tumor microenvironment.
Materials and methods
Vitexin (HPLC purity > 98%), and Calcitriol were purchased from Sigma-Aldrich. AOM were purchased from Sigma Aldrich. DSS were purchased from MP Biomedicals. Pronase were purchased from Roche. For in vitro experiments, vitexin was dissolved in DMSO and Calcitriol in water, and for in vivo studies, vitexin was dissolved in 1% carboxymethylcellulose sodium (CMC-Na) solution and Calcitriol in saline. Recombinant human protein VDR was constructed at KMD Bioscience (Tianjin, China) and recombinant human protein VDR-LBD was constructed and sequenced at DetaiBio. (Nanjing, China). APC anti-Mouse F4/80 Antibody and FITC anti-Human/Mouse CD11b Antibody were purchased from MULTI SCIENCES. PE/Cyanine7 anti-mouse CD206 (MMR) Antibody, PE anti-Nos2 (iNOS) Antibody, PE/Cyanine7 anti-human CD206 (MMR) Antibody and PE anti-human CD86 Antibody were purchased from BioLegend. PcDNA3.1(+)-VDR-3×FLAG, pGL4.10-CYP24A1 promoter, Wide-Type, pCMV3-flag-ratVDR, pCMV3-flag-ratVDR- T287A were constructed and sequenced by OBio. (Shanghai, China). Extraction kits for cell membrane and nucleus fractions were performed by beyotime (Shanghai, China).
SPF-grade Lyz2-Cre (JAX 004781) was purchased from the Jackson Laboratory, VDR fl/fl was purchased from GemPharmatech (Jiangsu, China), and Lyz2Cre-VDR fl/fl (VDR ΔMΦ ) mice were generated by crossing VDR fl/fl (VDR flox ) mice with Lyz2-Cre (Figure S2 , Supporting Information). The purchased VDR Fl/wt transgenic male mice will be co-housed with wild-type female C57BL/6J background mice at a ratio of 1:2 for mass breeding. After the newborn mice reach 2 weeks of age, they will be numbered and their tails will be clipped for genetic identification, with the identified PCR primers (Table S1 , Supporting Information).
SPF-grade male C57BL/ 6 J mice (18–22 g) were purchased from Guangzhou University of Chinese Medicine Laboratory Animal Centre. All mice were crossed with C57BL/6 for at least 10 generations. The animals were housed in the SPF-grade laboratory animal room of the Guangzhou University of Chinese Medicine and were given normal feed and free drinking water every day, with a relative humidity of 55 ± 5%, an indoor temperature of 22 ± 2 ℃, and 12 h of light. The bedding was changed every other day. The animals were acclimatized to the laboratory for at least 2 weeks before the start of the study. All animal experiments were conducted and analyzed in a blinded randomization manner.
AOM-DSS induced mid-stage colorectal cancer model
Mice were injected intraperitoneally with 10 mg/kg AOM (A5486, Sigma), followed by two cycles of 1.5% DSS in the drinking water on days 8–15 and 22–29 (referenced to previously published [ 25 ] literature but adapted). Disease progression was monitored by body weight measurements and DAI examination, and dissection was determined on day 36. The methodology for the determination of DAI is described in detail in Supplementary Table 2. At the end of the experiment, mice were executed, colon harvested and colon weight and length measured. Tumor load was quantified post-mortem by macroscopic examination of the colon.
Cell culture
THP-1 (Human Acute Monocytic Leukemia Cells) and RAW 264.7 (Mouse Mononuclear Macrophages Cells), is the most commonly used inflammatory cell model. CT26.WT (Mouse Colorectal Carcinoma Cells) are undifferentiated cells induced by N-nitroso-N-methylurea (NNMU). HCT 116 (Human Colorectal Carcinoma Cells) was isolated from male patients with colon cancer. Human Embryonic Kidney Cells (HEK) 293T cells, used as tool cells for plasmid transfection. All cells were purchased from Procell (Wuhan, China). CT26.WT, HCT116 cells were cultured in RPMI-1640 medium, and RAW264.7, HEK293T were cultured in DMEM medium supplemented with 10% heat-inactivated fetal bovine serum (FBS) and 100 U/ml penicillin-streptomycin. THP-1 cells were cultured in RPMI-1640 supplemented with 10% heat-inactivated FBS and 100 U/ml penicillin-streptomycin, with the addition of 0.05 mM β -mercaptoethanol, and PMA activation (Sigma, USA) THP-1 cells were used as human macrophage studies.
THP-1 cells were transfected with PBLD or control siRNA (Tsingke, China) using Lipofectamine ® 6000 Transfection Reagent (Beyotime, China) in Opti-MEM I Reduced Serum Medium for 72 h. Then, the transfected cells were used for further research.
Cellular RNA sequencing
Total RNA was extracted from THP-1 cells using TRIzol (Accurate Biotechnology, China). Library sequencing was performed by Gene Denovo Biotechnology (Guangzhou, China) on an Illumina HiseqTM 2500/4000. Bioinformatics analysis was performed using Omicsmart, a real-time interactive online platform for data analysis ( http://www.omicsmart.com ).
Gene expression profiles were analyzed by macrophage differentiation PCR arrays (Wcgene Biotech, Shanghai, China) according to the manufacturer’s protocol. β -actin and Gapdh were used as endogenous controls. Data were normalized to the reference gene based on cycle threshold (Ct) values. log2 (fold change) was calculated based on the 2 −ΔΔCt method.
Immunofluorescence staining and immunohistochemistry
The method according to a previous study [ 26 ]. Tissue wax blocks were hydrated by serial dewaxing, while cells were fixed by 4% paraformaldehyde. Then, after permeabilization with 0.05% Triton X-100 and sealing with 5% BSA, the cells were incubated with primary antibody at a dilution of 1:200 at 4 °C overnight. Fluorescently labeled secondary antibodies were used the following day. The stains were then counterstained with DAPI nuclear stain.
The operation of immunohistochemistry is carried out as described above. Following the procedures of primary antibody at a dilution of 1:200 at 4 °C overnight. After incubating with the secondary antibody the next day, DAI staining was performed. The sections were then stained with hematoxylin to visualize nuclei. The final processing steps included dehydration in graded alcohols, clearing in 100% xylene, and mounting. Images were taken with a fluorescence microscope equipped with a digital camera (Nikon, Tokyo, Japan).
Quantitative real-time PCR (qRT-PCR)
The method according to previous studies [ 27 , 28 ]. Total RNA was isolated from cells using TRIzol (Accurate Biotechnology).The concentration of total RNA was determined using a Nanodrop 2000, and 1 µg of total RNA was converted to cDNA by reverse transcription according to the PrimeScript™ RT kit instructions in a CFX96 Touch The target gene was detected in a CFX96 Touch Real-Time RCR Detection System (Bio-Rad) detector using a SYBR Green PCR master mix kit with GAPDH as an internal reference, and the primer sequences of the target gene were designed by Shanghai Sangong Biotechnology Co. The primer sequences of target genes were designed by Shanghai Bioengineering Biotechnology Company. The relative expression of target genes was calculated by using the 2- ΔΔ Ct method for quantification, and the correlation analysis was carried out. Primer sequences are shown in Supplementary Table S3 .
Western blot
The method is according to previous study [ 29 ]. In brief, proteins were isolated from cells and tissues and assayed by BCA assay. Proteins were separated by 8-12% gel electrophoresis separation kit (Beyotime, China) and transferred to polyvinylidene fluoride (PVDF) membranes using a semi-dry transfer apparatus (Bio-Rad). The membranes were blocked with 5% BSA for half an hour, and then the PVDF membranes were incubated with specific primary antibodies. The antibodies used in this study were as follows: VDR (Signalway Antibody, China, Cat#38397, 1:1000); PBLD (Proteintech, China, Cat#68317-1-Ig, 1:1000); PCNA (Affinity, China, Cat#AF0239, 1:1000); GAPDH (Affinity, China, Cat#AF7021, 1:3000); Histone (Affinity, China, Cat#BF9211, 1:3000). Immunoreactive bands were detected the following day with horseradish peroxidase-conjugated secondary antibodies and visualized by enhanced chemiluminescence. Analyses were performed using Image J analysis software and standardized against their respective controls.
Flow cytometry analysis
The method according to a previous study [ 30 ]. Cells were collected and resuspended in 500 µL of phosphate-buffered saline (PBS). Following a 30-minute incubation at room temperature with surface flow antibodies, the cells were centrifuged at 900 rpm for 4 min. The supernatant was discarded, and the cells were washed with PBS before being treated with a cell membrane-disrupting solution (Thermo Fisher, diluted 1:3) for 60 min. Afterward, the cells were washed again in PBS and incubated with polarization-associated flow antibodies for 1 h at room temperature. The cells were subsequently washed and resuspended in PBS containing 1% bovine serum albumin (BSA). Following another wash, the cells were resuspended in PBS with 1% BSA and analyzed using a NovoCyte Quanteon flow cytometer. Data were processed using NovoExpress software, with specific labeling for F4/80, CD11b, iNOS (M1), and CD206 (M2) flow-through antibodies.
VDR binding assay
The binding of vitexin to vdr was determined by several methods.
cellular thermal shift assay (CETSA), drug affinity responsive target stability (DARST), surface plasmon resonance assay (SPR), isothermal titration microcalorimetry assay (ITC), pull-down assay and immunofluorescence co-localization assay.
For CETSA , the CETSA method was performed according to the literature [ 31 ]. THP-1 cells were inoculated in a 100 mm dish and subsequently treated with FBS-free medium containing either 0.1% DMSO or 100 µM vitexin for 2 h the following day. Post-treatment, the cells were digested with trypsin, collected, and washed before being resuspended in 1 mL of PBS supplemented with a protease inhibitor. A 90 µL aliquot of this suspension was transferred into 0.2 mL PCR tubes and subjected to heating in a PCR machine for 3 min at temperatures ranging from 43 to 67 °C. 20 µL of RIPA buffer was added to each tube, and the precipitated proteins were removed by centrifugation at 15,000 × g for 20 min at 4 ℃ after thorough mixing. Subsequent supernatant manipulation was based on general Western blot experiments.
For DARTS , the DARTS method was performed according to the literature [ 32 ]. THP-1 cells were inoculated in a 100 mm dish at an appropriate density. The following day, the cells were harvested and lysed in 500 µL of RIPA buffer containing a protease inhibitor. The resulting lysate was aliquoted equally into 5 Ep tubes and subsequently diluted tenfold in TNC buffer (50 mM Tris-HCl, pH 8.0, 50 mM NaCl, 10 mM CaCl2). The samples were then incubated with 0.1% DMSO and varying concentrations of vitexin (0, 2.5, 5, and 20 µM) for 1 h at room temperature with gentle shaking. The samples were then proteolytically cleaved with protease (2.5 µg/mL) for 10 min. Subsequent operations were based on general Western blot experiments.
For SPR experiments , the interaction between vitexin and VDR (ligand-binding domain, LBD) protein was analyzed using a Biacore T200 system (GE Healthcare, Uppsala, Sweden). Recombinant VDR (LBD) at a concentration of 40 µg/mL was used to achieve non-covalent immobilization on the surface of the activated chip. The final level of immobilized VDR-LBD was approximately 12,000 reaction units (RU). Subsequently, different concentrations of vitexin (ranging from 6.25 µM ~ to 200 µM) were injected at a flow rate of 30 µL/min, and 1× PBST (1.37 M NaCl; 26.8 mM KCL; 81 mM Na 2 HPO 4 ; 17.6 mM KH 2 PO 4 ; Ph7.2-7.4, 0.05% Tween 20) was used as the running buffer. The results were analyzed using Biacore evaluation software (T200 version 1.0) and curves were fitted in 1:1 binding mode.
For the ITC experiments , the potential interaction between vitexin and VDR (LBD) was determined using the Nano-ITC instrument (TA instruments, USA) at 25 °C. A solution of 10 µM VDR (LBD) and 200 µM vitexin were dissolved in phosphate-buffered saline (PBS) containing 5% DMSO, and stirred at 250 rpm. Twenty titrations of 2.5 µL each were performed for each titration experiment. The heat of dilution of VDR protein was determined by titrating it into PBS. Data analysis was performed using the NanoAnalyze software package (TA Instruments). The total heat exchange during each injection of VDR into the vitexin solution was fitted to an independent model with variable parameters.
For pull-down experiments , Beaver-Beads streptavidin and biotinylated vitexin were utilized in this study. Specifically, 100 µL of biotinylated vitexin glycoside was added to 10 µL of streptavidin-agarose beads and incubated for 2 h at 25 °C. Controls included biotin, unbiotinylated vitexin, and untreated beads. The full-length prokaryotic proteins of the VDR were constructed at KMD Bioscience (Beijing, China), while the prokaryotic proteins corresponding to the VDR-LBD were constructed at Detai Bioscience (Nanjing, China). And mutant VDRs (T287) of the LBD structural domain was achieved by transfecting HEK-293 cells with the encoding plasmid under Lipofect 2000. Lysates were prepared from HEK-293 cells of the constructs and then the lysates were mixed into treated streptavidin-agarose beads. The mixture was incubated at 25 °C for 3 h with gentle shaking. The samples were then spun and washed three times. The samples were boiled with 5× loading buffer and loaded onto 10% polyacrylamide gels for western blot analysis.
For immunofluorescence co-localization experiments , THP-1 cells were seeded at a density of (1 × 10 3 cells/well) were inoculated in confocal dishes, and 60 µL of biotin-vitexin (20 µM), and biotin (20 µM) intervened in the incubation for 24 h. The cyto-fluorescence staining process was started. The cells were first washed once with PBS and then fixed with 4% paraformaldehyde for 10 min. the cells were washed three times with PBS, each time for 3 min, and then closed with 5% BSA for 30 min, and diluted with FITC-avidin as well as VDR antibody, and then incubated at 4 ℃ overnight. At the end of the incubation, the cells were washed three times with PBS for 3 min each time, a fluorescent secondary antibody (Alexa Fluor Plus 594) was added, and the cells were incubated for 1 h. The nuclei were washed three times with PBS for 3 min each time, and the nuclei were stained by DAPI for 5 min. After the washing with PBS, the co-localization of the green light and the red light in the cells by laser confocal microscopy was observed.
Molecular docking
The crystal structure of the LBD structural domain (PDB:1QBD) of human VDR was obtained from the Protein Data Bank. Initial structures of ligands and receptors for docking were prepared with MGLTools 1.5.6 (The Scripps Research Institute, CA, USA). Molecular docking was performed with AutoDock Vina 1.0.2. The binding affinity of each docking pose of the oyster glycosides was recovered by the MM/GBSA method in the AmberTools18 software package [ 33 ]. Finally, key residues for protein-ligand interactions were identified based on the breakdown energy calculations for each residue.
ChIP-seq and PCR
THP-1 cells were seeded at a density of 4 × 10 6 in 100 mm dishes. After 24 h, cell attachment was induced by treatment with 100 ng/ml of phorbol 12-myristate 13-acetate (PMA). Subsequently, the cells were preincubated with either dimethyl sulfoxide (DMSO) or vitexin at a concentration of 20 µM for an additional 24 h. Chromatin immunoprecipitation (ChIP) was performed in accordance with the protocol outlined in the Thermo Fisher manual (Catalog No. 26157). Following treatment, THP-1 cells were harvested and crosslinked using 1% paraformaldehyde for 15 min, after which 125 mM glycine was added to quench the crosslinking reaction. Chromatin was sheared into 200–500 bp DNA by sonication using a Biorupter (Diagenode, UCD-200). After reserving 5.0 µl of the sheared chromatin for input to the control samples, the rest of the sheared chromatin was rotationally incubated with 5 µg of VDR (CST, USA, Cat#12550, 1:50) or IgG at 4 °C overnight. The next day, samples were added to protein A/G dynamic beads and incubated at 4 °C for 2 h. The DNA-protein complexes were washed twice with dilution buffer and then eluted from the dynamic beads with SDS buffer. Purified ChIP DNA was obtained after reverse cross-linking, proteinase K digestion, phenol/chloroform extraction, and ethanol precipitation. For ChIP-sequencing, input DNA and ChIP DNA were used to generate sequencing libraries using the Illumina DNA Sample Preparation Kit according to the manufacturer’s protocol. Briefly, the DNA samples were end-repaired, then ligated with barcode adapters, and finally amplified and purified. These DNA libraries were sequenced in an Illumina. nextSeq500 sequencer, based on the 35nt pair-end sequencing protocol. Chromatin immunoprecipitation analyses and RNA sequencing were performed with the help of Wuhan GeneBen Biotechnology Co Ltd (Wuhan, China). The promoter primers Cyp24a1 and PBLD were subsequently constructed and detected by RT-PCR.
Luciferase reporter gene assay
HEK293T cells were seeded in 6-well plates at 1 × 10 5 cells/well, wall-adhered, and transiently transfected with pGL4.10, PGL4.10-CYP24A1, pcDNA3.1-VDR-3×FLAG, and TK-luc using the Lipofectamine 3000 reagent for 6 h. The transfection solution was discarded and added to the high and medium in turn, low concentrations of vitexin (20, 10, and 5 µM) and Calcitriol (50 nM) after induction for 48 h. The transfection solution was washed twice with PBS, and the dual-luciferase reporter gene assay system was used to measure the dual-luciferase activity and to study the effect of vitexin on the transcriptional activation of the CYP24A1 promoter.
Histological assessment
Colon tissues fixed in 4% paraformaldehyde were paraffin-embedded and sectioned were used for histological analysis. The sections were stained with hematoxylin and eosin (H&E) for routine histological evaluation. For immunohistochemistry, sections were deparaffinized and hydrated. Endogenous peroxidase was blocked with 3% H 2 O 2 for 30 min. Sections were then incubated with primary antibody at a dilution of 1:100 overnight at 4 °C. Secondary antibodies were incubated at 1:200 for 1 h at room temperature and immunoreactivity was detected by diaminobenzidine (DAB). All sections were counterstained with hematoxylin.
Statistical analysis
All in vitro data represent at least three independent experiments and in vivo data represent at least six independent experiments. All experimental data are expressed as mean ± SEM (standard error of the mean). Statistical analyses were performed using GraphPad Pro Prism 8.0 (GraphPad, San Diego, CA). One-way analysis of variance (ANOVA) followed by Tukey’s test was used to analyze differences between groups of data. A p value of < 0.05 was considered a significant difference.
VDR plays an important role in the transformation of chronic intestinal inflammation-induced cancer
To systematically and comprehensively identify the key signaling molecules involved in colon cancer, we performed mRNA microarray analysis of intestinal tissues (patients or mice) with CAC and CRC with inflammatory bowel cancer and normal controls and then intersected the previously published dataset to identify differentially expressed genes (DEGs). The results showed that 136 DEGs overlapped across the four datasets. Notably, we found that Vdr expression was downregulated in intestinal tissues in these transcriptomic data (Figure S1 , Supporting Information). We next performed modeling of chronic intestinal inflammation to carcinoma [ 25 ]. As illustrated in Fig. 1 A-C, the duration of this experimental study was 36 days. Post-dissection observations revealed that, in comparison to the control group, the colons of mice in the model group exhibited significant shortening, with tumors of varying sizes emerging at the distal end of the intestine, measuring between 1 and 2 cm. This finding is consistent with atypical hyperplasia characteristic of the intermediate stage of inflammation-initiated carcinogenesis. A comprehensive assessment of the Swiss Volume of Pathology, indicated that the morphology of the terminal intestinal tissues in the model group was markedly disordered, featuring severe disruption of the colonic epithelial barrier, extensive infiltration of inflammatory cells, hyperplasia of submucosal lymphocytes accompanied by lymphoid follicle formation, and structural ambiguity. These observations suggest that the colon tissue during the intermediate modeling stage is undergoing a transition from inflammation to cancer. Genome-wide analyses, along with cellular and animal experiments, have demonstrated that VDR proteins play a role in the prevention of IBD and CRC [ 34 ]. As shown in Fig. 1 D, VDR protein expression was significantly reduced in the model group compared to the normal group, which is in line with previous studies. In addition, as shown in Fig. 1 E, the results of the heatmap indicated that the nuclear transcription gene Vdr was significantly decreased compared to the model group. In addition, polarization-related genes such as ARG1 , MRC1 , IL1 , and Nos2 were also significantly elevated, suggesting that macrophages in the middle stage of CAC were significantly polarized and infiltrated with inflammatory factors (Fig. 1 F). Meanwhile, macrophage-polarized expression of colonic fluorescence was consistent with transcriptome results.

