Accessibility Links
- Skip to content
- Skip to search IOPscience
- Skip to Journals list
- Accessibility help
- Accessibility Help
Click here to close this panel.

As a society-owned publisher with a legacy of serving scientific communities, we are committed to offering a home to all scientifically valid and rigorously reviewed research. In doing so, we aim to accelerate the dissemination of scientific knowledge and the advancement of scholarly communications to benefit all.
Nano Express supports this mission and actively demonstrates our core values of inclusive publishing and trusted science . To find out more about these values and how they can help you publish your next paper with us, visit our journal scope .
Purpose-led Publishing is a coalition of three not-for-profit publishers in the field of physical sciences: AIP Publishing, the American Physical Society and IOP Publishing.
Together, as publishers that will always put purpose above profit, we have defined a set of industry standards that underpin high-quality, ethical scholarly communications.
We are proudly declaring that science is our only shareholder.
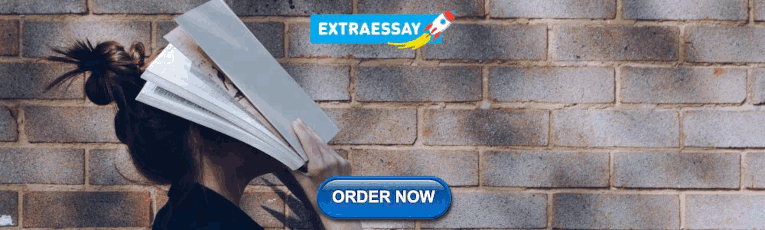
Hydrothermal synthesis and characterization of Zinc Oxide nanoparticles of various shapes under different reaction conditions
Sonima Mohan 1,2 , Mini Vellakkat 4,1 , Arun Aravind 3 and Reka U 1
Published 30 November 2020 • © 2020 The Author(s). Published by IOP Publishing Ltd Nano Express , Volume 1 , Number 3 Citation Sonima Mohan et al 2020 Nano Ex. 1 030028 DOI 10.1088/2632-959X/abc813
Article metrics
17984 Total downloads
Share this article
Author e-mails.
Author affiliations
1 Dept. of Physics, AMC Engineering College, 18 KM, Kalkere, Bannerghatta Main Rd, Bangalore–560083, India
2 Dept. of Science, St. Francis De Sales College, Electronic City, Bangalore–560100, India
3 Dept. of Physics, Bishop Moore College, Mavelikkara, Kallumala–690110, India
Author notes
4 Author to whom any correspondence should be addressed.
Mini Vellakkat https://orcid.org/0000-0001-9312-0340
Arun Aravind https://orcid.org/0000-0003-1942-5343
- Received 4 August 2020
- Revised 15 October 2020
- Accepted 5 November 2020
- Published 30 November 2020
Peer review information
Method : Single-anonymous Revisions: 1 Screened for originality? Yes
Buy this article in print
Zinc Oxide (ZnO) nanoparticles were synthesized by hydrothermal method under different conditions and studied various properties. FTIR studies proved the presence of ZnO bonding and purity of the samples. Grain size was found to be decreased with the increase of reaction temperature and increased with reaction time. TEM images show formation of nanorods under same reaction temperature, also nanoflowers and nanospheres for different temperatures. Intensity of luminescence peaks is found to be changed with variation in interplanar spacing. UV–vis spectra helped to identify the increased photon absorption in particles of bigger size. Change in bandgap value is also observed due to the difference in size of nanoparticles.
Export citation and abstract BibTeX RIS
Original content from this work may be used under the terms of the Creative Commons Attribution 4.0 licence . Any further distribution of this work must maintain attribution to the author(s) and the title of the work, journal citation and DOI.
1. Introduction
Nanoparticles of transition metal oxide semiconductors exhibit unique electrical, optical and mechanical properties. These nanostructures have wide applications in different fields based on their size and shape [ 1 – 3 ]. Nanoparticles of various morphology like- nanorods, nanosheets, nanobelts etc can be utilized for solar cells, light emitting diodes, gas sensors, and biological probes. Size, shape, lattice constant and crystallinity of nanoparticles have very important role in choosing these materials for different applications [ 4 ]. Research work in the field of renewable energy sources has already been started since energy crisis is one of the most important issues which is expected to happen in near future [ 5 , 6 ]. Nanoparticles of ZnO, NiO, TiO 2 etc exhibit excellent optical and electronic properties which are the main requirements for the functioning of optoelectronic devices [ 7 – 9 ] Structural and optical characterization help us in suggesting materials for fabrication units.
Zinc Oxide is a transition metal oxide semiconductor with wide bandgap and high exciton binding energy. Hence ZnO is one of the most preferable materials in optoelectronic research field [ 10 – 18 ]. It is a low-cost material and easily available in nature [ 19 ]. ZnO nanoparticles are transparent to visible part of light and absorbing UV radiations. It is less toxic, high resistant and durable material [ 20 , 21 ]. Morphology of ZnO nanoparticles can be modified easily [ 22 ]. Electron mobility is high for ZnO nanostructures [ 23 ].
Many techniques like chemical vapour deposition, spray pyrolysis, sol-gel method, hydrothermal method etc are already available for the synthesis of nanomaterials. Preparation techniques play a very important role in determining the size and shape of nanoparticles [ 18 , 24 ]. Hydrothermal method has been attracted many researchers because of its distinct advantages like simple equipment, low cost and mild preparation conditions [ 25 ]. It is an environment friendly technique. Size and shape of nanomaterials can be modified by hydrothermal method. Morphology of nanoparticles mainly depends on reaction time, temperature and concentration of reacting solutions. This in turn affects the physical and chemical properties of nanoparticles. Particles are choosing for various applications according to their morphology. In this work we mainly emphasize the effect of reaction parameters on structural and optical properties of nanoparticles. Here hydrothermal reaction time is varied from 1 h to 5 h and temperature from 100 °C to 150 °C. Ph of solution mixture is kept as 12 to understand its influence on nanomaterial growth [ 22 , 26 – 29 ]. In nano level, minute change in morphology of the particles affects the structural and optical properties drastically. We can consider reaction parameters as the tuning factors for the production of nanomaterial according to our requirement.
2. Experimental details
2.1. materials.
Zinc Nitrate [Zn (NO 3 ) 2 .6H 2 O] and Sodium Hydroxide (NaOH) pellets from Sigma-Aldrich. All chemicals used were analytical grade without further purification. Distilled water was used throughout the experiment.
2.2. Synthesis
Hydrothermal method is opted for the synthesis of nanoparticles. 0.5 M Zinc Nitrate solutions were prepared in 30 ml distilled water under stirring for 30 min. Meanwhile 5 M Sodium Hydroxide solutions were prepared by mixing weighed pellets of NaOH in 30 ml distilled water under stirring for same duration. NaOH solution is added dropwise to former solution under continuous stirring until Ph of the reactants becomes 12 [ 3 , 27 , 29 – 32 ]. This solution mixture was transferred into Teflon lined sealed stainless-steel autoclaves and kept in hydrothermal oven at a temperature of 100 °C for 2 h. Then the beaker was taken outside and allowed to cool naturally to room temperature. The resultant solution was filtered, then washed with distilled water and kept for drying under lamp. The experimental procedure is repeated for 125 °C and 150 °C by keeping 2 h duration as constant and samples were named as HTZNP1, HTZNP2 and HTZNP3 respectively. Samples were also prepared by varying the reaction time and keeping temperature as constant. Samples synthesized at a temperature of 120 °C for a reaction time of 1 h, 3 h and 5 h were named as HTZNP4, HTZNP5 and HTZNP6. The method of preparation for the nanoparticles is shown in scheme 1 .
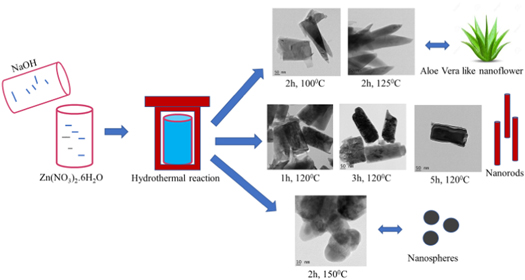
Scheme 1. Hydrothermal synthesis of various nanostructures under different reaction parameters.
Download figure:
2.3. Characterization details
Crystal structure and average crystallite size were measured by XRD Bruker D8 Advance. Presence of metal oxide bonding and purity of samples were confirmed by FTIR Thermo Nicolet Avtar 370 (4000 cm −1 to 400 cm −1 ). Morphology of the samples was observed by SEM Jeol 6390LA/OXFORD XMX N and HRTEM: Jeol/JEM 2100. Thermal stability was determined by TGA Perkin Elmer STA6000. UV absorption was measured by JascoV-760 spectrophotometer. Photoluminescence is observed by Fluoromax 4 spectrofluorometer.
3. Results and discussion
3.1. ftir analysis.
FTIR studies help us to analyse the possible bonding between neighbouring structures in practical applications [ 18 ]. FTIR measurements were carried out for a range of 4000–400 cm −1 and are shown in figure 1 . In this range bands are due to vibration of ions in crystal lattice [ 33 ]. The intense band near 3439 cm −1 in all samples indicates presence of OH stretching vibration. This may be due to oscillation of water molecule [ 3 , 14 , 18 , 34 , 35 ]. It is shifted towards higher wave number side and intensity is more for HTZNP2 sample. Also, this band is appeared as narrow for HTZNP2, but widened for HTZNP1 and HTZNP3. This is because water content and its energy may be more at a temperature of 125 °C. Broadening of this band occurred in HTZNP1 and HTZNP 3 may be attributed to decrease in particle size [ 11 ]. There is no shift in this wave number for samples HTZNP4, HTZNP5 and HTZNP6 which are prepared at same temperature. Band at 2925 cm −1 which arises due to –CH 2 vibration is present only in HTZNP2, HTZNP5 and HTZNP6 [ 34 , 35 ]. The band at 1633 cm −1 shows presence of C=O functional group [ 34 ]. There is no shift in this band due to change in reaction time and temperature. But intensity of peak is observed to be more for HTZNP3. This also can be due to small particle size [ 11 ]. The band at 1560 cm −1 belongs to symmetric C=O stretching [ 18 ]. In figure 1 (a), this band is shifted to higher wave number side for HTZNP2 and in figure 1 (b), this is shifted to higher wave number side for HTZNP5. This is assigned to minute change in bond length [ 11 ]. The bands at 1507, 1383 and 831 cm −1 arise due to vibration and bending modes of CO 3 2− [ 3 , 14 , 18 , 36 ]. These bands are not visible in HTZNP3. Disappearance of these bands at high temperature indicates complete decomposition of precursors. Increased concentration at low temperature causes high intensity for HTZNP 1 [ 14 , 18 ].
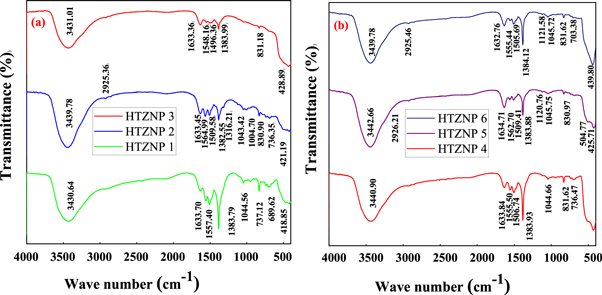
Figure 1. FT-IR spectra of (a) HTZNP1, HTZNP2 and HTZNP3 (b) HTZNP4, HTZNP5 and HTZNP6.
Band at 1120 is present only for HTZNP5 and HTZNP6. This may be due to O–H deformation [ 37 , 38 ]. The band close to 1043 cm −1 shows –CH 2 vibration. It is present in all samples except HTZNP3. Disappearance of this band at high temperature may be ascribed to change in particle size. Band near 736 cm −1 is found in HTZNP1, HTZNP2 and HTZNP4 due to H–O–H bending vibration which indicates the presence of water of crystallization [ 13 , 30 ]. This band is absent in other samples. Due to high temperature and more duration, changes might have happened in this bond. Band at 688 cm −1 is due to deformation vibration. It is present in HTZNP1 and HTZNP2, but absent in HTZNP3 [ 31 ]. Band present at 418 cm −1 which belong to Zn–O stretching mode is shifted to higher wave number region as temperature is increased [ 39 ]. This is attributed to increase in stretching energy due to increased temperature. Reaction temperature influence vibration of various functional groups present in samples. High temperature may affect morphology of nanoparticles which in turn causes intensity variation of bands. Increase in temperature causes increase in vibrational energy of Zn–O bond and hence a shift in band towards higher wavenumber side [ 39 ].
3.2. XRD analysis
Crystal structure of the samples were analysed by using XRD spectrometer. XRD is helpful for determining crystallite size, sample purity and lattice strain due to dislocation. Peaks are observed at 2 θ values of 31.52, 34.15, 35.95, 47.28, 56.28, 62.52, 66.11, 67.68, 68.83, 72.22 and 76.84. The intense peaks appeared at different 2 θ values indicate hexagonal wurtzite structure of ZnO nanoparticles. Peaks and intensities are matching with literature values and are shown in figure 2 [ 18 , 30 , 35 , 38 , 40 , 41 ]. Intensity of (101) plane is found to be maximum for all six samples. Intensity of peak corresponding to (100) plane is found to be increased for samples synthesized at same temperature under different reaction time. Absence of other peaks indicates purity of samples.
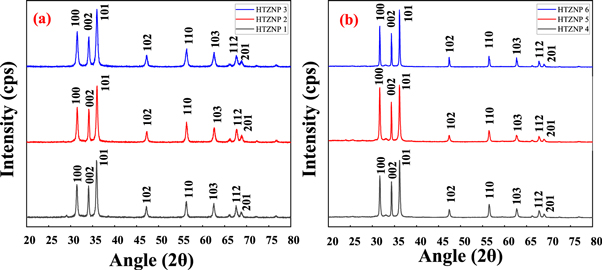
Figure 2. XRD patterns of (a) HTZNP1, HTZNP2 and HTZNP3 (b) HTZNP4, HTZNP5 and HTZNP6.
In figure 2 (a), intensity of (101) is appeared as lowest for HTZNP3. Diffraction angle is found to be right shifted for this sample. This can be assigned to stress developed and defects formed inside the material. In figure 2 (b), it was observed that intensity is less for HTZNP5. Sharpness of diffraction peaks corresponding to (100), (002) and (101) planes are observed to be increased for nanoparticles formed at same temperature which indicates good crystallinity of samples [ 42 – 44 ].
Average grain size of ZnO is determined by using equation ( 1 )-Debye–Scherrer formula
Where D is the crystallite size, λ is the incident radiation wave length, β is the full width at half- maximum of the ZnO (101) line and θ is the diffraction angle [ 3 , 12 , 18 , 26 , 35 , 45 ]. Grain size was determined for various samples. When temperature is increased, crystallite size is found to be decreased. When reaction time is increased, grain size is increased. Grain size and FWHM of samples are shown in table 1 .
Table 1. Grain size and FWHM of samples prepared.
3.3. SEM analysis
SEM images of hydrothermally prepared samples are shown in figure 3 . Nanostructures with various size and shape are obtained under different reaction parameters. It is observed that reaction temperature and duration influence morphology very much and hence the structural properties of nanoparticles. By varying reaction parameters 0D, 1D, 2D and 3D structures are obtained. SEM reveals the flower like structure (3D) to first two samples (figures 3 (a), (b)), spherical granular shape (0D) to HTZNP3 (figure 3 (c)) and nanorod (1D) like morphology to HTZNP4, HTZNP5 and HTZNP6 (figure 3 (d)–(f)). HTZNP1 (2D) has nanosheets joined to form flower structure [ 2 , 3 , 29 , 39 , 46 , 47 ]. Aloe Vera like pattern with more uniformity of flower like pattern is very clear in HTZNP2.
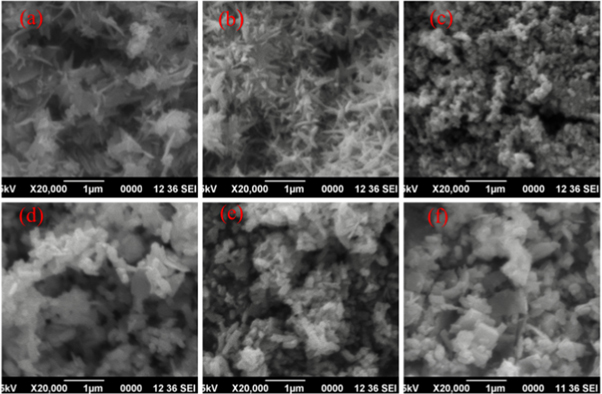
Figure 3. SEM images of (a) HTZNP1 (b) HTZNP2 (c) HTZNP3 (d) HTZNP4 (e) HTZNP5 and (f) HTZNP6.
From FTIR analysis it is observed that intensity of peak 1383 cm −1 is appeared to be maximum for smaller rods. This is because carboxylate group exists at the surface. As size of the particle increases intensity of the peak decreases. Vibration bands due to CO 3 2− and –CH 2 groups and deformation vibration bands are absent in nanoparticles of spherical shape [ 35 ].
Sharpness of XRD peaks corresponding to (100), (002) and (101) planes are found to be increased for nanorods [ 42 – 44 , 46 ]. Position of diffraction angle shift, intensity variation in XRD peaks and presence/absence of some bands in FTIR also predicted possibility of change in morphology and size of nanoparticles.
3.4. TEM analysis
TEM images of hydrothermally grown samples are shown in figures 4 – 9 . Flower shaped ZnO nanoparticles were obtained for 100 °C and 125 °C. Length of flower petal is obtained as 413 nm and diameter 78 nm for HTZNP1. Length is 495 nm and diameter 131 nm for HTZNP2. When temperature is increased to 150 °C nano particle is changed to spherical in shape. Average size of HTZNP3 is obtained as 40 nm. Among the three samples synthesized for same duration, size of HTZNP3 at high temperature is found to be less. Nanostructures synthesized at same temperature are having same morphology [ 32 ]. Length of HTZNP4 is obtained as 312 nm and diameter as 160 nm. For HTZNP5, length is found to be 160 nm and diameter 70 nm. HTZNP6 is seen with a length of 216 nm and diameter 112 nm. From XRD graphs it is clear that intensity of (100) plane is increased for nanoparticles of rod morphology. Also, intensity of peak corresponding to (101) plane is found to be maximum for nanorods of bigger size and peak intensity is minimum for smaller rods. Intensity of all peaks is found to be minimum for particles of smaller size. Interplanar spacing is found to be 0.23 nm for HTZNP1, HTZNP3, HTZNP4 and HTZNP5 and 0.25 nm for HTZNP2 and HTZNP6. Interplanar spacing is matching with literature [1, 26, 37, 44, 46 ]. From SAED pattern it is clear that HTZNP1 and HTZNP2 are polycrystalline and other samples are single crystalline in nature [ 48 ].