VDR plays an important role in the transformation of chronic intestinal inflammation-induced cancer. A ) AOM/DSS induced mid-cycle CAC modeling protocol. B ) Macroscopic view of colon obtained by mid-stage CAC, Scale bar, 1 cm. C ) H&E staining of the colon, Scale bar, 200 μm. D ) Immunohistochemistry of VDR protein and statistics in colon (Scale bar, 100 μm, ** P < 0.01). E ) Heatmap of the colon. F ) Immunofluorescence double staining of iNOS and CD206 protein in colon tissue, Scale bar, 100 μm. G ) Schematic diagram of the experimental design of VDR ΔMΦ mice. H ) Body weight in the mice, n = 6. I ) DAI scores, n = 6. J ) macroscopic image of the colon. K ) H&E staining of the colon. The data are presented as the mean ± SEM ( n = 3). ## P < 0.01 vs. WT group
Most reports suggest that intestinal conditional knockout of host VDR significantly increased tumor formation. We next explored the immunosuppressive role of VDR in the development of murine myeloid knockouts, the construction process of the knockout mouse is shown in Fig. 1 G. Western blot analysis showed that colonic tissues of VDR knockout animals displayed a loss of VDR (Figure S2 and S3 , Supporting Information), suggesting a successful knockout animal model. We then recorded changes in body weight and DAI in AOM/DSS mice (Fig. 1 H-I). As expected, VDR deletion (VDR ΔMΦ ) resulted in significantly slower body weight recovery in AOM/DSS mice, along with significantly higher DAI scores, compared to AOM/DSS controls (WT) and caged controls (VDR flox ). Further observation of micro- and macro-colonic tissues showed that the VDR ΔMΦ group showed more pronounced colonic barrier disruption and inflammatory infiltration compared to the control group (Fig. 1 J-K). These results suggest that VDR plays an important role in the transformation of chronic intestinal inflammation-induced cancer.
Vitexin targets VDR and binds amino acid motifs in its VDR-LBD region to regulate its nuclear transcription
We further investigated whether vitexin could act on the VDR. Cellular thermal shift assay (CETSA), a method for assessing the direct binding of drugs in cells, showed that vitexin, an anti-inflammatory flavonoid, increased the stability of the VDR protein in cells when subjected to a temperature gradient (Fig. 2 A). In addition, DARTS experiments showed that vitexin could stabilize the VDR, leading to its increased susceptibility to proteolysis (Fig. 2 B). These observations support the possibility that the VDR may serve as a potential cellular target for vitexin. To further confirm the direct binding of vitexin to the VDR, we synthesized a biotin-labeled vitexin probe (Fig. 2 C, Figure S4 - S6 , Supporting Information), and performed immunofluorescence experiments to explore the co-localization of the VDR and biotin-vitexin in THP-1 cells. As shown in Fig. 2 D, the VDR (red) showed a clear fluorescence overlap (yellow) with biotin-vitexin (green), suggesting a direct vitexin-VDR interaction in the cells. Altogether, these results suggest that VDR is a direct cellular target of vitexin. Therefore, we further investigated how it regulates VDR. Since the reported small-molecule VDR agonists all bind to the LBD structural domain of the VDR, we suspected that this structural domain might be the site of the vitexin interaction. To verify this experimentally, we constructed prokaryotic proteins of the full-length VDR and the LBD structural domain of the VDR (Figure S7 , Supporting Information). Immunoprecipitation pull-down confirmed that biotinylated vitexin interacts with the LBD structural domain of the VDR (Fig. 2 E, Figure S8 , Supporting Information). Next, we used surface plasmon resonance (SPR) to show that vitexin and VDR-LBD interact with an association rate constant (K D ) of 34.67 µM (Fig. 2 F). Finally, we performed isothermal titration calorimetry (ITC) analysis and found that vitexin and VDR-LBD interacted with a binding K D of 21.07 × 10 − 5 µM (Fig. 2 G). To further determine the structural stability of the binding complex of vitexin and VDR, we performed 100 ns molecular dynamics simulations to determine whether vitexin could exert allosteric modulation on VDR. The results showed that vitexin was able to tighten the VDR structural domain and adjust its conformation (Fig. 2 H-I, Figure S9 , Supporting Information). In addition, we performed energy decomposition calculations for each of the 11 docking poses in the binding site. We identified 11 residues with low average energies: ALA231, VAL234, SER235, ILE238, MET272, ASN276, THR287, ARG296, THR301, SER306, and ILE314 (Fig. 2 J). Of these, we selected THR287 for further study based on their energy values. We transfected HEK 293T cells with wild-type VDR or mutant T287A. Biotinylated vitexin pulled down the wild-type VDR but failed to interact with the mutant VDR (Fig. 2 K). These results suggest that THR287 in the LBD structural domain is essential for the interaction of VDR with vitexin.
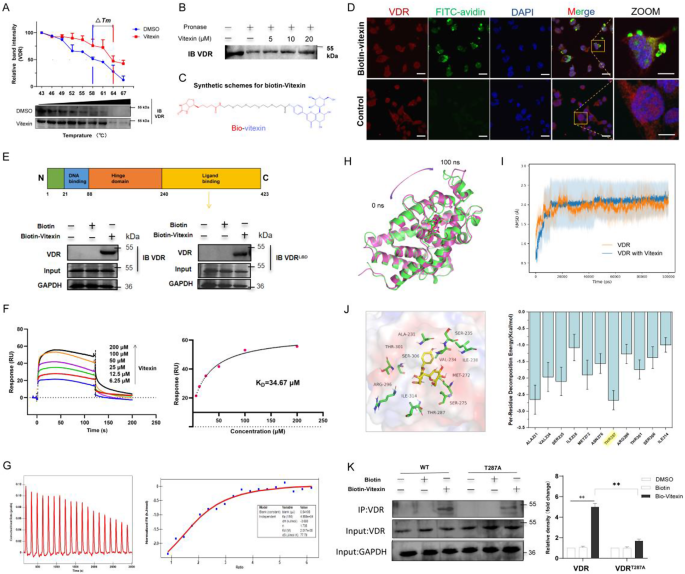
Vitexin targets VDR and binds amino acid motifs in its VDR-LBD region. A ) Vitexin promotes the resistance of VDR to different temperature gradients as detected by CETSA in THP-1 cells. B ) Vitexin enhances the resistance of VDR to proteolytic enzymes as investigated by DARTS. C ) Chemical structure of Biotin-vitexin (Bio-vitexin). D ) Co-localization of biotin-vitexin (green) and VDR (red) by immunofluorescence, Scale bar, 10 μm. E ) Protein blotting analysis of biotin-vitexin binding to the VDR-LBD structural domain. Recombinant proteins VDR and VDR-LBD structural domains were incubated with either biotin- vitexin-loaded magnetic beads or biotin-loaded magnetic beads. The top panel shows the structure of VDR and the bottom panel shows the results of the pulled-down proteins. F ) SPR analysis showing the interaction between vitexin and recombinant VDR-LBD protein (left). Different concentrations of vitexin were added and K D values were calculated (right). G ) ITC analysis of VDR-LBD binding to vitexin, representative images are shown. Representative titration temperature plots are shown on the left, and data integration with the fitted curve (independent model) of vitexin versus VDR-LBD is shown on the right. H ) The presentative conformation of VDR protein binding with vitexin upon molecular dynamics simulation. I ) free energy landscape. J ) The left panel shows the carbon atoms of the side chains of the 11 key residues, with vitexin indicated as green and yellow bars, respectively. The right panel shows a box plot of the per-residue catabolic energy for the 11 residues. K ) Pull-down analysis of biotin-vitexin binding to mutant VDRs containing T287A. HEK 393T cells were transfected with wild-type VDR or mutants. Lysates were used to assay for binding to biotin-vitexin. The data are presented as the means ± SEM ( n = 3). **, P < 0.01; ns, no significance
The VDR protein is implicated in both classical and non-classical signaling pathways, exerting effects through nuclear transcription as well as cytoplasmic interactions. To further elucidate the nuclear import effects of vitexin following VDR targeting, we conducted validation experiments using murine and human-derived macrophages. As illustrated in Fig. 3 A, gradient concentrations of both vitexin and calcitriol resulted in an increase in Vdr mRNA levels. Additionally, Fig. 3 B presents immunofluorescence data demonstrating the subcellular distribution of VDR protein in the cytoplasm and nucleus following drug treatment. The results indicate that both vitexin and calcitriol significantly enhance VDR protein levels within the nucleus; however, vitexin treatment did not produce a notable increase in cytoplasmic VDR levels, instead promoting its accumulation in the nucleus. We further isolated cytoplasmic and nuclear proteins, confirming that treatment with vitexin and calcitriol facilitated the translocation of VDR proteins into the nucleus (Fig. 3 C). In order to measure transcriptional effect, the transcription factor expression plasmid VDR and its CYP24A1 promoter plasmid, were co-transfected into the HEK293T cell. As seen from Fig. 3 D, compared with the vector group, the transcription factor VDR was able to activate the target promoter CYP24A1, which was significantly increased after the stimulation by adding increasing concentrations of vitexin and calcitriol, indicating that vitexin enhances the transcriptional activity of VDR onto CYP24A1 (Figure S14 A-B, Supporting Information). Consistent with the above results, the results of ChIP-PCR showed that the assay results illustrated that both vitexin and calcitriol significantly elevated the expression of CYP24A1 (Fig. 3 E). The above experiments confirmed that vitexin binds to amino acid motifs in the VDR-LBD region to enhance the nuclear translocation of VDR, and increases its transcriptional activities.
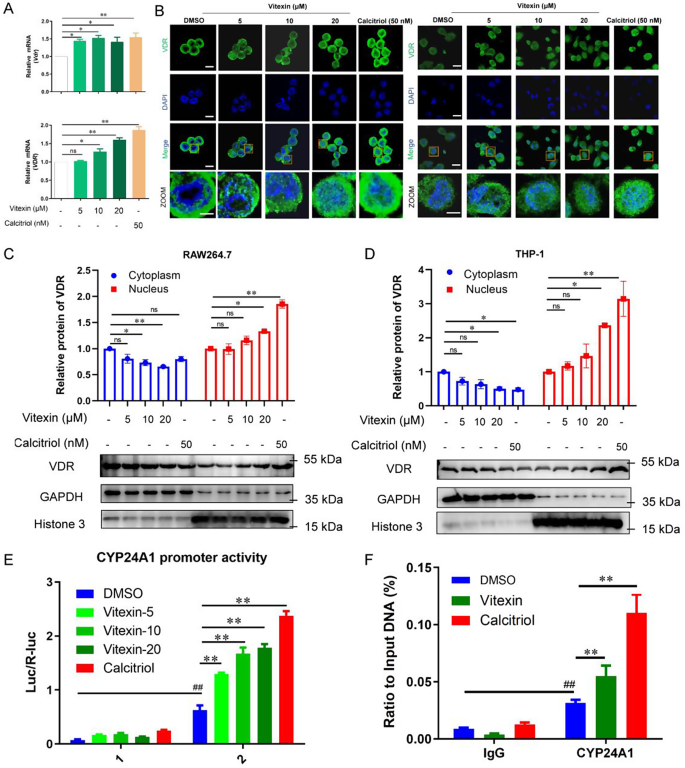
Vitexin promotes nuclear translocation of VDR protein in macrophages and regulates nuclear transcription. Both RAW264.7 and THP-1 cells were treated with different concentrations of vitexin and Calcitriol. A ) Gene levels of VDR in RAW264.7 cells (top) and THP-1 cells (bottom) were detected by RT-PCR. B ) Immunofluorescence was used to examine the levels of VDR in the nucleus and cytoplasm, RAW264.7 cells (left) and THP-1 cells (right). Scale bar, 10 μm. C-D ) Western blot was used to examine the levels of VDR in the nucleus and cytoplasm. E ) Detection of transcription factor VDR against CYP24A1 promoter activity using dual luciferase in HEK293T cells. F ) ChIP-PCR amplification showing VDR activity against the promoter of CYP24A1. The data are presented as the means ± SEM ( n = 3). *, P < 0.05; **, P < 0.01; ns, no significance
In the tumor microenvironment, vitexin promotes VDR entry into the nucleus
We subsequently investigated the role of vitexin within the tumor microenvironment. As illustrated in Fig. 4 A, we utilized bone marrow-derived macrophages (BMDMs) co-cultured with the homologous intestinal cancer cell line CT26 to simulate the tumor microenvironment for subsequent intervention experiments. Figure 4 B demonstrates that following co-culture, the expression of the Vdr gene in the simulated tumor microenvironment was significantly diminished compared to the control group, which aligns with the observed reduction of colon VDR levels in the animal model. PCR analysis revealed a marked decrease in Vdr gene expression after co-culture relative to the normal group; however, treatment with vitexin resulted in a dose-dependent increase in Vdr expression (Fig. 4 C). In addition, our results demonstrated that the VDR levels in the cytoplasm and nucleus were reduced after co-culture when compared to the normal group and that the VDR protein in the nucleus was increased in a concentration-dependent manner after the treatment with of vitexin (Fig. 4 D). These results were further confirmed by immunofluorescence (Fig. 4 E). Together our results suggest that the expression of VDR is significantly reduced in the tumor microenvironment and that the level of VDR in the nucleus increased significantly following treatment with of vitexin.
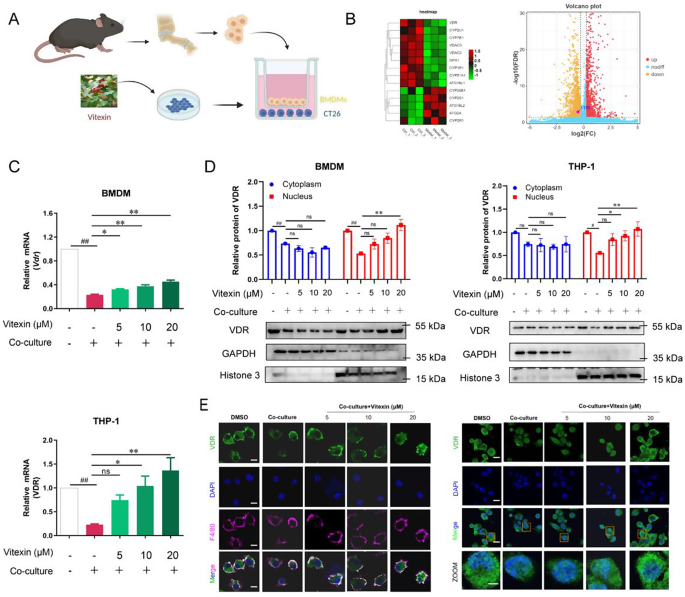
In the tumor microenvironment, vitexin promotes the nuclear translocation of VDR. A ) After 48 h incubation with vitexin, CT26 was co-cultured with BMDM for 24 h, which was at the transwell insert, and CT26 was located at the bottom of the cell plate. The experiments related to this section are all co-culture assays. B ) Left: RNA-seq analysis of THP-1 cells (THP-1 and HCT116) co-cultured with or without treatment with vitexin of differential genes ( n = 3). Right: volcano plot of differential gene enrichment. C ) Gene levels of VDR in BMDM cells (top) and THP-1 cells (bottom) were detected by RT-PCR. D ) Western blot was used to examine the levels of VDR in the nucleus and cytoplasm. E ) Immunofluorescence was used to examine the levels of VDR in the nucleus and cytoplasm. Scale bar, 10 μm. The data are presented as the means ± SEM ( n = 3). # , P < 0.05; ## , P < 0.01; *, P < 0.05; **, P < 0.01; ns, no significance
Vitexin regulates polarization in macrophages in the tumor microenvironment, dependent on VDR