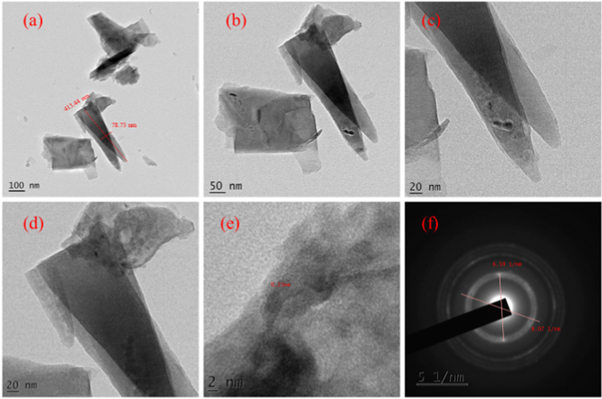
Figure 4. TEM images and SAED image of HTZNP1.
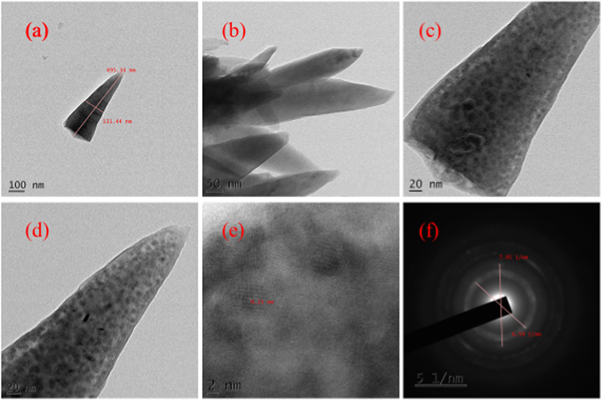
Figure 5. TEM images and SAED image of HTZNP2.
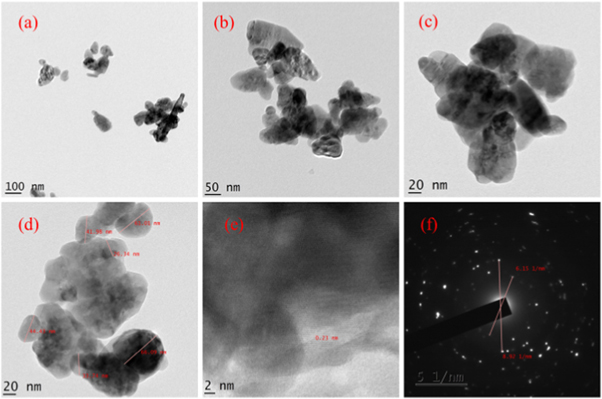
Figure 6. TEM images and SAED image of HTZNP3.
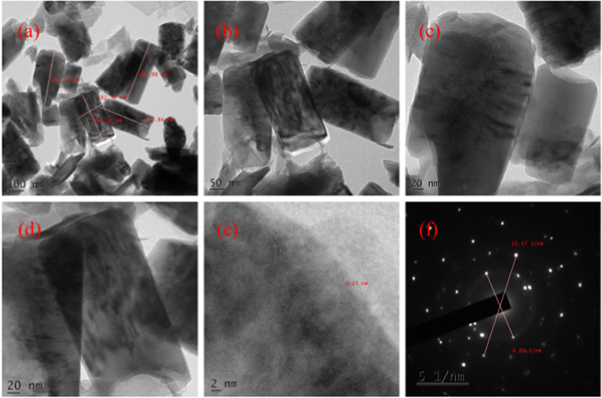
Figure 7. TEM images and SAED image of HTZNP4.
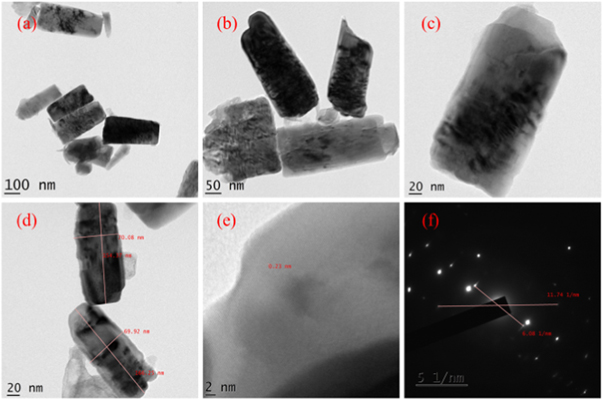
Figure 8. TEM images and SAED image of HTZNP5.
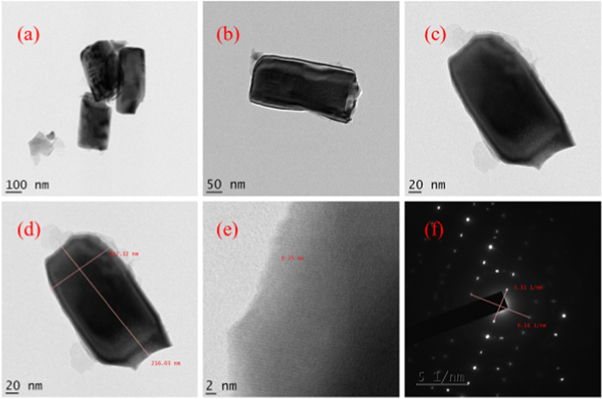
Figure 9. TEM images and SAED image of HTZNP6.
3.5. TGA analysis
Thermogravimetric analysis (TGA) helps us to study thermal behaviour of samples. In figure 10 (a), it is shown that first decomposition started at a temperature of 210 °C for HTZNP1 and at 270 °C for HTZNP2 and HTZNP3. It is observed that sample synthesized at high temperatures decayed at higher temperature. Above 80% of material was existing even after heating up to 700 °C. Thermal decomposition of rod-shaped nanoparticles is shown in figure 10 (b). Only 5% of the material is decomposed and it is started only at 200 °C. After 250 °C material was almost stable. All nanorods are showing same type of thermal behaviour. Less than 2% weight loss happened below 200 °C in all samples is due to desorption of physically adsorbed water present in samples [ 18 , 29 , 36 ]. Thermal degradation of poly crystalline samples HTZNP1 and HTZNP2 is more comparing to other samples. Weight loss is observed to be large in Poly crystalline nanoparticles than single crystalline particles. Hence single crystalline materials are thermally stable as observed in literature [ 13 ].
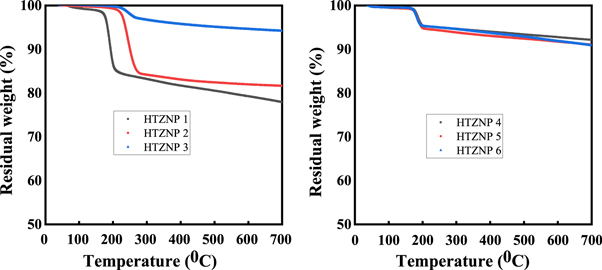
Figure 10. TGA curves of (a) HTZNP1, HTZNP2 and HTZNP3 (b) HTZNP4, HTZNP5 and HTZNP6.
3.6. PL analysis
Physical and optical properties of semiconducting particles will change due to quantum size effects [ 48 ]. Photoluminescence spectroscopy (PL) provides information about extrinsic and intrinsic defects. It gives information about different energy states existing in between valance band and conduction band [ 46 , 49 ]. PL spectra of ZnO nanoparticles exhibit two emission bands: one is in UV and one is in visible region [ 29 , 44 ]. PL spectra were observed by using spectrofluorometer with an excitation wave length of 350 nm at room temperature. Peaks appeared in PL emission spectrum in the range from 370 nm to 500 nm indicates transition of electrons from bottom of conduction band to zinc vacant energy levels. UV emission peak appeared in various samples from 378–387 nm corresponds to near band edge emission of ZnO. This is due to radiative recombination of free excitons [ 9 , 29 , 33 , 50 ]. Different peaks spread between 430 to 500 nm can be assigned to recombination of photogenerated holes with singly ionized state related to defects [ 9 , 14 ]. Blue emission band appeared near 477 nm is caused by transition of electron from conduction band to zinc interstitial oxygen defects present in the sample [ 51 ]. Violet band observed near 416 nm in all samples is attributed to recombination of electron at the zinc interstitial and a hole in the valance band. Other violet peak present near 405 nm is referred to transition from Zinc interstitial to Zinc vacancy and peak at 382 is due to near band edge emission [ 52 ]. Oxygen vacancies, Zinc vacancies, Oxygen interstitials and Zinc interstitials are main defects which are attributed to visible emission [ 53 ].