Previous study have confirmed that vitexin upregulates the expression of the M1 type of macrophages in intestinal tissues thereby mitigating intestinal cancer [ 21 ]. However, the impact of vitexin on macrophage regulation during the transition from chronic intestinal inflammation to cancer has not been demonstrated. To address this gap, we employed a co-culture system of tumor cells and macrophages to simulate the tumor microenvironment, allowing for a more detailed investigation of the regulatory effects of vitexin on macrophages within this context (Fig. 5 A). As shown in Fig. 5 B, after treatment with vitexin, we detected macrophage-related genes by real-time PCR and compared the differentially expressed genes in the model or vitexin. As shown in the heatmap, the mRNA levels of CD163 , IL1B , CCL2 , IL6 , and TNF were significantly increased, while the mRNA level of MRC1 was significantly decreased, suggesting that vitexin promotes M1 polarization of macrophages in the tumor microenvironment. In addition, we examined the mRNA levels of Nos2 , Ccl-2 , Il-1b , and Il-6 in RAW264.7, and the results were consistent with the above trend (Figure S13 , Supporting Information). Consistent with the PCR results, the results of flow cytometry also showed that in the tumor microenvironment, vitexin regulated macrophage M1 polarization in a dose-dependent manner, while M2-polarised macrophages were significantly decreased (Fig. 5 C-D). Next, we used VDR knockdown macrophages to reverse validate the role of vitexin in regulating macrophages (Fig. 5 E). It was found by flow cytometry results (Fig. 5 F-G) that the macrophage-regulating effect of vitexin was eliminated in the VDR-knockdown cell model, however, the control treatment group was expressed consistent with the above results. Meanwhile, there was no significant change in the mRNA levels of Nos2 and Arg-1 by vitexin in BMDM cells with VDR knocked down (Fig. 5 H), suggesting that vitexin could regulate macrophage polarization but is dependent on VDR.
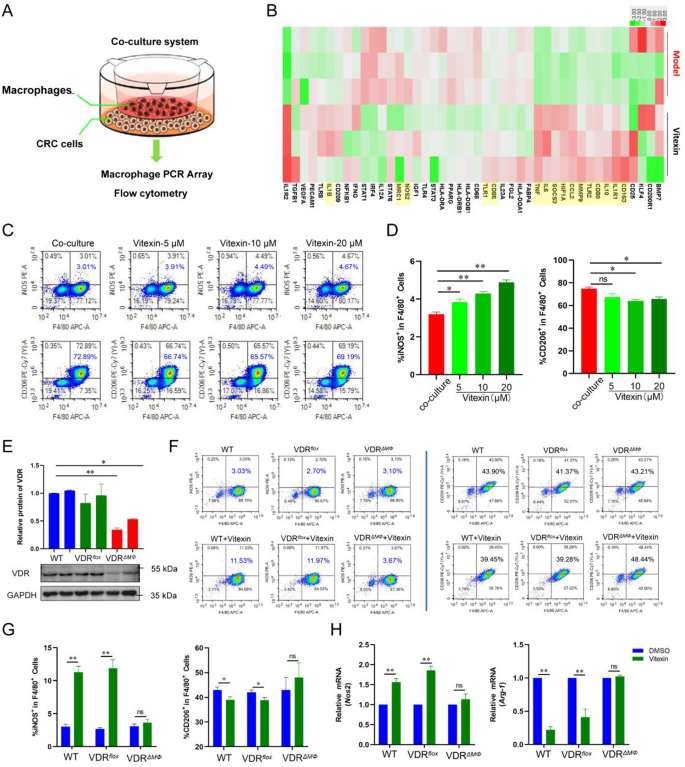
Vitexin regulates polarization in macrophages in co-culture, dependent on VDR. A ) Schematic diagram of the in vitro Transwell-based coculture system. B ) Heatmap of gene expression levels detected in macrophage arrays of co-cultured THP-1 cells for 24 h with or without vitexin treatment ( n = 3 each). C ) Data are presented as representative FACS plots. D ) Flow cytometry analysis of iNOS or CD206 levels in BMDM cells from WT mice. E ) Western blot detection of VDR expression in BMDM from WT, VDR flox , and VDR ΔMΦ . F ) Flow cytometry analysis of iNOS or CD206 levels in BMDM cells. G ) Data are presented as representative FACS plots and in summary plots. H ) The levels of Nos2 and Arg-1 mRNA. The data are presented as the mean ± SEM ( n = 3). *, P < 0.05; **, P < 0.01; ns, no significance
Vitexin regulates macrophage polarization in the tumor microenvironment via the VDR/PBLD pathway
Our findings suggest that Vitexin can regulate macrophage polarization through VDR protein. Next, we further explored which genes are regulated by VDR to regulate macrophage polarization. Co-cultured THP-1 cells using ChIP-Seq data are shown with high-resolution peaks identified in the read density (Fig. 6 A-B, Figure S14 C-D, Supporting Information). Guided by the ChIP-Seq results, further ChIP-PCR experiments were performed to confirm the binding regions. A series of primers were designed for the genomic fragments surrounding the transcription start site. Real-time fluorescence quantitative PCR analysis of immunoprecipitated DNA showed that the P1 (-310/-290 bp) and P2 (-327/-313 bp) fragments were the most enriched (Fig. 6 C), suggesting that the P1 and P2 fragments may contribute to the vitexin-regulated VDR regulation of PBLD transcription. Based on the ChIP results, a series of luciferase reporter genes driven by different PBLD gene fragments were constructed. Based on bioinformatics analysis, we deleted two VDRE sites in specific intervals of the PBLD promoter and examined the effect of VDR on the transcriptional activity of the PBLD promoter (Fig. 6 D). Mutation 2 significantly attenuated the enhancing effect of VDR, whereas mutation 1 had no effect (Fig. 6 E). These results suggest that VDR can enhance PBLD transcription by directly binding to the P1 fragment of the PBLD promoter. The VDR was significantly increased following stimulation with vitexin, indicating that vitexin may enhance the activation of the PBLD promoter by VDR. Previous studies have demonstrated that epithelial PBLD plays a role in mitigating intestinal inflammatory responses and enhancing intestinal barrier function, potentially through the inhibition of the NF-κB signaling pathway [ 35 ]. However, whether it affects macrophage polarization has not been reported. Weknocked down the PBLD protein in THP-1 cells (Fig. 6 F) and analyzed the effects by flow cytometry and PCR. The results showed that the ability to regulate macrophages disappeared in the siPBLD group compared with the control group after vitexin treatment (Fig. 6 G-H). Meanwhile, there was no significant change in the mRNA levels of iNOS2 and ARG-1 by vitexin in THP-1 cells with VDR knocked down (Fig. 6 I). Overall, the regulation of macrophage polarization by vitexin in the tumor microenvironment is dependent on the VDR-PBLD pathway.
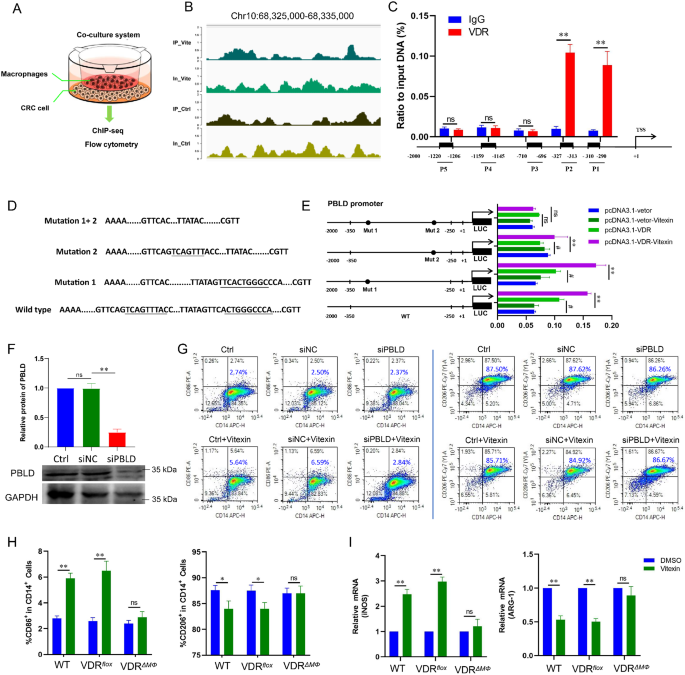
Vitexin regulates macrophage polarization in the tumor microenvironment via the VDR/PBLD pathway. A ) Schematic diagram of the in vitro Transwell-based coculture system. B ) Binding maps of VDR to the PBLD gene were analyzed from ChIP-Seq data in the control and vitexin groups and visualized by IGV software. C ) The abundance of gene fragments in the input and immunoprecipitates was evaluated using designated primers through real-time PCR, and the position of the primers was detected by ChIP. D ) Schematic representation of the PBLD promoter containing three VDREs, with the promoter’s mutation strategy shown in the figure. E ) Transcriptional regulatory activity of VDR on full-length PBLD promoter and doubly VDRE-mutated PBLD promoter in HEK293T cells with or without Vitexin treatment measured by dual-luciferase reporter gene assay. F ) Western blot assay for VDR expression. G ) Flow cytometry analysis of CD86 and CD206 levels. Data are presented as representative FACS plots. H ) Statistical results of macrophage typing. I ) mRNA levels of INOS and ARG-1. Data are expressed as SEM ± mean ( n = 3). # , P < 0.05; ## , P < 0.01; *, P < 0.05; **, P < 0.01; ns, no significance
In vivo, vitexin mitigates the transition from chronic intestinal inflammation to cancer by modulating macrophage polarization and is dependent on the VDR/PBLD pathway
The above results in vitro demonstrated that vitexin modulates the VDR/PBLD pathway to regulate macrophage polarization in the tumor microenvironment. We constructed mid-CAC mice and administered vitexin intervention. As shown in Fig. 7 A-B, all mice in the mid-stage CAC group had significantly decreased body weight compared with the normal group. In animals with normal macrophage VDR expression (VDR flox ), the body weights of animals in both calcitriol and vitexin intervention groups were significantly increased, however, in animals with macrophage VDR deletion (VDR ΔMΦ ), administration of the intervention groups did not have an effect, and the body weights of the animals were not affected compared to the CAC group. As shown in Fig. 7 C, in the VDR flox group, the body weight DAI of mice in the mid-term CAC group was significantly increased compared to the normal group, but the DAI was significantly decreased after intervention with both calcitriol and vitexin. However, in the VDR ΔMΦ group, the administered intervention group did not have an intervention effect, and the animals’ DAI was not changed compared with the CAC group. As shown in Fig. 7 D, compared with the normal group, the colons of mice in the model group had different degrees of shortening, swelling, and a little tumor-like growth. As shown in Fig. 7 E-F, in the VDR flox group, the length of the colon was significantly longer and the thickness was significantly decreased after the treatment with calcitriol and vitexin compared with the model group. The number of tumors was significantly reduced after calcitriol treatment, but there was no significant difference for vitexin. In addition, as shown in the colon pathogram (Fig. 7 G), the inhibitory effect of vitexin on the development of colorectal cancer was not significant in the VDR ΔMΦ group. This suggests that vitexin inhibits colorectal cancer development through VDR. In the VDR ΔMΦ group, there was a loss of tissue morphology in the marginal zone of colorectal cancer, with disturbed cell arrangement, necrosis, and hyperplasia. In the VDR flox group, the intervention of vitexin was able to reduce the hyperplasia of the cancerous zone and improve the tissue morphology, whereas the improvement of the cancerous zone by vitexin was not significant in the VDR ΔMΦ group, which indicated that the improvement of cancerous cell hyperplasia by vitexin in the cancerous zone relied on the VDR proteins. We employed multi-immunohistochemistry techniques, as illustrated in Fig. 7 H, to analyze colonic tissues from normal mice. The results indicated that the normal group exhibited abundant expression of VDR proteins, while the phenotypic expression of macrophage cells was notably low. In contrast, macrophages in the model group displayed significant polarization and markedly reduced levels of VDR compared to the normal group. However, following vitexin administration, there was a significant increase in both VDR and CD163 levels, suggesting that vitexin upregulates VDR protein and modulates macrophage polarization towards the M1 phenotype. Consistent with these findings, western blot analysis revealed that the expression of PBLD and PCNA proteins was significantly decreased in the CAC group compared to the normal group. Notably, after vitexin treatment, the expression levels of PBLD and PCNA proteins in the colon tissues of unknocked-out VDR mice approached those observed in normal mice (Fig. 7 I-J). However, for the tissues of mice with knockout VDR, the above proteins did not change significantly after vitexin treatment (Fig. 7 I-J). In conclusion, vitexin mitigates the transition from chronic intestinal inflammation to cancer by modulating macrophage polarization and is dependent on the VDR/PBLD pathway.
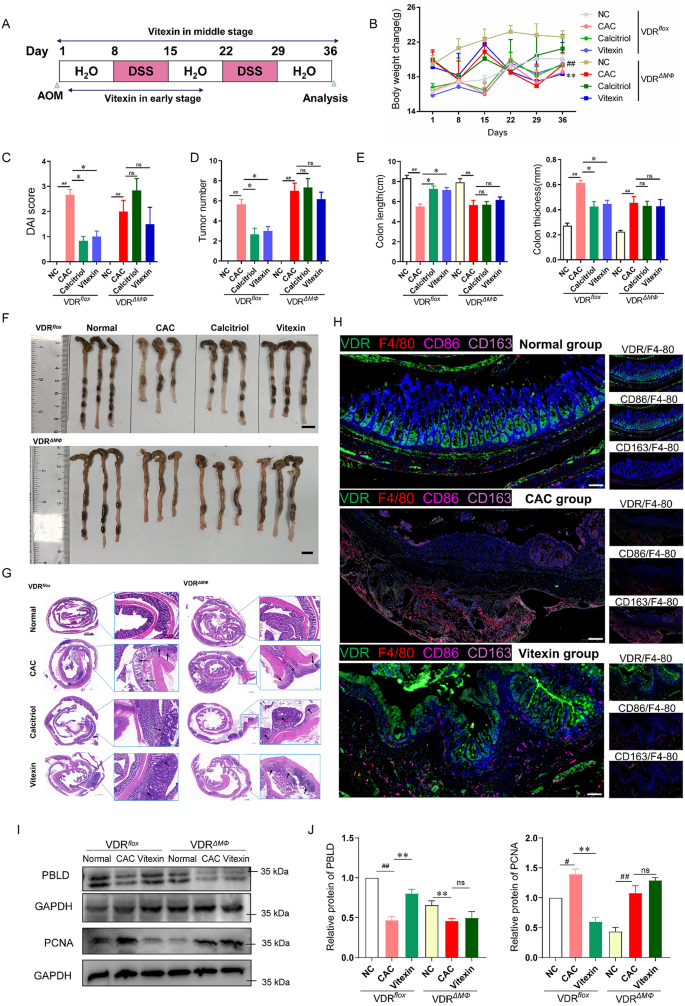
Vitexin mitigates the transition from chronic intestinal inflammation to cancer by modulating macrophage polarization and is dependent on the VDR/PBLD pathway. A ) Flow chart of drug administration. B ) Graph of body weight changes (The data are presented as the means ± SEM ( n = 6). In the VDR flox group, ## P < 0.01 compared with the NC group and ** P < 0.01 compared with the CAC group.). C ) DAI score. D ) Tumor number. E ) Colon length (left), colon thickness (right). F ) Macroscopic view of colon, Scale bar, 1 cm. G ) H&E staining of the colon, Scale bar: 1000 μm and 200 μm, n = 3). H ) Multicolour immunohistochemistry of colon tissue (VDR-green, F4/80 red, CD86 pink, CD163 rose. Scale bar, 500 μm, n = 3). I-J ) The expression of PBLD and PCNA proteins and statistical plots ( n = 3). The data are presented as the means ± SEM ( n = 6). # P < 0.05, ## P < 0.01;* P < 0.05, ** P < 0.01; ns, no significance
Chronic colitis is recognized as a significant risk factor for the development of colitis-associated cancer, which typically progresses through a sequence of inflammation, typical hyperplasia, and subsequent cancerization. This progression involves a transition of the lesion microenvironment from an inflammatory state to a tumor-promoting microenvironment. In this study, we specifically targeted the VDR to modulate macrophage polarization, demonstrating that vitexin can inhibit the transition from chronic colitis to CAC. Our findings indicate that vitexin binds to the VDR protein, thereby preventing the differentiation of macrophages into the M2 phenotype and preserving the cytotoxic effects of inflammatory cytokines produced by M1-type macrophages. In vitro co-culture experiments revealed that vitexin increased the proportion of M1-type macrophages, supporting its anti-cancer properties. Furthermore, we employed myeloid-specific VDR gene knockout mice to elucidate the critical role of VDR in the mid-term progression of CAC and to confirm the targeting of VDR by vitexin. These key findings are illustrated in the graphical abstract.
One of the most important contributions of this study may be the discovery of a natural product - vitexin, which is a novel VDR agonist. Vitexin, as a flavonoid active substance, has been reported to have therapeutic effects on many cancers, including breast cancer, cervical cancer, and many other cancers [ 36 , 37 , 38 ]. Our previous studies have also confirmed that vitexin can alleviate the disease in UC and CAC mice, suggesting that vitexin may have a broad effect on cancers associated with the evolution of colitis [ 21 , 39 ]. However, the molecular mechanisms and targets of vitexin remain unknown, limiting the application of vitexin.