In figure 11 (a), we can see that when temperature is increased from 100 to 125 °C, intense peak present in UV region is slightly shifted to shorter wave length region. This can be interpreted as increase of exciton energy due to decrease in grain size when reaction temperature is increased. Different behaviour of HTZNP3 graph is caused by its spherical nature. Higher UV emission intensities observed for HTZNP1 and HTZNP2 can be assigned to larger grain size and flower like structure. Intensity of this band is comparatively less for HTZNP3. Excited electrons lose their energy mainly by two ways—radiative recombination and nonradiative recombination. Non radiative trapping rate of excited charge carriers may be high for spherical nanoparticles. This can be the reason for less photoluminescence intensity in UV region. Maximum intensity peaks observed for HTZNP2 confirmed the formation of large number of defects in the sample [ 46 ]. In figure 11 (b), all three graphs are similar in nature due to same shape. There is a red shift for peak in UV region from HTZNP4 to HTZNP6. This is ascribed to the increase in crystallite size from HTZNP4 to HTZNP6 as shown in XRD analysis. One broad band centred at 456 nm can be seen in all the three samples. Intensity of this peak is found to be increased when reaction time is increased. This is due to increase in grain size. All results are matching with literature [ 46 , 47 , 54 ]. Blue emission band is observed at 428 for HTZNP1, 431 for HTZNP4 and HTZNP5 and at 432 for HTZNP6. This can be explained as near band edge emission due to free exciton recombination. The emission band occurred at about 486 nm can be referred as deep level emission. Presence of intrinsic and extrinsic defects which arises due to electron recombination in Oxygen vacancy with hole in valance band is the main reason for this band [ 55 ]. Small peaks at 476, 486 and 496 nm are attributed to the presence of more surface defects, Zinc and Oxygen interstitials in these three samples. Here also intensity is maximum for 5 h and minimum for 1 h. This can be assigned to the formation of more surface defects, Zinc and Oxygen interstitials due to more reaction time [ 27 , 46 ]. From figure 11 (a), it is clear that luminescence observed for HTZNP2 in UV and visible regions is more than other two samples. Among samples prepared under same temperature a slight increase in luminescence is observed for HTZNP6 and is shown in figure 11 (b). TEM analysis is showing an interplanar spacing of 0.25 nm for these two samples. Other samples are appeared with an interplanar spacing of 0.23 nm. High luminescence of HTZNP2 and HTZNP6 may be due to structural difference occurred as a result of variation in interplanar spacing. Full-width at half maximum is only few nm for the peaks. So, size of the particle is in nano range [ 56 ].
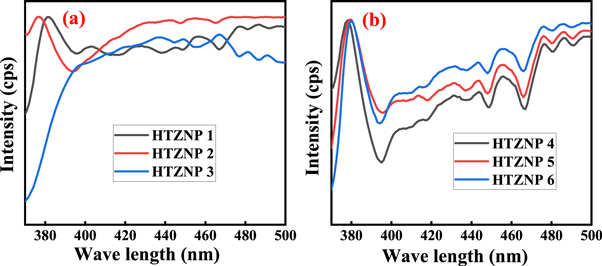
Figure 11. Photoluminescence spectra of (a) HTZNP1, HTZNP2 and HTZNP3 (b) HTZNP4, HTZNP5 and HTZNP6.
3.7. UV analysis
UV absorption spectra is analysed for all samples from 200 nm to 800 nm. Peaks observed in the absorption spectra are due to transition of electrons between valance band, conduction band and intrinsic defect levels [ 46 ]. In figure 12 (a), an intense broad peak is observed for HTZNP1 and HTZNP2 from 360–380 nm. This is the characteristic absorption peak of ZnO which is ascribed to intrinsic band-gap absorption of ZnO. But this is widened for HTZNP3. This may be due to its spherical shape and smaller size. Absorption is found to be maximum for HTZNP2. This may be referred to its larger size and flower like 3D structure.
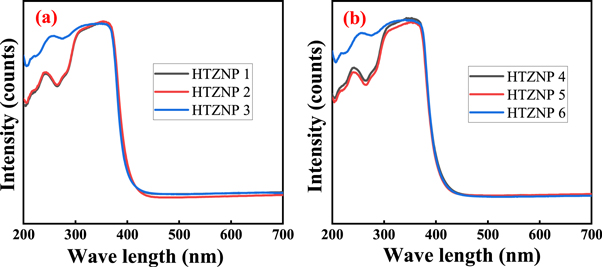
Figure 12. UV–vis absorption spectra of (a) HTZNP1, HTZNP2 and HTZNP3 (b) HTZNP4, HTZNP5 and HTZNP6.
In figure 12 (b), peak in UV region is found to be at 242 nm for HTZNP4 and HTZNP5. But for HTZNP6 it is at 259 nm. This red shift is due to increase in grain size. Here also an intense peak is observed at 360–380 nm. Maximum absorption is observed for HTZNP4. Size of nanorod is found to be maximum for HTZNP4. When particle size increases visible absorption increases. This may be due to the increase in number of Oxygen vacancies [ 22 , 54 , 57 ].
UV–vis spectra for determination of bandgap is given in figures 13 and 14 . From figure 13 , bandgap of HTZNP1 is found to be 3.14 eV, 3.12 eV for HTZNP2 and 3.18 eV for HTZNP3. Bandgap of HTZNP2 is found to be minimum. In figure 14 , bandgap of HTZNP4 is obtained as 3.13 eV, 3.11 eV for HTZNP5 and 3.10 eV for HTZNP6. HTZNP6 is having minimum bandgap. PL intensity of HTZNP2 and HTZNP6 is found to be maximum. Bandgap value is minimum for these samples. Interplanar spacing is found to be maximum for these two samples. Variation in bandgap can also be occurred due to size difference [ 29 , 41 ].
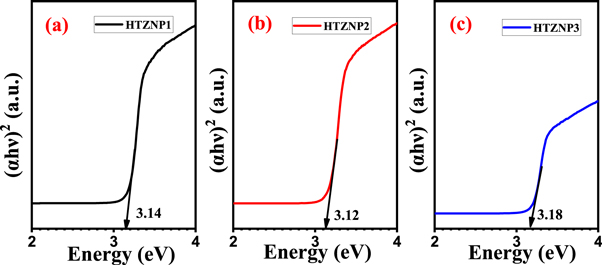
Figure 13. Bandgap determination from UV–vis absorption spectra of (a) HTZNP1, (b) HTZNP2 and (c) HTZNP3.
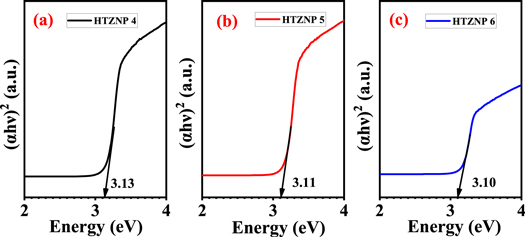
Figure 14. Bandgap determination from UV–vis absorption spectra of (a) HTZNP4, (b) HTZNP5 and (c) HTZNP6.
3.8. Strain calculations from W-H plot
Strain is developed in nanoparticles due to crystalline imperfections and distortions [ 11 ]. High intensity of photoluminescence peaks, smaller bandgaps and large interplanar spacing observed in HTZNP2 and HTZNP6 may be caused by this induced strain. Figures 15 and 16 show W-H plots which are used to determine the strain. Negative slope of the fitted line found in HTZNP2, HTZNP4, HTZNP5 and HTZNP6 is referred to compressive strain and positive slope obtained for HTZNP1 and HTZNP3 indicates tensile strain [ 11 ]. W-H equation for determination of strain is given by equation ( 2 )
Here, θ is the x-ray diffraction angle and β is full width at half maximum obtained from XRD analysis. ε is the slope of the fitted line which indicates strain in the sample [ 11 ]. From figure 15 strain present in HTZNP1 is obtained as 0.093, 0.302 for HTZNP2 and 0.116 for HTZNP3. Strain calculated in HTZNP2 is more than other two samples. Figure 16 shows a strain of 0.115 in HTZNP4, 0.120 in HTZNP5 and 0.152 in HTZNP6. Here HTZNP6 is showing maximum strain. Micro strain is related to lattice misfit, which causes changes in d-spacing [ 46 ].
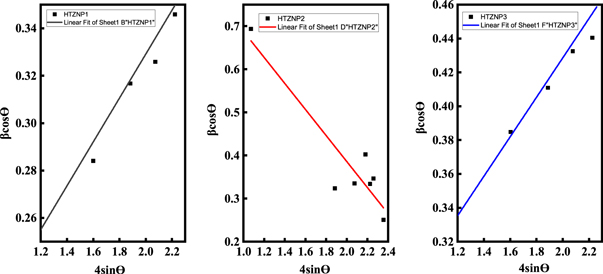
Figure 15. W-H plot of HTZNP1, HTZNP2 and HTZNP3.
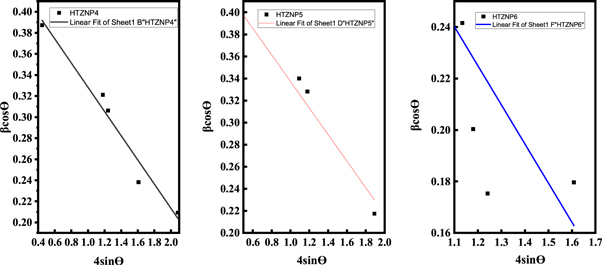
Figure 16. W-H plot of HTZNP4, HTZNP5 and HTZNP6.
Enhanced optical properties (High intensity of photoluminescence peaks, smaller bandgaps) and morphological changes (large interplanar spacing) observed in HTZNP2 and HTZNP6 may be caused by this high value of strain developed in these samples.