VDR, a nuclear receptor transcription factor, has been investigated in clinical and basic studies for its important role in colitis and colorectal cancer [ 40 , 41 , 42 , 43 ]. Studies have correlated vitamin D with survival and mortality in colorectal cancer, and in a large group of CRC patients, higher VDR expression in tumor stromal fibroblasts was associated with longer survival [ 44 ]. In the present study, it was also confirmed that mid-stage CAC mice with VDR on the table of CAC mice deficient in VDR showed more severe colon lesions and accelerated their cancerous process compared to control mice. These results suggest that VDR is a potential therapeutic target. However, one of the most promising vitamin D analogs, seocalcitol, has not demonstrated efficacy in phase II clinical trials [ 45 ]. Recent findings suggest that the relationship between elevated vitamin D levels and a reduced risk of colorectal cancer is not universally supported. Additionally, systemic activation of vitamin D signaling poses a potential risk of hypercalcemia, which can lead to severe toxicity. Notably, this study identifies vitexin as a novel agonist of the vitamin D receptor (VDR), demonstrating its ability to bind directly to the ligand-binding domain of VDR. The interaction between vitexin and VDR is significantly influenced by the residue THR-287 within the VDR structural domain, which may facilitate the design and development of new small-molecule VDR agonists. Furthermore, our findings indicate that vitexin markedly upregulates the transcription of VDR’s classical target gene, Cyp24a1. However, the implications of vitexin on the overall targeting of VDR during the CAC process, following VDR’s translocation to the nucleus, warrant further investigation.
Previous studies have shown that vitexin can alleviate the condition of CAC mice by regulating the polarization of macrophages, but its mechanism has not been thoroughly studied. As a novel VDR agonist, it is unknown whether vitexin is related to macrophage polarization. Therefore, we used an in vitro co-culture method to simulate the regulatory effect of vitexin on macrophages in the tumor microenvironment. The results found that vitexin can upregulate the content of VDR in the nucleus of macrophages, promote its entry into the cell nucleus, upregulate the polarization of M1-type macrophages, and downregulate the polarization of M2-type macrophages. However, when we extracted VDR knockout cells for further verification, we found that in macrophages with VDR knockout, vitexin could not exert its regulatory effect on macrophage polarization, indicating that the regulation of vitexin on macrophage polarization depends on VDR protein. Recent studies have confirmed that VDR-deficient keratinocyte-derived exosome miR-4505 promotes macrophage polarization towards the M1 phenotype, and that VDR can mediate hepatic ischemia-reperfusion injury by regulating M2 macrophage polarization through autophagy [ 46 , 47 ]. However, how vitexin affects VDR and thus regulates macrophage polarization is not yet known.
Genomics and proteomics data have shown that PBLD expression is deficient in a variety of tumors. PBLD is expressed in the liver, stomach, mammary gland, kidney, and intestine, and acts as a tumor suppressor in gastric carcinoma [ 48 ], hepatocellular carcinoma [ 49 , 50 ], and breast cancer [ 51 ]. acting as a tumor suppressor. The inhibitory role of PBLD in cancer is relatively clear; however, little is known about the role of PBLD in macrophage polarization, especially in CAC. A previous study demonstrated that PBLD levels were significantly reduced in UC and that PBLD expression levels were negatively correlated with UC severity, inhibiting NF-κB and epithelial-mesenchymal transition signaling pathways and acting as a tumor suppressor [ 35 ]. We report, for the first time, that the VDR directly binds to the − 310/-290 promoter region of the PBLD gene, thereby promoting its transcription and expression. Following treatment with vitexin, we observed a significant increase in the transcriptional expression of PBLD, indicating that vitexin enhances PBLD expression. Subsequently, we knocked down PBLD expression in THP-1 cells within the tumor microenvironment and found that the regulatory effect of vitexin on macrophage polarization was abolished. These findings demonstrate that vitexin can modulate macrophage polarization in the tumor microenvironment, with this effect being dependent on the VDR/PBLD signaling pathway.
In this study, we generated bone marrow-specific VDR gene knockout mice, which demonstrated an accelerated progression of colorectal cancer. These findings provide compelling evidence that VDR may serve as a potential target for preventing the transition from colitis to colorectal cancer. Notably, we observed that the absence of VDR in colitis-associated colorectal cancer (CAC) mice during mid-term treatment with vitexin did not yield therapeutic effects, nor did we detect any modulation of macrophage polarization by vitexin. A limitation of this study may be the lack of evaluation of VDR expression in specific intestinal macrophage populations. Although VDR expression in the intestine is relatively low, its expression in intestinal macrophages is significant, underscoring VDR’s critical role in intestinal immunity.
In conclusion, our findings indicate a significant reduction in the expression of VDR protein in mid-term CAC mice, with the absence of VDR protein appearing to accelerate the progression of CAC. This acceleration is likely attributable to alterations in macrophage polarization. Vitexin has been shown to directly target VDR, binding to the VDR-LBD structural domain, which facilitates its translocation into the cell nucleus. This process subsequently regulates the transcription of PBLD and influences macrophage polarization. These results underscore the direct targeting capability of vitexin and highlight the critical role of VDR as a key pathogenic factor in the transition from colitis to colorectal cancer. Furthermore, our study supports the potential for future research and the application of vitexin in preventing the progression from colitis to colorectal cancer.
Data availability
No datasets were generated or analysed during the current study.
Abbreviations
Azoxymethane
Bone marrow-derived macrophage
Colitis associated cancer
Cellular heat transfer assay
Colorectal cancer
Drug affinity responsive target stability
Dextran sulfate sodium salt
Isothermal Titration Calorimetry
Phenazine biosynthesis like protein domain
Surface plasmon resonance assay
Tumor microenvironment
Vitamin D receptors
Zhang L, Li Z, Skrzypczynska KM, Fang Q, Zhang W, O’Brien SA, He Y, Wang L, Zhang Q, Kim A, et al. Single-cell analyses inform mechanisms of myeloid-targeted therapies in Colon cancer. Cell. 2020;181:442–+.
Article CAS PubMed Google Scholar
Fujita M, Matsubara N, Matsuda I, Maejima K, Oosawa A, Yamano T, Fujimoto A, Furuta M, Nakano K, Oku-Sasaki A, et al. Genomic landscape of colitis-associated cancer indicates the impact of chronic inflammation and its stratification by mutations in the wnt signaling. Oncotarget. 2018;9:969–81.
Article PubMed Google Scholar
Wang X, Chen JDZ. Therapeutic potential and mechanisms of sacral nerve stimulation for gastrointestinal diseases. J Translational Intern Med. 2023;11:115–27.
Article CAS Google Scholar
Xu Y, Liu K, Li C, Li M, Liu F, Zhou X, Sun M, Ranganathan M, Zhang L, Wang S et al. The largest Chinese cohort study indicates homologous recombination pathway gene mutations as another major genetic risk factor for colorectal Cancer with heterogeneous clinical phenotypes. Research 2023, 6.
Yaeger R, Shah MA, Miller VA, Kelsen JR, Wang K, Heins ZJ, Ross JS, He Y, Sanford E, Yantiss RK, et al. Genomic alterations observed in Colitis-Associated Cancers are distinct from those found in sporadic colorectal cancers and vary by type of inflammatory bowel disease. Gastroenterology. 2016;151:278–.
Ma X, Meng Z, Jin L, Xiao Z, Wang X, Tsark WM, Ding L, Gu Y, Zhang J, Kim B, et al. CAMK2γ in intestinal epithelial cells modulates colitis-associated colorectal carcinogenesis via enhancing STAT3 activation. Oncogene. 2017;36:4060–71.
Article CAS PubMed PubMed Central Google Scholar
Xu R, Du A, Deng X, Du W, Zhang K, Li J, Lu Y, Wei X, Yang Q, Tang H. tsRNA-GlyGCC promotes colorectal cancer progression and 5-FU resistance by regulating SPIB. J Exp Clin Cancer Res. 2024;43:230.
Saeed H, Leibowitz BJ, Zhang L, Yu J. Targeting myc-driven stress addiction in colorectal cancer. Drug Resist Updates 2023, 69.
Xing P, Wang S, Cao Y, Liu B, Zheng F, Guo W, Huang J, Zhao Z, Yang Z, Lin X et al. Treatment strategies and drug resistance mechanisms in adenocarcinoma of different. Drug Resist Updates 2023, 71.
Wu S, Yan M, Liang M, Yang W, Chen J, Zhou J. Supramolecular host-guest nanosystems for overcoming cancer drug resistance. Cancer drug Resist (Alhambra Calif). 2023;6:805–27.
Rainho MA, Siqueira PB, de Amorim ISS, Mencalha AL, Thole AA. Mitochondria in colorectal cancer stem cells - a target in drug resistance. Cancer drug Resist (Alhambra Calif). 2023;6:273–83.
Jiang Z, Zou Q, Chen Q, Zhang J, Tang H, Chen J, Qin Y, Yang L, Chen Z, Cao L. Therapeutic role of Wuda granule in gastrointestinal motility disorder through promoting gastrointestinal motility and decreasing inflammatory level. Front Pharmacol 2023, 14.
Wu B, Shi X, Jiang M, Liu H. Cross-talk between cancer stem cells and immune cells: potential therapeutic targets in the tumor immune microenvironment. Mol Cancer 2023, 22.
Yuan Z, Li Y, Zhang S, Wang X, Dou H, Yu X, Zhang Z, Yang S, Xiao M. Extracellular matrix remodeling in tumor progression and immune escape: from mechanisms to treatments. Mol Cancer 2023, 22.
Xiang D, Jiang L, Yuan Q, Yu Y, Liu R, Chen M, Kuai Z, Zhang W, Yang F, Wu T et al. Leukocyte-specific morrbid promotes leukocyte differentiation and atherogenesis. Research 2023, 6.
Yang W, Ma Y, Xu H, Zhu Z, Wu J, Xu C, Sun W, Zhao E, Wang M, Reis RL et al. Mulberry uiomass-derived nanomedicines mitigate colitis through improved inflamed mucosa accumulation and intestinal microenvironment modulation. Research 2023, 6.
Zhang H, Wang X, Zhang J, He Y, Yang X, Nie Y, Sun L. Crosstalk between gut microbiota and gut resident macrophages in inflammatory bowel disease. J Translational Intern Med. 2023;11:382–92.
Article Google Scholar
Lu J, Li J, Lin Z, Li H, Lou L, Ding W, Ouyang S, Wu Y, Wen Y, Chen X et al. Reprogramming of TAMs via the STAT3/CD47-SIRPα axis promotes acquired resistance to EGFR-TKIs in lung cancer. Cancer Lett 2023, 564.
Wang Z, Wang S, Jia Z, Hu Y, Cao D, Yang M, Liu L, Gao L, Qiu S, Yan W et al. YKL-40 derived from infiltrating macrophages cooperates with GDF15 to establish an immune suppressive microenvironment in gallbladder cancer. Cancer Lett 2023, 563.
Shan H, Dou W, Zhang Y, Qi M. Targeted ferritin nanoparticle encapsulating CpG oligodeoxynucleotides induces tumor-associated macrophage M2 phenotype polarization into M1 phenotype and inhibits tumor growth. Nanoscale. 2020;12:22268–80.
Chen Y, Wang B, Yuan X, Lu Y, Hu J, Gao J, Lin J, Liang J, Hou S, Chen S. Vitexin prevents colitis-associated carcinogenesis in mice through regulating macrophage polarization. Phytomedicine 2021, 83.
Abreu MT, Kantorovich V, Vasiliauskas EA, Gruntmanis U, Matuk R, Daigle K, Chen S, Zehnder D, Lin YC, Yang H, et al. Measurement of vitamin D levels in inflammatory bowel disease patients reveals a subset of Crohn’s disease patients with elevated 1,25-dihydroxyvitamin D and low bone mineral density. Gut. 2004;53:1129–36.
Song M, Chan AT, Sun J. Influence of the gut microbiome, diet, and environment on risk of colorectal cancer. Gastroenterology. 2020;158:322–40.
Knackstedt R. The Importance of vitamin D, the vitamin D receptor (VDR) and retinoid X receptor alpha (RXRα) in murine colitis development and progression. Dissertation/Thesis. 2013.
Levi-Galibov O, Lavon H, Wassermann-Dozorets R, Pevsner-Fischer M, Mayer S, Wershof E, Stein Y, Brown LE, Zhang W, Friedman G et al. Heat shock factor 1-dependent extracellular matrix remodeling mediates the transition from chronic intestinal inflammation to colon cancer. Nat Commun 2020, 11.
Kohler LHF, Reich S, Yusenko M, Klempnauer K-H, Begemann G, Schobert R, Biersack B. Multimodal 4-arylchromene derivatives with microtubule-destabilizing, anti-angiogenic, and MYB-inhibitory activities. Cancer drug Resist (Alhambra Calif). 2023;6:59–77.