4. Conclusions
ZnO nanoparticles were synthesized by hydrothermal method under different reaction conditions. It is observed that reaction parameters play a very important role in determining size and shape of nanoparticles. FTIR study revealed the effect of reaction parameters on vibrations of various functional groups. From TGA it is clear that all samples started to decompose only after 200 °C and increase in reaction temperature pushed this value to a higher temperature 270 °C. XRD and TEM analysis proved the change in structural properties of nanomaterials with respect to the change in reaction time and temperature. When reaction temperature is increased, crystallite size is reduced and a blue shift is observed in PL analysis. It is observed an increase in grain size as a result of increase in reaction time and hence a red shift in photo luminescence. A decrease in bandgap value is observed for samples with more interplanar spacing. PL intensity is appeared maximum for these samples. Strain calculated from W-H plots is maximum for HTZNP2 under same reaction temperature and HTZNP6 under same reaction time.
Hydrothermal synthesis can be chosen as a suitable method for synthesis of different nanostructures with considerable optical properties. Here nanoflowers and nanorods are showing excellent photoluminescence, UV absorption and suitable bandgap. Nanorods are thermally stable than nanoflowers. Optical properties and thermal stability are the key factors for solar cell materials. Based on their optical properties and thermal stability we can propose these hydrothermally synthesised nanorods and nanoflowers for different layers of solar-cell.
Acknowledgments
Authors would like to thank Department of Physics, Bishop Moor College, Mavelikkara, Kerala, India for providing lab facility to complete synthesis part and optical characterization. Also thank Sophisticated Analytical Instrument Facility, STIC, Cochin, Kerala for FTIR, XRD, TGA, SEM, TEM characterization.
Zinc oxide nanoparticles: A comprehensive review on its synthesis, anticancer and drug delivery applications as well as health risks
Affiliations.
- 1 Advance School of Chemical Sciences, Faculty of Basic Sciences, Shoolini University, Solan, Himachal Pradesh 173212, India.
- 2 Advance School of Chemical Sciences, Faculty of Basic Sciences, Shoolini University, Solan, Himachal Pradesh 173212, India. Electronic address: [email protected].
- 3 Division of Molecular Medicine, Bose Institute, P-1/12, CIT Scheme VII M, Kolkata 700054, India. Electronic address: [email protected].
- PMID: 33212389
- DOI: 10.1016/j.cis.2020.102317
In recent years, zinc oxide nanoparticles (ZnONPs) emerged as an excellent candidate in the field of optical, electrical, food packaging and particularly in biomedical research. ZnONPs show cancer cell specific toxicity via the pH-dependent (low pH) dissolution into Zn 2+ ions, which generate reactive oxygen species and induce cytotoxicity in cancer cells. Further, ZnONPs have also been used as an effective carrier for the targeted delivery of several anticancer drugs into tumor cells. The increasing focus on ZnONPs resulted in the development of various synthesis approaches including chemical, pHysical, and green or biological for the manufacturing of ZnONPs. In this article, at first we have discussed the various synthesis methods of ZnONPs and secondly its biomedical applications. We have extensively reviewed the anticancer mechanism of ZnONPs on different types of cancers considering its size, shape and surface charge dependent cytotoxicity. Photoirradiation with UV light or NIR laser further increase its anticancer activity via synergistic chemo-photodynamic effect. The drug delivery applications of ZnONPs with special emphasis on drug loading mechanism, stimuli-responsive controlled release and therapeutic effects have also been discussed in this review. Finally, its side effects to vital body organs with mechanism via different exposure routes, the future direction of the ZnONPs research and application are also discussed.
Keywords: Adverse effects; Anticancer; Drug delivery; Synthesis, zinc oxide nanoparticles.
Copyright © 2020 Elsevier B.V. All rights reserved.
Publication types
- Antineoplastic Agents / adverse effects
- Antineoplastic Agents / chemical synthesis
- Antineoplastic Agents / chemistry
- Antineoplastic Agents / pharmacology
- Chemistry Techniques, Synthetic
- Drug Carriers / adverse effects
- Drug Carriers / chemical synthesis
- Drug Carriers / chemistry
- Drug Carriers / pharmacology
- Nanoparticles*
- Zinc Oxide / adverse effects
- Zinc Oxide / chemistry*
- Zinc Oxide / pharmacology*
- Antineoplastic Agents
- Drug Carriers
Thank you for visiting nature.com. You are using a browser version with limited support for CSS. To obtain the best experience, we recommend you use a more up to date browser (or turn off compatibility mode in Internet Explorer). In the meantime, to ensure continued support, we are displaying the site without styles and JavaScript.
- View all journals
- My Account Login
- Explore content
- About the journal
- Publish with us
- Sign up for alerts
- Open access
- Published: 20 February 2024
Biosynthesis and biocompatibility evaluation of zinc oxide nanoparticles prepared using Priestia megaterium bacteria
- Mona A. Ashour 1 &
- Basma T. Abd-Elhalim ORCID: orcid.org/0000-0002-3009-332X 1
Scientific Reports volume 14 , Article number: 4147 ( 2024 ) Cite this article
658 Accesses
1 Altmetric
Metrics details
- Biotechnology
- Nanobiotechnology
- Nanoscience and technology
The current study aimed to find an effective, simple, ecological, and nontoxic method for bacterial green synthesis of zinc oxide nanoparticles (ZnONPs) using the bacterial strain Priestia megaterium BASMA 2022 (OP572246). The biosynthesis was confirmed by the change in color of the cell-free supernatant added to the zinc nitrate from yellow to pale brown. The Priestia megaterium zinc oxide nanoparticles (Pm/ZnONPs) were characterized using UV–Vis spectroscopy, high-resolution transmission electron microscopy (HR-TEM), energy-dispersive X-ray spectroscopy (EDX), Fourier transform infrared spectroscopy (FTIR), and zeta potential. The Pm/ZnONPs characterization showed that they have a size ranging between 5.77 and 13.9 nm with a semi-sphere shape that is coated with a protein-carbohydrate complex. An EDX analysis of the Pm/ZnONPs revealed the presence of the shield matrix, which was composed of carbon, nitrogen, oxygen, chlorine, potassium, sodium, aluminum, sulfur, and zinc. The results of the FTIR analysis showed that the reduction and stabilization of the zinc salt solution were caused by the presence of O–H alcohols and phenols, O=C=O stretching of carbon dioxide, N=C=S stretching of isothiocyanate, and N–H bending of amine functional groups. The produced ZnONPs had good stability with a charge of − 16.2 mV, as evidenced by zeta potential analysis. The MTT assay revealed IC 50 values of 8.42% and 200%, respectively, for the human A375 skin melanoma and human bone marrow 2M-302 cell lines. These findings revealed that the obtained Pm/ZnONPs have the biocompatibility to be applied in the pharmaceutical and biomedical sectors.
Similar content being viewed by others
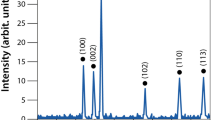
Antibacterial action and target mechanisms of zinc oxide nanoparticles against bacterial pathogens
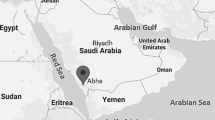
Bio fabrication of silver nanoparticles with antibacterial and cytotoxic abilities using lichens
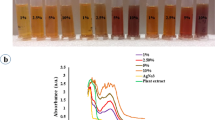
Comparative assessment of the biological activity of the green synthesized silver nanoparticles and aqueous leaf extract of Perilla frutescens (L.)
Introduction.
It is well known that supplemental minerals and their nanoform derivatives are safe and biocompatible to consume by humans and that they have broad-spectrum antimicrobial activity at low doses 1 . Because it may be possible to create effective bio-nano materials with precise biological activities, zinc and its oxide nanoparticle substances are gaining increasing attention 2 , 3 . Comparing the green amalgamation of NPs to physical and chemical synthesis approaches, it has been shown that it is less hazardous, requires less work, and is environmentally benign. Nowadays, there is a significant push to innovate green biosynthesis techniques to produce a high yield of biocompatible nanoparticles. ZnO particles are generally utilized in testing biological methods and analyzing them in many biomedical applications. ZnO is one of the most significant semiconducting resources due to its diverse applications and exceptional properties. ZnO is a substance that may be found in nature and is biocompatible, which is necessary, especially for uses in the biomedical industry 3 , 4 . There is now a significant quantity of knowledge available thanks to recent studies on biological nanoparticles. Reductants, solvents, and ligating agents for nanoparticle formation have all been thoroughly studied to achieve the biological nanoscience goals mentioned above 5 . Natural, environmentally friendly nanoparticle amalgamation techniques avoid using any dangerous chemicals throughout the amalgamation process. As a result, these developed methods that rely on naturally occurring biomaterials offer a choice in how to get the nanoparticles that the industry needs. The synthesis of ZnONPs with inorganic complexes utilizing naturally occurring ligating agents derived from biological resources is one of the main areas of interest for the research of biological nanostructures 6 . In addition to the qualities already described, these ZnONPs that have been combined with bacterial extracts and supernatants are also known to possess beneficial antibacterial, anti-inflammatory, and anticancer qualities 7 . Extracellular biomass-free production was investigated for many metal nanoparticle investigations of many bacterial strains, such as Bacillus sp., Pseudomonas sp., and Streptomyces sp. 8 . The intermediate stage of research on the biological generation of metallic nanoparticles is being dominated by bacteria due to their ability and tolerance for metal bioaccumulation. Organic acids, phenolic derivatives, polyphenols, dihydroxy benzene, The reduction may be explained by the existence of numerous aromatic rings, OH groups, and groups., bioformation, and capping of many nanoparticles. The goal of the current study was to determine the biocompatibility and cytotoxicity of ZnONPs, which could be used in many future biotechnological fields. The bacterial biosynthesis of ZnONPs was carried out using a local bacterial strain as a trial to overcome the hazards caused by the physical and chemical approaches.