Chen Y, Zhu S, Chen Z, Liu Y, Pei C, Huang H, Hou S, Ning W, Liang J. Gingerenone A alleviates ferroptosis in secondary liver injury in colitis mice via activating Nrf2-Gpx4 signaling pathway. J Agric Food Chem. 2022;70:12525–34.
Liang J, Dai W, Liu C, Wen Y, Chen C, Xu Y, Huang S, Hou S, Li C, Chen Y et al. Gingerenone A attenuates Ulcerative Colitis via Targeting IL-17RA to inhibit inflammation and restore intestinal barrier function. Adv Sci 2024.
Chen Y-e, Xu S-j, Lu Y-y, Chen S-x, Du X-h, Hou S-z, Huang H-y, Liang J. Asperuloside suppressing oxidative stress and inflammation in DSS-induced chronic colitis and RAW 264.7 macrophages via Nrf2/HO-1 and NF-κB pathways. Chemico-Biol Interact 2021, 344.
Chen Y, Yuan X, Pei C, Deng Z, Du X, Liang J, He L, Hou S. Vitexin alleviates breast tumor in mice via skewing TAMs toward an iNOS < SUP>+ profile orchestrating effective CD8 < SUP>+ T cell activation. J Funct Foods 2022, 95.
Dong Y, Zhu G, Wang S-F, Keon KA, Rubinstein JL, Zeng S-X, Zhang S, Chen Q-L, Fu J, Li M, et al. Toosendanin, a novel potent vacuolar-type H plus -translocating ATPase inhibitor, sensitizes cancer cells to chemotherapy by blocking protective autophagy. Int J Biol Sci. 2022;18:2684–702.
Yang H, Liu Y, Zhao M-M, Guo Q, Zheng X-K, Liu D, Zeng K-W, Tu P-F. Therapeutic potential of targeting membrane-spanning proteoglycan SDC4 in hepatocellular carcinoma. Cell Death Dis 2021, 12.
Luo W, Lin K, Hua JY, Han JB, Zhang QY, Chen LF, Khan ZA, Wu GJ, Wang Y, Liang G. Schisandrin B attenuates diabetic Cardiomyopathy by targeting MyD88 and inhibiting MyD88-dependent inflammation. Adv Sci 2022, 9.
Takada I, Makishima M. Control of inflammatory bowel disease and colorectal cancer by synthetic vitamin D receptor ligands. Curr Med Chem. 2017;24:868–75.
Chen S, Liu H, Li Z, Tang J, Huang B, Zhi F, Zhao X. Epithelial PBLD attenuates intestinal inflammatory response and improves intestinal barrier function by inhibiting NF-κB signaling. Cell Death Dis 2021, 12.
Liang C, Jiang Y, Sun L. Vitexin suppresses the proliferation, angiogenesis and stemness of endometrial cancer through the PI3K/AKT pathway. Pharm Biol. 2023;61:581–9.
Najafipour R, Momeni AM, Mirmazloomi Y, Moghbelinejad S. Vitexin induces apoptosis in MCF-7 breast Cancer cells through the regulation of specific miRNAs expression. Int J Mol Cell Med. 2022;11:197–206.
CAS PubMed PubMed Central Google Scholar
Wang Q, Zhang J, Ye J, Guo J. Vitexin exerts anti-tumor and anti-angiogensis effects on cervical cancer through VEGFA/VEGFR2 pathway. Eur J Gynaecol Oncol. 2022;43:86–91.
Google Scholar
Zhang J, Liang F, Chen Z, Chen Y, Yuan J, Xiong Q, Hou S, Huang S, Liu C, Liang J. Vitexin protects against dextran sodium sulfate-induced colitis in mice and its potential mechanisms. J Agric Food Chem 2022.
Vernia F, Valvano M, Longo S, Cesaro N, Viscido A, Latella G. Vitamin D in inflammatory bowel diseases. mechanisms of action and therapeutic implications. Nutrients 2022, 14.
Chen J, Ruan X, Yuan S, Deng M, Zhang H, Sun J, Yu L, Satsangi J, Larsson SC, Therdoratou E, et al. Antioxidants, minerals and vitamins in relation to Crohn’s disease and ulcerative colitis: a mendelian randomization study. Aliment Pharmacol Ther. 2023;57:399–408.
Garcia-Martinez JM, Chocarro-Calvo A, Martinez-Useros J, Regueira-Acebedo N, Fernandez-Acenero MJ, Munoz A, Larriba MJ, Garcia-Jimenez C. SIRT1 mediates the antagonism of Wnt/β-catenin pathway by vitamin D in colon carcinoma cells. bioRxiv 2024.
Zhang Y, Xie J. Targeting ferroptosis regulators by natural products in colorectal cancer. Front Pharmacol 2024, 15.
Xia X, Xu F, Dai D, Xiong A, Sun R, Ling Y, Qiu L, Wang R, Ding Y, Lin M et al. VDR is a potential prognostic biomarker and positively correlated with immune infiltration: a comprehensive pan-cancer analysis with experimental verification. Biosci Rep 2024, 44.
Tocchini-Valentini G, Rochel N, Wurtz JM, Moras D. Crystal structures of the vitamin D nuclear receptor liganded with the vitamin D side chain analogues calcipotriol and seocalcitol, receptor agonists of clinical importance. Insights into a structural basis for the switching of calcipotriol to a receptor antagonist by further side chain modification. J Med Chem. 2004;47:1956–61.
Sun W, Chen J, Li J, She X, Ma H, Wang S, Liu J, Yuan Y. Vitamin D receptor-deficient keratinocytes-derived exosomal miR-4505 promotes the macrophage polarization towards the M1 phenotype. Peerj 2023, 11.
Zhang Y, Zhou J, Hua L, Li P, Wu J, Shang S, Deng F, Luo J, Liao M, Wang N et al. Vitamin D receptor (VDR) on the cell membrane of mouse macrophages participates in the formation of lipopolysaccharide tolerance: mVDR is related to the effect of artesunate to reverse LPS tolerance. Cell Communication Signal 2023, 21.
Li D-M, Zhang J, Li W-M, Cui J-T, Pan Y-M, Liu S-Q, Xing R, Lu Y-Y. MAWBP and MAWD inhibit proliferation and invasion in gastric cancer. World J Gastroenterol. 2013;19:2781–92.
Li A, Yan Q, Zhao X, Zhong J, Yang H, Feng Z, Du Y, Wang Y, Wang Z, Wang H, et al. Decreased expression of PBLD correlates with poor prognosis and functions as a tumor suppressor in human hepatocellular carcinoma. Oncotarget. 2016;7:524–37.
Wu J, Niu Q, Yuan J, Xu X, Cao L. Novel compound cedrelone inhibits hepatocellular carcinoma progression via PBLD and Ras/Rap1. Experimental Therapeutic Med. 2019;18:4209–20.
CAS Google Scholar
Liang Y, Song X, Li Y, Su P, Han D, Ma T, Guo R, Chen B, Zhao W, Sang Y et al. vol 38, pg 6850, : circKDM4C suppresses tumor progression and attenuates doxorubicin resistance by regulating miR-548p/PBLD axis in breast cancer (2019). Oncogene 2021, 40:2816–2816.
Download references
This work was supported by the Open Subjects of State Key Laboratory of Dampness Syndrome of Chinese Medicine jointly established by the Ministry of Provincial Affairs of the People’s Republic of China; National Natural Science Foundation of China (82304905, 82272326); Natural Science Foundation of Guangdong Province (2023A1515011729, 2022A1515012408); Foundation and Applied Basic Research Fund Project of Guangdong Province (2021B1515140045 and 2021B1515140050), Guangzhou Science and Technology Bureau (202102080643), Science and Technology Program of Guangzhou (202002020032), The core technology project in the field of biological industry (2021ZD006), Regional Union Fund-Regional Nurturing Project (2021B1515140065), and Science and technology project in key fields of Nansha District (2022ZD004), the Open Project of State Key Laboratory of Respiratory Disease (SKLRD-OP-202202 and SKLRD-OP-202317); the Guangzhou Medical University Discipline Construction Funds (Basic Medicine) (JCXKJS2022A11).
Author information
Yonger Chen, Jian Liang and Shuxian Chen contributed equally to this work.
Authors and Affiliations
School of Basic Medical Sciences, State Key Laboratory of Respiratory Disease, Sino-French Hoffmann Institute, Guangzhou Medical University; The Affiliated Panyu Central Hospital of Guangzhou Medical University; Guangdong Provincial Key Laboratory of Allergy & Clinical Immunology, The Second Affiliated Hospital of Guangzhou Medical University, Guangzhou, Guangdong, 511436, China
Yonger Chen & Mingsheng Cai
State Key Laboratory of Dampness Syndrome of Chinese Medicine, The Second Affiliated Hospital of Guangzhou University of Chinese Medicine; School of Pharmaceutical Sciences, Guangzhou University of Chinese Medicine, Guangzhou, Guangdong, 510006, China
Yonger Chen, Jian Liang, Shuoxi Xu, Jindian Miao, Jing Zhang, Chen Chen & Shaozhen Hou
Department of Hepatobiliary Surgery, The Third Affiliated Hospital of Sun Yat-Sen University, Guangzhou, 510000, China
Shuxian Chen & Nan Lin
Department of Pharmacy, The Second Affiliated Hospital of Guangzhou, University of Chinese Medicine, Guangzhou, 510120, China
State Key Laboratory of Oncology in South China Guangdong Provincial Clinical Research, Center for Cancer Sun Yat-Sen University Cancer Center Guangzhou, Guangzhou, 510060, China
Zhuoya Xie, Xiaoli Wei & Hailin Tang
Clinical Medical College of Acupuncture Moxibustion and Rehabilitation, Guangzhou University of Chinese Medicine, Guangzhou, Guangdong, 510006, China
You can also search for this author in PubMed Google Scholar
Contributions
Y. C., J. L and S. C. designed, performed, and analyzed experiments, and wrote the manuscript. N. L., and X.S. designed and analyzed experiments. J.Z. and C. C. designed image analysis and provided intellectual input. Y.C. and X.Y. designed and performed statistical and image analysis. Z. X., M. C. and E. Z. assisted with image acquisition and designed image analysis. M. C., S. W., S. H. and H. T. directed the study, designed and analyzed experiments, and wrote the manuscript.
Corresponding authors
Correspondence to Mingsheng Cai , Xiaoli Wei , Shaozhen Hou or Hailin Tang .
Ethics declarations
Ethics approval and consent to participate.
All mouse experiments were approved by the Animal Ethics Committee of the NKI and were performed according to institutional and national guidelines.
Competing interests
The authors declare no competing interests.
Additional information
Publisher’s note.
Springer Nature remains neutral with regard to jurisdictional claims in published maps and institutional affiliations.
Electronic supplementary material
Below is the link to the electronic supplementary material.
Supplementary Material 1
Supplementary material 2, rights and permissions.
Open Access This article is licensed under a Creative Commons Attribution-NonCommercial-NoDerivatives 4.0 International License, which permits any non-commercial use, sharing, distribution and reproduction in any medium or format, as long as you give appropriate credit to the original author(s) and the source, provide a link to the Creative Commons licence, and indicate if you modified the licensed material. You do not have permission under this licence to share adapted material derived from this article or parts of it. The images or other third party material in this article are included in the article’s Creative Commons licence, unless indicated otherwise in a credit line to the material. If material is not included in the article’s Creative Commons licence and your intended use is not permitted by statutory regulation or exceeds the permitted use, you will need to obtain permission directly from the copyright holder. To view a copy of this licence, visit http://creativecommons.org/licenses/by-nc-nd/4.0/ .
Reprints and permissions
About this article
Cite this article.
Chen, Y., Liang, J., Chen, S. et al. Discovery of vitexin as a novel VDR agonist that mitigates the transition from chronic intestinal inflammation to colorectal cancer. Mol Cancer 23 , 196 (2024). https://doi.org/10.1186/s12943-024-02108-6
Download citation
Received : 01 July 2024
Accepted : 02 September 2024
Published : 13 September 2024
DOI : https://doi.org/10.1186/s12943-024-02108-6
Share this article
Anyone you share the following link with will be able to read this content:
Sorry, a shareable link is not currently available for this article.
Provided by the Springer Nature SharedIt content-sharing initiative
- Vitamin D receptor
- Colitis-associated colorectal cancer
Molecular Cancer
ISSN: 1476-4598
- General enquiries: [email protected]
- Alzheimer's disease & dementia
- Arthritis & Rheumatism
- Attention deficit disorders
- Autism spectrum disorders
- Biomedical technology
- Diseases, Conditions, Syndromes
- Endocrinology & Metabolism
- Gastroenterology
- Gerontology & Geriatrics
- Health informatics
- Inflammatory disorders
- Medical economics
- Medical research
- Medications
- Neuroscience
- Obstetrics & gynaecology
- Oncology & Cancer
- Ophthalmology
- Overweight & Obesity
- Parkinson's & Movement disorders
- Psychology & Psychiatry
- Radiology & Imaging
- Sleep disorders
- Sports medicine & Kinesiology
- Vaccination
- Breast cancer
- Cardiovascular disease
- Chronic obstructive pulmonary disease
- Colon cancer
- Coronary artery disease
- Heart attack
- Heart disease
- High blood pressure
- Kidney disease
- Lung cancer
- Multiple sclerosis
- Myocardial infarction
- Ovarian cancer
- Post traumatic stress disorder
- Rheumatoid arthritis
- Schizophrenia
- Skin cancer
- Type 2 diabetes
- Full List »
share this!
September 16, 2024
This article has been reviewed according to Science X's editorial process and policies . Editors have highlighted the following attributes while ensuring the content's credibility:
fact-checked
trusted source
High-dose vitamin D3 does not provide benefit for metastatic colorectal cancer, clinical trial shows
by Dana-Farber Cancer Institute