Material and methods
Chemicals and reagents.
Zinc sulfate (ZnSO 4 · 7H 2 O) was purchased from Sigma, Aldrich, Germany, and was used for zinc solution preparation. Nutrient broth and agar medium were purchased from Oxoid, UK. 3-(4, 5-dimethyIthiazol-2-y1)-2-5-diphenyl tetrazolium bromide (MTT) was obtained from Merck KGaA (Darmstadt, Germany). 10% fetal bovine serum (FBS), 2 mM L-glutamine solution, 100 units/ml penicillin G sodium, 100 units/ml streptomycin sulfate, and 250 g/ml amphotericin B were obtained from Lonza, Basel, Switzerland. All chemicals were analytical grades.
Bacteria strain
Priestia megaterium BASMA 2022 (OP572246) strain was collected from the Microbiology Department, Faculty of Agriculture, Ain Shams University. The strain was maintained and subcultured periodically in a nutrient broth medium and preserved at 4 °C.
Culture filtrate preparation
The culture filtrate was prepared by inoculating a loop of freshly prepared microbial culture into the sterilized nutrient broth medium 9 in a 100-ml flask. The inoculated flask was then incubated for 24 h at 30 °C in an orbital shaker incubator (Shin Saeng; South Korea) at 150rpm, and O.D. was observed at 620 nm 10 . One ml of the inoculum contained 2.55 × 10 6 CFU/ml.
Biosynthesis of the ZnONPs using P. megaterium (Pm/ZnONPs)
The P. megaterium zinc oxide nanoparticles were synthesized following the procedure described by Kasana et al 11 . After 12 h of inoculum incubation, 50 ml of sterilized 1 mM ZnSO 4 · 7H 2 O solution was added under aseptic conditions, then re-incubated (150 rpm) at 30 °C for 24 h. The biosynthesis of Pm/ZnONPs was indicated by changing the color of the reaction mixture from pale yellow to pale brown. After that, the promising nanoparticle-supernatant mixture solution was centrifuged using the SIGMA 2–16 P, USA centrifuge at 10,000rpm for 10 min. The bacterial pellets were discarded, whereas the cell-free supernatant was collected separately to investigate the extracellular biosynthesized Pm/ZnONPs.
P. megaterium zinc oxide nanoparticle characterization
The preliminary investigation for Pm/ZnONPs formation was detected by a color change of the P. megaterium- 1 mM ZnSO 4 · 7H 2 O reaction mixture from pale-yellow to dark. The investigation of Pm/ZnONPs biosynthesis was detected through UV–vis spectroscopy (UNICO UV-2100, China) at a wavelength of 400–700 nm 12 . HR-TEM (JEOL JEM-2100, Japan) was utilized to investigate the shape regulation and poly-dispersity of Pm/ZnONPs using an amorphous carbon-coated copper grid loaded with an aliquot of Pm/ZnONPs suspension, dried, and analyzed with a 20.0 kV accelerating voltage. The TEM images were captured with an image resolution of 512 by 442 and an image pixel size of 0.04 μm 12 , 13 . The polydispersity of the produced nanoparticles was expressed as PDI by estimating the average size of nanoparticles and the standard deviation of synthesized nanoparticles 13 . PDI was calculated as follows :
whereas PDI = polydispersity index; σ = standard deviation of nanoparticle size; and RAvg = nanoparticle average size.
The subsequent investigations, including concentration estimation, were carried out using an atomic absorption spectrophotometer (Perkin Elmer A Analyst 100; Canada), whereas Fourier transform infrared spectroscopy (FTIR) (Perkin Elmer, 400-FTIR; USA) was used in the wavenumber range 4000–400 cm −1 , with a resolution of 4 cm −1 and a refractive index of 2.4. FTIR was used for the identification of the functional groups in the P. megaterium supernatant responsible for reducing the ZnSO 4 · 7H 2 O, according to Izzi et al 8 . Elemental content and surface images of Pm/ZnONPs 8 , 12 , 13 were detected using SEM–EDX (Quanta FEG 250, FEI Company, Hillsboro, Oregon, USA) at The Desert Research Center in Egypt (EDRC), Cairo, Egypt.
Cytotoxicity activity assay of Pm/ZnONPs
The cytotoxicity of the biosynthesized Pm/ZnONPs on cell viability was assessed at Creative Egyptian Biotechnologists (CEB), Cairo, Egypt using an MTT assay. For calibration before the MTT assay, the suspension cells in the 96-well plate were spined at 2500rpm at 4 °C for 5 min in a microplate-compatible centrifuge (CAPPRondo Microplate Centrifuge, Germany) and carefully aspirated. Ensure that the same volume of existing media is present for each sample. Then add 50 µl of serum-free medium and 50 µl of MTT solution into each well 14 . Incubate the plate at 37 °C for 3 h. Human A375 skin melanoma and human bone marrow 2M-302 cell lines were routinely cultured in RPMI. Fetal bovine serum (FBS) at 10%, 2 mM L-glutamine, 100 units/ml penicillin G sodium, 100 units/ml streptomycin sulfate, and 250 g/ml amphotericin B are added as supplements. Cells were kept in humidified air with 5% CO 2 at 37 °C when sub-confluent. After trypsin/EDTA treatment at 37 °C, monolayer cells were collected for subculturing. When confluence had reached 75%, cells were utilized. Another aliquot of 100 μl of medium containing different doses of medicines was used to treat the cells. After 48 h of drug exposure, medium was discarded, and MTT solution (20 μl of 1 mg/ml stock solution) was added to 100 μl of phosphate buffer solution (PBS) in each well and incubated at 37 °C for 4 h. Then the formed formazan crystals were dissolved in 100 μl of absolute DMSO. The absorbance of formazan solutions was measured at λmax 570 nm using an ELISA plate reader (FLUOstar OPTIMA, BMG LABTECH GmbH, Ortenberg, Germany).
Statistical analysis
Cytotoxicity and IC 50 data are reported as mean ± SD (n = 3) using Graph Pad Prism 8.4.1 (GraphPad Software, San Diego, CA, www.graphpad.com ), and the interaction was found to be significant at P < 0.05.
Ethical approval
This article does not contain any studies with human participants or animals performed by any of the authors.
Biosynthesis of Pm/ZnONPs
The biosynthesis of Pm/ZnONPs was detected gradually by the change in color from a pale yellow color (before the reduction of Zn) to a pale brownish solution (after the reduction of Zn), indicating the formation of ZnO nanoparticles.
Characterization of the biosynthesized Pm/ZnONPs
Uv–vis spectroscopic analysis of pm/znonps.
UV–Vis spectroscopy (Fig. 1 ) of Pm/ZnONPs indicated a high absorption spectrum between 200 and 800 nm, with maximum absorption at 280 nm and a surface plasmon resonance (SPR) peak of 3.7.
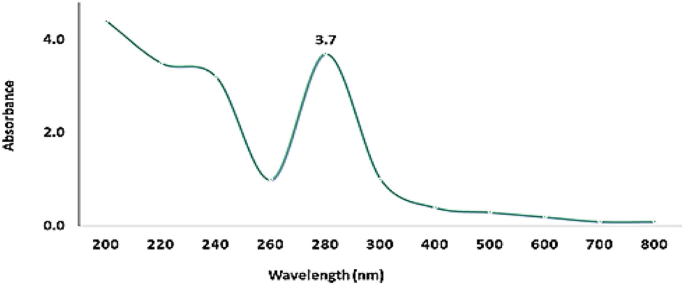
UV–Vis spectrum of green synthesized Pm/ZnONPs.
High-resolution transmission electron microscope (HR-TEM) analysis of the biosynthesized Pm/ZnONPs
The morphology and size of the biosynthesized Pm/ZnONPs were observed by HR-TEM (Fig. 2 ). The particles were semi-spheres and well-despised, with a size range of 5.77–13.9 nm. The polydispersed nature of particles was confirmed with a PDI value of 0.15.
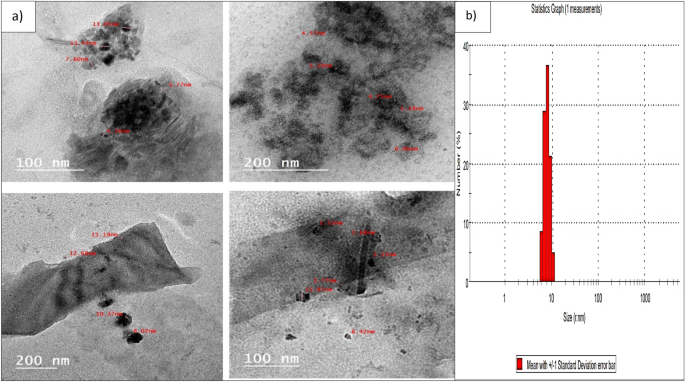
High-resolution transmission electron microscopy (HR-TEM) image of the biosynthesized Pm/ZnONPs, ( a ) Morphology and size of Pm/ZnONPs, ( b ) Mean standard division of Pm/ZnONPs.