SOLARIS (Alliance A021703), a multicenter, double-blind, randomized Phase III clinical trial led by Dana-Farber Cancer Institute researchers and conducted across several hundred cancer centers in the U.S. tested the addition of high-dose vitamin D3 to standard treatment for patients with untreated metastatic colorectal cancer. More than 450 patients received standard chemotherapy plus bevacizumab and were randomized to high-dose or standard dose vitamin D3.
The results were presented at the European Society for Medical Oncology 2024 congress.
The team observed no additional concerning side-effects or toxicities with the addition of high-dose vitamin D3. However, the addition of high-dose vitamin D3 to standard treatment did not delay the progression of cancer more so than standard-dose vitamin D3, according to the team's analysis after a median 20-month follow up.
A potential benefit for high-dose vitamin D3 was observed for patients with left-sided disease (i.e., primary tumors that arise in the descending colon, sigmoid colon, or rectum) and requires further investigation.
The SOLARIS trial was inspired by previous research suggesting that higher levels of vitamin D in the blood are associated with improved survival for metastatic colorectal cancer and that the addition of high-dose vitamin D3 to standard therapy could potentially improve progression free survival.
The SOLARIS results suggest, however, that high-dose vitamin D3 cannot be recommended as a treatment for patients with untreated metastatic colon cancer.
Explore further
Feedback to editors

10-year study shows tomosynthesis improves breast cancer detection
13 minutes ago

Research suggests many people already have T cells with the power to fight avian flu
17 minutes ago

New tumor models provide insights into deadly sarcomas
21 minutes ago

Intimate partner violence: Preserving patient privacy can save lives
33 minutes ago

Language sentiment can predict future changes in depression symptoms, study finds

High BMI during childhood linked to increased risk of schizophrenia in adulthood
34 minutes ago

Lower neighborhood opportunity may increase risk for preterm birth

Moderate caffeine consumption associated with lower risk of developing multiple cardiometabolic diseases, study finds

Propolis from a Morocco-native plant found to improve cognitive function in male rats experiencing chronic mild stress

New treatment extends ovarian function in older mice
11 hours ago
Related Stories

High-dose vitamin D shows benefit in patients with advanced colorectal cancer
Apr 9, 2019

Vitamin D and colorectal cancer survival
Jun 11, 2019

Do you need more vitamin D in the winter?
Dec 6, 2023

Higher doses of vitamin D did not reduce falls in at risk older adults
Dec 8, 2020

Study suggests that maintaining normal vitamin D levels may benefit patients with advanced skin cancer
Apr 24, 2023
Guideline recommends healthy adults under the age of 75 take the recommended daily allowance of vitamin D
Jun 3, 2024
Recommended for you

A new way to reprogram immune cells and direct them toward anti-tumor immunity
21 hours ago

Immunotherapy after surgery helps people with high-risk bladder cancer live cancer-free longer, clinical trial finds
20 hours ago

Study reveals how mutant p53 protein converts other proteins into cancer drivers
22 hours ago

Reprogramming cancer cells to attack themselves
Sep 16, 2024

Experimental drug helps cancer patients regain weight, study shows

Global trial ends 20-year debate over gastro-esophageal cancer treatment
23 hours ago
Let us know if there is a problem with our content
Use this form if you have come across a typo, inaccuracy or would like to send an edit request for the content on this page. For general inquiries, please use our contact form . For general feedback, use the public comments section below (please adhere to guidelines ).
Please select the most appropriate category to facilitate processing of your request
Thank you for taking time to provide your feedback to the editors.
Your feedback is important to us. However, we do not guarantee individual replies due to the high volume of messages.
E-mail the story
Your email address is used only to let the recipient know who sent the email. Neither your address nor the recipient's address will be used for any other purpose. The information you enter will appear in your e-mail message and is not retained by Medical Xpress in any form.
Newsletter sign up
Get weekly and/or daily updates delivered to your inbox. You can unsubscribe at any time and we'll never share your details to third parties.
More information Privacy policy
Donate and enjoy an ad-free experience
We keep our content available to everyone. Consider supporting Science X's mission by getting a premium account.
E-mail newsletter
- dana-farber.org
- Breast Oncology
- Gastrointestinal Oncology
- Genitourinary Oncology
- Gynecologic Oncology
- Hematologic Oncology
- Pediatric Oncology
- Find a Physician
- Find a Clinical Trial
- Refer a Patient
- Physician Liaison information
- General Contact Information
- Innovation and Collaboration Opportunities
High-Dose Vitamin D3 Does Not Provide Benefit for Metastatic Colorectal Cancer
RESEARCH SUMMARY Study Title: SOLARIS (Alliance A021703): A multicenter double-blind phase III randomized clinical trial of vitamin D combined with standard chemotherapy plus bevacizumab in patients with previously untreated metastatic colorectal cancer.
Publication: European Society for Medical Oncology 2024 Abstract LBA26
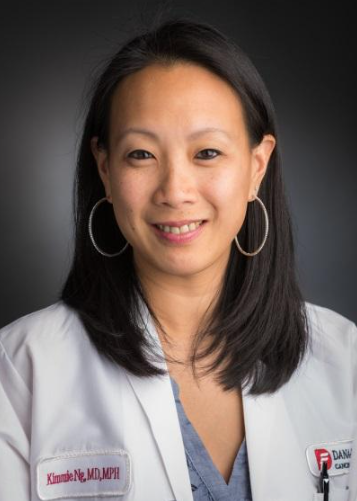
Kimmie Ng, MD, MPH/Dana-Farber Cancer Institute
Dana-Farber Cancer Institute authors : Kimmie Ng, MD, MPH , Nadine McCleary, MD, MPH , Jeffrey A. Meyerhardt, MD, MPH
Summary: A double-blind randomized phase 3 clinical trial led by Dana-Farber Cancer Institute researchers and conducted across several hundred cancer centers in the U.S. tested the addition of high-dose vitamin D3 to standard treatment for patients with untreated metastatic colorectal cancer. More than 450 patients received standard chemotherapy plus bevacizumab and were randomized to high-dose or standard dose vitamin D3. The team observed no additional concerning side-effects or toxicities with the addition of high-dose vitamin D3. However, the addition of high-dose vitamin D3 to standard treatment did not delay the progression of cancer more so than standard-dose vitamin D3, according to the team’s analysis after a median 20-month follow-up. A potential benefit for high-dose vitamin D3 was observed for patients with left-sided disease (i.e., primary tumors that arise in the descending colon, sigmoid colon, or rectum) and requires further investigation.
Significance: The SOLARIS trial was inspired by previous research suggesting that higher levels of vitamin D in the blood are associated with improved survival for metastatic colorectal cancer and that the addition of high-dose vitamin D3 to standard therapy could potentially improve progression free survival. The SOLARIS results suggest, however, that high-dose vitamin D3 cannot be recommended as a treatment for patients with untreated metastatic colon cancer.
Funding: National Cancer Institute; Pharmavite Contact: Victoria Warren, [email protected]
High-dose vitamin D3 cannot be recommended as treatment for metastatic colon cancer
- Download PDF Copy
Study title: SOLARIS (Alliance A021703): A multicenter double-blind phase III randomized clinical trial of vitamin D combined with standard chemotherapy plus bevacizumab in patients with previously untreated metastatic colorectal cancer.
Publication: European Society for Medical Oncology 2024 Abstract LBA26
Dana-Farber Cancer Institute authors: Kimmie Ng, MD, MPH, Nadine McCleary, MD, MPH, Jeffrey A. Meyerhardt, MD, MPH
Summary: A double-blind randomized phase 3 clinical trial led by Dana-Farber Cancer Institute researchers and conducted across several hundred cancer centers in the U.S. tested the addition of high-dose vitamin D3 to standard treatment for patients with untreated metastatic colorectal cancer. More than 450 patients received standard chemotherapy plus bevacizumab and were randomized to high-dose or standard dose vitamin D3. The team observed no additional concerning side-effects or toxicities with the addition of high-dose vitamin D3. However, the addition of high-dose vitamin D3 to standard treatment did not delay the progression of cancer more so than standard-dose vitamin D3, according to the team's analysis after a median 20-month follow up. A potential benefit for high-dose vitamin D3 was observed for patients with left-sided disease (i.e., primary tumors that arise in the descending colon, sigmoid colon, or rectum) and requires further investigation.
Related Stories
- Breakthrough technology detects cancer mutant genes with unprecedented sensitivity
- Cancer cases in the US continued to be underdiagnosed during the second year of the COVID-19 pandemic
- Researchers uncover why typically normal protein drives prostate cancer
Significance: The SOLARIS trial was inspired by previous research suggesting that higher levels of vitamin D in the blood are associated with improved survival for metastatic colorectal cancer and that the addition of high-dose vitamin D3 to standard therapy could potentially improve progression free survival. The SOLARIS results suggest, however, that high-dose vitamin D3 cannot be recommended as a treatment for patients with untreated metastatic colon cancer.
Funding: National Cancer Institute; Pharmavite
Dana-Farber Cancer Institute
Posted in: Drug Trial News | Medical Condition News
Tags: Bevacizumab , Blood , Cancer , Chemotherapy , Clinical Trial , Colon , Colon Cancer , Colorectal , Colorectal Cancer , Oncology , Q , Research , Vitamin D
Suggested Reading

Cancel reply to comment
- Trending Stories
- Latest Interviews
- Top Health Articles

How can microdialysis benefit drug development
Ilona Vuist
In this interview, discover how Charles River uses the power of microdialysis for drug development as well as CNS therapeutics.

Global and Local Efforts to Take Action Against Hepatitis
Lindsey Hiebert and James Amugsi
In this interview, we explore global and local efforts to combat viral hepatitis with Lindsey Hiebert, Deputy Director of the Coalition for Global Hepatitis Elimination (CGHE), and James Amugsi, a Mandela Washington Fellow and Physician Assistant at Sandema Hospital in Ghana. Together, they provide valuable insights into the challenges, successes, and the importance of partnerships in the fight against hepatitis.

Addressing Important Cardiac Biology Questions with Shotgun Top-Down Proteomics
In this interview conducted at Pittcon 2024, we spoke to Professor John Yates about capturing cardiomyocyte cell-to-cell heterogeneity via shotgun top-down proteomics.

Latest News

Online Help
Our 24/7 cancer helpline provides information and answers for people dealing with cancer. We can connect you with trained cancer information specialists who will answer questions about a cancer diagnosis and provide guidance and a compassionate ear.

Chat live online
Select the Live Chat button at the bottom of the page

Call us at 1-800-227-2345
Available any time of day or night
Our highly trained specialists are available 24/7 via phone and on weekdays can assist through online chat. We connect patients, caregivers, and family members with essential services and resources at every step of their cancer journey. Ask us how you can get involved and support the fight against cancer. Some of the topics we can assist with include:
- Referrals to patient-related programs or resources
- Donations, website, or event-related assistance
- Tobacco-related topics
- Volunteer opportunities
- Cancer Information
For medical questions, we encourage you to review our information with your doctor.
Colorectal Cancer
Whether you or a loved one are worried about getting colorectal cancer, have just been diagnosed, are going through colorectal cancer treatment, or are trying to stay well after treatment, this detailed information can help you find the answers you need.
About Colorectal Cancer
Colorectal cancer causes, risk factors, and prevention, early detection, diagnosis, and staging, treating colorectal cancer, after treatment, easy reading, if you have colon or rectal cancer.
If you have been diagnosed with colon or rectal cancer, this simple guide can help you understand the kind of treatment you may need and what questions to ask.
Downloadable PDFs
- About and Key Statistics [PDF]
- Causes, Risks, Prevention [PDF]
- Detection, Diagnosis, Staging [PDF]
- Treatment and Side Effects [PDF]
- Next Steps After Treatment [PDF]
Colon Pathology
Understanding your colon pathology report.
Information here can help you understand the medical language you might find in the pathology report from your colon or rectal biopsy.
This information is possible thanks to people like you.
We depend on donations to keep our cancer information available for the people who need it most.
Tools & Resources
Quiz: how much do you know about colorectal cancer.
Don’t be fooled by rumors and misinformation about your colon. Test your knowledge of 6 common beliefs about colorectal cancer.
Flyer: Colorectal Cancer: Catch It Early and Reduce Your Risk
Infographic: colorectal cancer screening guideline for people at average risk, colorectal cancer videos.
Watch videos about colon cancer risk factors, screening tests, and treatments. There are also personal stories from colon cancer survivors.
Personal Health Manager
Organize your cancer journey with the American Cancer Society Personal Health Manager.
Caregiver Resource Guide
A helpful tool for people who are caring for someone with cancer. View the complete guide here.
News & Stories
Colorectal cancer news, stories of hope, colorectal cancer research highlights, help us end cancer as we know it, for everyone..