Energy-dispersive X-ray spectroscopy (EDX) analysis of the biosynthesized Pm/ZnONPs
Energy-dispersive X-ray (EDX) investigation of Pm/ZnONPs confirmed the presence of the shield matrix, which consisted of carbon, nitrogen, oxygen, chlorine, potassium, sodium, aluminum, sulfur, and zinc, attached to Pm/ZnONPs with percentages of 40.2, 15.5, 26.3, 5.7, 0.6, 1.4, 7.3, 1.2, and 1.8%, respectively. This indicates active biomolecule formation that coated the ZnONPs (Fig. 3 ).
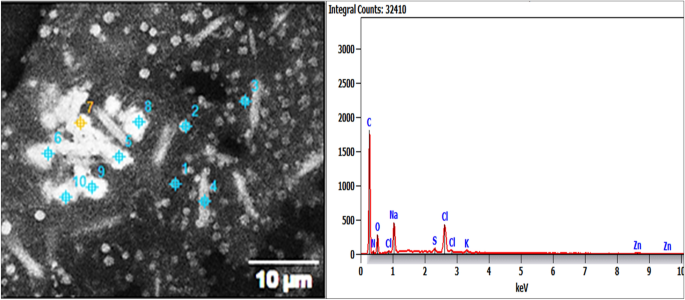
Energy-dispersive X-ray (EDX) spectroscopy of the biosynthesized Pm/ZnONPs.
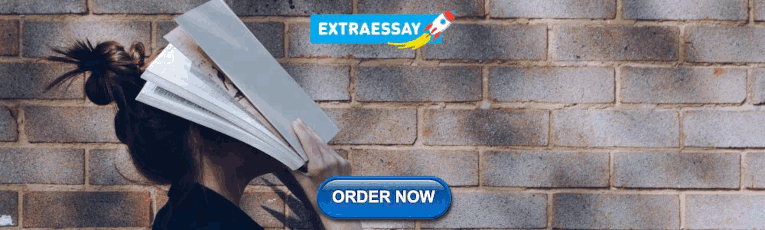
Fourier transformation infrared (FTIR) characterization of the biosynthesized Pm/ZnONPs
The Fourier transformation infrared (FTIR) characterization analysis of the biosynthesized Pm/ZnONPs is illustrated in Fig. 4 . The infrared spectrum shows five mean independent peaks at 3742.24, 3257.69, 2355.67, 2177.12, and 1640.02 cm − 1 . This spectrum clarifies the presence of O–H in alcohols and phenols, O=C=O stretching of carbon dioxide, N=C=S stretching of isothiocyanate, and N–H bending of amine.
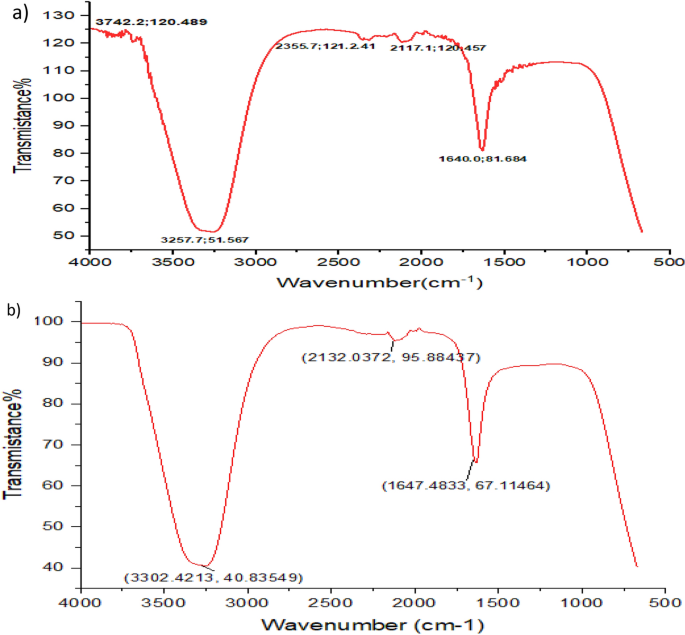
Fourier transmission infrared spectroscopy (FTIR) spectrum of, ( a ) the biosynthesized Pm/ZnONPs. ( b ) P. megaterium culture supernatant (control).
Zeta potential determination of the biosynthesized Pm/ZnONPs
As shown in Fig. 5 , Pm/ZnONPs have a zeta potential of − 16.2 mV, which can be attributed to the nonionic character of the capping molecules in the P. megaterium culture supernatant. This negative charge indicates the good stability of the biosynthesized ZnONPs.
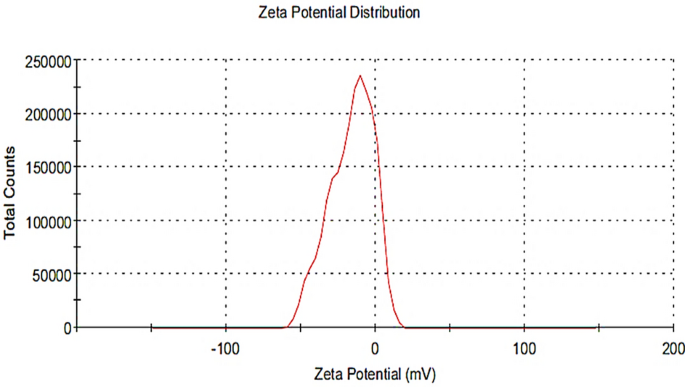
Zeta potential analysis of Pm/ZnONPs showed a -16.2 mV charge.
Assessment of the biosynthesized Pm/ZnONPs cytotoxicity
The MTT assay revealed different effects of each different sample on the cell lines. The Pm/ZnONPs sample was toxic to the skin A375 cell line with an IC 50 equal to 8.42% v/v. The cell viability (%) was 47.36, 116.76, 132.92, 134.01, 133.57, and 115.67% for Pm/ZnONPs concentrations of 10, 5, 2.5, 1.25, 0.625, and 0.312 μg/ml, respectively, with an IC 50 dose of > 6.1% Pm/ZnONPs as presented in Fig. 6 a. While the bone marrow cells showed increased proliferation up to 200% in a dose-dependent manner, The cell viability (%) was 208.76, 158.04, 148.07, 94.72, 95.18, and 100.12% for Pm/ZnONPs concentrations of 10, 5, 2.5, 1.25, 0.625, and 0.312 μg/ml, respectively, as presented in Fig. 6 a, b.
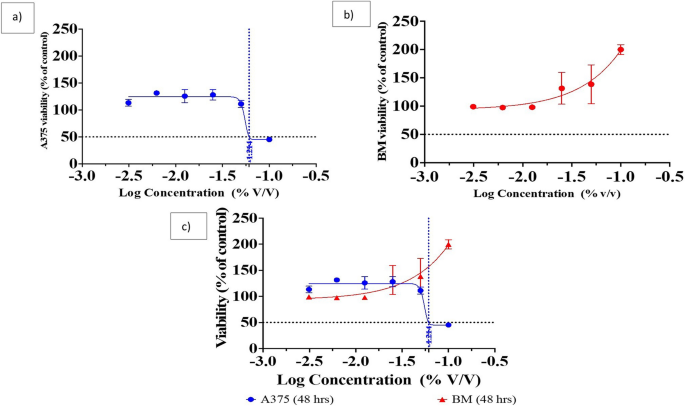
The dose curve of the various biosynthesized Pm/ZnONPs concentrations and IC 50 doses using the human A375 skin melanoma and human bone marrow 2M-302 cell lines. ( a ) Human A375 skin melanoma; ( b ) Bone marrow 2M-302 cell lines; and ( c ) Human A375 skin melanoma versus Bone marrow 2M-302 cell line.
As known, ZnONPs have been listed as “Generally Recognized as Safe” (GRAS) by the US Food and Drug Administration (FDA 21CFR182.8991) due to their non-toxic properties 15 , 16 . In the present investigation, bio-augmented zinc oxide nanoparticles (ZnONPs) were prepared from P. megaterium BASMA 2022 (OP572246) bacteria extracellular supernatant 17 . Biological synthesis using microbes offers an advantage over plants since microbes are easily reproduced. The synthesis of metal and metal oxide NPs depends on the ability of microbes to tolerate heavy metals. Moreover, it is well known that high metal stress may affect various microbial activities 18 , 19 , 20 . Under stressful environments, the microbes tend to reduce metals to ions. As such, this demonstrates their capability to act as a natural nano factory 21 , 22 . Generally, microbes that inhabit rich metals exhibit metal resistance due to chelation by extracellular proteins 17 . Many bacteria were reported for extracellular biosynthesis of ZnONPs, such as Bacillus licheniformis MTCC9555, Bacillus megaterium (NCIM2326), Lactobacillus paracasei LB3, and Lactobacillus sporogenes 22 , 23 , 24 , 25 .
The biological synthesis of metal and its oxide NPs requires metal precursors, which are usually supplied in the form of soluble salts and precipitated in the suspension containing microbial cells and/or biological compound extracts from the microbe’s culture growth. The synthesis reaction is usually completed within minutes or hours, depending on the culture conditions, which results in white deposition in the bottom flasks or changes in the color of suspensions 16 , 21 . This observation in color change was confirmed by many previous studies 4 , 5 , 20 , 26 , 27 .
The room-temperature UV–Vis absorbance spectrum was identified as the surface plasmon resonance (SPR) that is a characteristic of metal nanoparticles and their oxides 23 , 24 . The confirmation investigation of Pm/ZnONPs formation was using UV–Vis spectroscopy at an absorption spectrum between 200 and 800 nm with maximum absorption at 280 nm and SPR peak of 3.7. Biosynthesis of Pm/ZnONPs was detected gradually by the change in color from a pale yellow color to a brownish solution, indicating the formation of ZnO nanoparticles due to the excitation of nanoparticles’ surface plasmon resonance (SPR). On the other hand, it was reported that the UV–vis spectra results indicated a strong and broad peak at 250 and 374 nm, implying the successful formation of ZnONPs 5 , 17 , 28 .