If this was helpful, donate to help fund patient support services, research, and cancer content updates.

- Environment
- Election 2024
University of Kentucky research links ‘forever chemicals’ and colorectal cancer
By: sarah ladd - september 13, 2024 5:50 am.

New Hampshire collected PFAS-laden firefighting foam during a take-back program for fire departments in August. Disposal is through a technology called the PFAS Annihilator that breaks down the powerful chemical bonds. (New Hampshire Bulletin/Courtesy of Revive Environmental)
Over a lifetime, Kentuckians are repeatedly exposed to environmental pollutants known as “ forever chemicals ” — and a new study links such exposure to colorectal (colon) cancer .
Specifically, researchers at the University of Kentucky looked at long-term exposure to PFOS (perfluorooctane sulfonic acid) in mice and confirmed their findings in human cells. Results showed that exposure — commonly in food and water — can chip away at an enzyme in the intestines (HMGCS2) that can help protect against cancer.
Research has established that PFOS can increase risk of other types of cancer, including breast and liver, but this is the first to study the effects of the chemicals on this enzyme.
One of the researchers, Yekaterina Zaytseva, said it’s “not so easy” to mitigate these pollutants in the environment.
So, she turned her attention to its effects on the human body, and how to intercept them.
“We try to understand how these pollutants affect human health and … how we can mitigate the effect of these pollutants,” she said.

Scientists wanted to learn, she said, “if people live in (an) area with high exposure to these chemicals, how we can help … to prevent the harmful effects of these chemicals, or if they (are) already exposed, how we can mitigate … how they can get rid of these pollutants in their body.”
PFOS falls under the umbrella of per- and polyfluoroalkyl substances (PFAS), which are manmade and don’t break down in the environment easily because they have a molecular bond — carbon and fluorine — that’s among the strongest. Thus they have earned the nickname “forever chemicals.”
Their commonplace applications make exposure more complicated. The National Institute of Environmental Health Sciences explains that PFAS “ are used to keep food from sticking to packaging or cookware, make clothes and carpets resistant to stains and create firefighting foam that is more effective.”
PFOS are among the five “forever chemicals” included in a drinking-water regulation i ssued by the Biden administration in April. Utilities will be required to reduce PFAS in drinking water to the lowest level that can be reliably measured.
‘Forever chemicals:’ Now that feds have acted, some say Kentucky should do more
In Kentucky, state testing revealed 83 of 194 water treatment plants had at least one PFAS, as did 36 of 40 monitoring station testing surfaces, the Lantern has reported. In 2022, nearly 900 Kentuckians died with colorectal cancer, according to the Centers for Disease Control and Prevention.
Damage over time
People who have colorectal cancer or inflammatory bowel disease tend to not have the HMGCS2 enzyme, Zaytseva said, “suggesting that it’s actually … kind of protective against cancer.”
During exposure to PFOS, she said, “this enzyme is also lost.”
But this breakdown happens over time, and a person wouldn’t necessarily notice it happening based on side effects in the body.
“If you have, for example, chronic exposure to PFAS, you don’t see … this enzyme shut down right away,” said Zaytseva. “You wouldn’t notice this. We try right now … to look at this as a potential biomarker of PFOS exposure.”
The study, led by Josiane Tessmann, a post-doctoral scholar working in Zaytseva’s lab, was published in the journal Chemosphere.
In 2023, the General Assembly passed a law that now requires health plans in Kentucky to cover biomarker testing . The law, sponsored by Rep. Kimberly Poore Moser , R-Taylor Mill, is aimed at lowering cancer mortality in the state.
Biomarker testing “is a way to look for genes, proteins, and other substances (called biomarkers or tumor markers) that can provide information about cancer,” according to the National Cancer Institute .
How to protect yourself
Future research will look at specific diets that can help.
For now, she said, high fiber diets appear to “partially mitigate the harmful effect of these pollutants on liver and also microbiota .” It cannot, however, fully stop the loss of the enzyme.
There are also several water filters that can help reduce PFAS in drinking water, as well as non-PFAS cooking pans.
“You just need to be kind of aware of this. But it’s no need to panic,” Zaytseva said. “Just … carefully look (at) what you buy and what you consume.”
SUPPORT NEWS YOU TRUST.
Our stories may be republished online or in print under Creative Commons license CC BY-NC-ND 4.0. We ask that you edit only for style or to shorten, provide proper attribution and link to our website. AP and Getty images may not be republished. Please see our republishing guidelines for use of any other photos and graphics.

Sarah Ladd is a Louisville-based journalist from West Kentucky who's covered everything from crime to higher education. She spent nearly two years on the metro breaking news desk at The Courier Journal. In 2020, she started reporting on the COVID-19 pandemic and has covered health ever since. As the Kentucky Lantern's health reporter, she focuses on mental health, LGBTQ+ issues, children's welfare, COVID-19 and more.
Kentucky Lantern is part of States Newsroom , the nation’s largest state-focused nonprofit news organization.
Related News

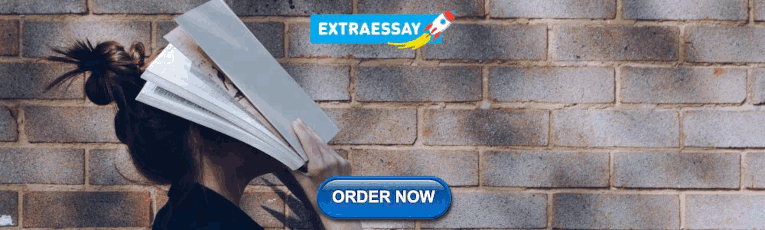
COMMENTS
Advances in Colorectal Cancer Research. Colorectal cells grown into organoids, stem cell-derived human 'mini-organs' that are used to study human development and disease. NCI-funded researchers are working to advance our understanding of how to prevent, detect, and treat colorectal cancer. They are also looking at what factors influence ...
Colorectal cancer, also known as bowel cancer, is a cancer formed by uncontrolled cell growth in the colon or rectum (parts of the large intestine), or in the appendix. Genetic analysis shows that ...
A total of 89.6% of the participants without any advanced colorectal neoplasia (colorectal cancer or advanced precancerous lesions) identified on colonoscopy had a negative cfDNA blood-based test ...
1. Introduction. Colorectal cancer (CRC), which comprises colon and/or rectum cancer, represents a significant health problem as the world's third most commonly diagnosed and second most fatal cancer globally [].Approximately 9.4% of cancer-related deaths were due to CRC in 2020 [].However, in light of the significant increase in the number of identified cases in the older population, it is ...
Colon cancer is the disease characterized by the development of malignant cells in the lining or epithelium of the first and longest portion of the large intestine. Latest Research and Reviews
1. Introduction. Globally, colorectal cancer (CRC) is the third most commonly diagnosed cancer (with approximately 1.9 million cases) and the second most common cause of death (with approximately 0.9 million deaths worldwide) in 2020 [].Thus, colorectal cancer (CRC) accounts for about 10% of cancer cases and deaths, thus constituting a major component of the global cancer burden [].
Researchers have identified four warning signs that they believe may help identify colorectal cancer early in younger adults. The signs or symptoms are abdominal pain, rectal bleeding, diarrhea, and iron deficiency anemia. Drug Regimen Boosts Survival of People with Advanced Colorectal Cancer. Posted: May 24, 2023.
At the National Institutes of Health (NIH), the research effort on colorectal cancer is led by the National Cancer Institute (NCI). A vast network of NCI-supported scientists, located on the NIH campus in Bethesda, Md., and at research centers across the nation and around the world, are exploring new ways to prevent, detect, and treat colorectal cancer.
Supported by research grants from the Research Council of Norway (197309), Nordic Cancer Union, Norwegian Cancer Society, and Health Fund of South-East Norway (5135); bowel preparation free of ...
Over the past two decades of successive clinical trials in metastatic colorectal cancer (CRC), the median overall survival of both control and experimental arms has steadily improved. However, the ...
Researchers have found that the blood of people with colorectal cancer often contains cancer cells, as well as pieces of DNA from the cancer. Removing blood and testing it for cancer (cells or DNA) is sometimes referred to as a liquid biopsy. Researchers are studying whether liquid biopsy samples can be tested for specific gene or protein ...
Our research program is a critical driving force in our mission to end colorectal cancer in our lifetime. We've invested nearly $5 million in colorectal cancer research, including nearly $3 million as early-stage grants to innovative researchers. Learn more about how our funding mechanisms guide our investments in colorectal cancer research.
Several decades ago, colorectal cancer was infrequently diagnosed. Nowadays, it is the world's fourth most deadly cancer with almost 900 000 deaths annually. Besides an ageing population and dietary habits of high-income countries, unfavourable risk factors such as obesity, lack of physical exercise, and smoking increase the risk of colorectal cancer. Advancements in pathophysiological ...
Colorectal cancer is one of the most common cancers worldwide. 1 In the United States, 147,000 individuals received a diagnosis of the disease in 2020, and 53,200 died from it. 2 Most patients with colorectal cancer are older than 50 years of age at diagnosis. 2 Men have a higher risk than do women and are on average 5 to 10 years younger than women when they receive the diagnosis. 3,4
Colorectal cancer (CRC) is the second leading cause of cancer deaths in the United States. The Stanford Cancer Institute has labeled it a priority cancer. In observance of Colorectal Cancer Awareness Month and to learn more about recent advancements in the treatment and knowledge of CRC, we talked to SCI members George A. Fisher Jr., MD ...
The Colorectal Cancer Facts & Figures 2023-2025 report is an educational companion for Colorectal Cancer Statistics, 2023, a scientific paper published in the American Cancer Society flagship journal, CA: A Cancer Journal for Clinicians. The incidence of colorectal cancer is declining in people ages 65 and older.
55% of Colorectal Cancer Cases & Deaths Have the Potential to Be Prevented with Healthy Lifestyle Choices. Colorectal cancer is the 3rd most common cancer worldwide and the 2nd leading cause of death from cancer. It's estimated that about 55% of colorectal cases and deaths could be attributed to modifiable risk factors, such as certain diets ...
Colorectal cancer is a multifactorial disease. It is the third most frequently diagnosed cancer, and the second most common cause of cancer-related deaths worldwide. The etiology of colorectal cancer remains unclear. Although early diagnosis can significantly improve the prognosis, colorectal cancer patients often have no typical clinical manifestations, or display only non-specific signs in ...
Colorectal Cancer. Colon cancer is cancer that forms in the tissues of the first part of the large intestine. Rectal cancer is a malignancy that develops in the rectum, which along with the anal canal, makes up the last 6- to 8-inch part of the large intestine. These cancers are often referred to together as colorectal cancer.
This study reviews the current research trends and hotspots in CRC and epigenetics, which can promote the development of this field and provide references for researchers in this field. ... Vitamin D and gut microbiota mediate colorectal cancer and epigenetics, and probiotics may reduce colorectal cancer-related symptoms. ...
Genome-wide association studies of colorectal cancer (CRC) have identified 170 autosomal risk loci. ... (ICR), this work was supported by Cancer Research UK (CRUK) (C1298/A25514 to R.S.H.), the ...
Detailed information about bowel cancer (colorectal cancer). Learn about causes, symptoms, treatment and survival rates, as well as getting diagnosed and support. ... Cancer Research UK is a registered charity in England and Wales (1089464), Scotland (SC041666), the Isle of Man (1103) and Jersey (247). A company limited by guarantee.
Colorectal cancer (CRC) is one of the most common malignancies and causes of cancer-related deaths, being the third most common cancer and the second most common cause of cancer death worldwide. ... The author declare that the research was conducted in the absence of any commercial or financial relationships that could be construed as a ...
Colitis-associated colorectal cancer (CAC) frequently develops in patients with inflammatory bowel disease (IBD) who have been exposed to a prolonged state of chronic inflammation. The investigation of pharmacological agents and their mechanisms to prevent precancerous lesions and inhibit their progression remains a significant focus and challenge in CAC research. Previous studies have ...
The SOLARIS trial was inspired by previous research suggesting that higher levels of vitamin D in the blood are associated with improved survival for metastatic colorectal cancer and that the ...
The colon and rectum. To understand colorectal cancer, it helps to know about the normal structure and function of the colon and rectum. The colon and rectum make up the large intestine (or large bowel), which is part of the digestive system, also called the gastrointestinal (GI) system (see illustration below).. Most of the large intestine is made up of the colon, a muscular tube about 5 feet ...
Significance: The SOLARIS trial was inspired by previous research suggesting that higher levels of vitamin D in the blood are associated with improved survival for metastatic colorectal cancer and that the addition of high-dose vitamin D3 to standard therapy could potentially improve progression free survival. The SOLARIS results suggest ...
Significance: The SOLARIS trial was inspired by previous research suggesting that higher levels of vitamin D in the blood are associated with improved survival for metastatic colorectal cancer and ...
Help us end cancer as we know it, for everyone. Cancer information, answers, and hope. Available every minute of every day. Learn where colorectal cancer starts, ways to prevent it, tests to diagnose it, the options for treatment, and what to do after you have finished treatment.
Over a lifetime, Kentuckians are repeatedly exposed to environmental pollutants known as " forever chemicals " — and a new study links such exposure to colorectal (colon) cancer. Specifically, researchers at the University of Kentucky looked at long-term exposure to PFOS (perfluorooctane sulfonic acid) in mice and confirmed their findings in human cells.