HR-TEM investigation of the biosynthesized Pm/ZnONPs indicated the average diameter of the biosynthesized Pm/ZnONPs was 5.77–13.9 nm of semi-spheres and well-despised nanoparticles. While in other reports it was reported to be lower than in the findings of Selvarajan and Mohanasrinivasan 29 demonstrated a spherical shape ZNO–NP synthesis using Lactobacillus plantarum VITES07 with size ranging from 7 to 19 nm and B. licheniformis MTCC9555 with size at 250 nm 23 . In the study of Król et al 24 , ZnONPs mediated L. paracasei LB3 with a larger size of 1179 nm. The spherical and semi-sphere shapes of ZnONPs were observed in many reports 1 , 4 , 27 , 30 , 31 .
The EDX investigation of Pm/ZnONPs confirmed the presence of a strong shield matrix coat that consisted of C, N, O, Cl, K, and Na that attached to ZnONPs with 40.2, 15.5, 26.3, 5.7, 0.6, 1.4, 7.3, 1.2, and 1.8%, respectively. This indicates active biomolecule formation that coated the ZnONPs. These elements indicate that the presence of various enzymes, proteins, and other biomolecules from P. megaterium cell-free supernatant plays a vital role in the reduction process of Zn metal solution. These multiple organic components secreted in the suspension or growth medium are attributed to the formation of multiple sizes and shapes of mono- and polydispersed NPs 31 , 32 . The capping effect of the accumulated active compounds on the metal nanoparticle core is responsible for the reduction, stability, and capping of the nanoparticles, as described by Mohamed 31 .
The FTIR characterization analysis of the biosynthesized Pm/ZnONPs showed mean five independent peaks at (3742.24 and 3257.69), 2355.67, 2177.12, and 1640.02 cm −1 . This spectrum clarifies the presence of O–H in alcohols and phenols, O=C=O stretching of carbon dioxide, N=C=S stretching of isothiocyanate, and N–H bending of amine. Velmurugan et al 33 . findings revealed the presence of protein and amide, with one and two peaks at 1100, 1400, 1650, 2900, and 3000 cm −1 , respectively. Nevertheless, there was no protein signal detected in the zinc crystal produced by the dead biomass of Fusarium spp.. Similarly 34 , evaluated the chemical composition of the ligands capping the NPs. The FTIR results demonstrated two absorption bands at 1650 and 1566 cm −1 , which indicated the typical amide absorptions of protein molecules. These findings were in line with the findings obtained by 35 , as biomolecules were identified as the molecules that had the ability for biosynthesized NP capping and stabilization. It was found that the N–H peak appeared at 1640.02 cm −1 and covered amine groups and nitro compound bonds that identify the bounds of protein groups responsible for biosynthesis and between the biosynthesized nanoparticles as stabilizing caps attached to proteins and amino acid residues. Numerous investigations have also suggested that nitrate reductase is involved in extracellular production, which results in the reduction of metal ions into metal NPs 24 , 36 , 37 , 38 .
The extracellular synthesis route entails either enzyme-mediated synthesis occurring on the cell membrane or the release of the enzyme as an extracellular enzyme into the growth medium. Nitrate reductase is an enzyme in the nitrogen cycle that catalyzes the conversion of nitrate to nitrite. For instance, NADH-dependent reductase, which serves as an electron carrier, transferred an electron from NADH to begin the bioreduction of Zn 2+ 24 , 39 . As a result, Zn 2+ attracted electrons and transformed them into Zn 0 and ZnONPs were then created as a result of this 35 . The Pm/ZnONPs have a zeta potential of − 16.2 mV, which can be attributed to the nonionic character of the capping molecules in the P. megaterium culture supernatant. And indicated that the nanoparticles synthesized were highly stable. It scored a zeta potential value of − 33.4 mV with Serratia nematodiphila 40 and Pseudomonas aeruginosa 41 with − 18.0 mV.
As reported by 17 , 42 despite ZnONPs potential use as a feed supplement, it also tends to cause adverse effects on animals and human cells. However, the toxicological hazards of ZnONPs remain controversial because, while a few studies have reported ZnONPs to have therapeutic benefits, other studies have reported their toxicity to living organisms. The MTT assay of cytotoxicity assessment revealed various effects on the human A375 skin melanoma cell line, with an IC 50 of 8.42% for Pm/ZnONPs. While the human bone marrow 2M-302 cell line showed increased proliferation up to 200% in a dose-dependent manner, studies have suggested that the toxicity effects of ZnONPs are dependent on their dose (concentration) 42 , morphology and composition 43 and size 44 . As reported, the smaller size of NPs ranging between 3 and 6 nm is more easily cleared out of the kidneys compared to bigger NPs with a size near 30 nm, which remain and accumulate in the liver. In addition 45 , 46 , 47 reported that larger NPs also tend to stay longer in the kidneys and skin due to the slower excretion mechanisms of glomerular filtration, and this long-term retention can lead to organ toxicity. In addition, different morphologies of NPs also contribute to the toxicity effects, regardless of their specific surface area. investigated the cytotoxicity effects of ZnONPs with different morphologies, such as nonuplets, nanorods, nanosheets, and nanoflowers, on malignant human T98G gliomas and fibroblast cells 48 . Nanorods demonstrated higher cytotoxicity and inhibitory effects on normal and tumor cells due to a larger effective surface area that potentially induces higher oxidative stress on cells.
Due to the circumstances of the chemical reaction in the usual approach, it was also noted that the chemically and physically manufactured ZnONPs might be one of the potential sources of the inherent toxicity of NPs 47 . It has been hypothesized that ZnONPs’ harmful effects result from their ability to readily penetrate cells, attach to membranes, or release Zn 2+ , which causes oxidative stress-mediated DNA damage and lipid peroxidation, all of which lead to apoptosis. Several studies have reported that high doses of ZnONPs supplementation could lead to toxicity 47 , 49 , 50 , 51 , 52 . Oral administration of ZnONPs (20%) in lambs caused toxicity effects, which included increased levels of blood urea nitrogen (BUN) and creatinine, indicating renal dysfunction 51 . Also, results of an in vivo experiment conducted by Wang et al 53 ., showed that by reducing body weight and increasing the relative weight of the pancreas, brain, and lung in mice, high dosages of ZnO-NP supplementation at 50% resulted in toxicity. In addition, zinc buildup was seen in the bones, kidney, liver, and pancreas. Meanwhile, long-term exposure to ZnONPs at 5% only showed minimal toxicity. Furthermore, in the histopathological examination, a high concentration of oral administration of ZnONPs at 4% induced focal hemorrhages and necrosis on the liver and heart tissue of Wistar rats, which were caused by oxidative stress 53 , 54 . Also, it was discovered that the surface-bound active compounds on the surface of NPs play a crucial role in their biological interactions.
As reported previously, coatings for the surface of ZnONPs were effective in reducing their cytotoxicity effect on epithelial cells by restricting the dissociation of ZnONPs to Zn 2+ 55 , 56 . On the other hand, the current findings demonstrate that Pm/ZnONPs stimulate the synthesis of bone marrow cells and may be used to treat bone marrow production deficiencies. Deylam et al 57 . reported that ZnONPs with average sizes of 10–30 and 35–45 nm on bone marrow and mesenchymal stem cells (MSCs) were found to be safe at concentrations of 5 and 10 µg/ml. As the cell-cycle analysis indicated, they upregulate the aging-related genes NF–kB and p53 and downregulate the anti-aging gene Nanog. Wang et al 58 , discovered that zinc-whitlockite ((ZnWH)/G/H) nanoparticles exhibited interconnected pore structures, outstanding mechanical characteristics, and tunable swelling ratios. The high quantities of alkaline phosphatase (ALP), osteocalcin (OCN), and osteopontin that are released by human bone marrow mesenchymal stem cells (hBMSCs) can induce osteogenic development in addition to their good biocompatibility (OPN). The ZnWH scaffold dramatically sped up the process of bone restoration after 12 weeks of therapy in the rabbit femoral defect model, making it a viable choice for bone regeneration. In summary, the toxic effects of ZnONPs are caused by their dosage, size, and shape; thus, the use of ZnONPs in many applications should be restricted to a specific minimum concentration to avoid their toxic effects. Moreover, for improved safety of Pm/ZnONPs, microbe-mediated synthesis should be considered in NP production due to its biocompatibility as well as controllable NPs size and shape, which can be achieved through the optimization process.
The study aimed to develop a simple, eco-friendly, and non-toxic method for bacterial green synthesis of zinc oxide nanoparticles using P. megaterium BASMA 2022 (OP572246). The resulting Pm/ZnONPs were characterized using various techniques, including UV–Vis spectroscopy, HR-TEM, EDX, FTIR, and zeta potential. The Pm/ZnONPs had a semi-sphere shape and good stability, with IC 50 values of 8.42 and 200% for human A375 skin melanoma and human bone marrow 2M-302 cell lines, respectively. These findings could help in future applications in various sectors such as agriculture, nutrition, pharmaceuticals, and biomedical research.
Data availability
The datasets generated during the current study are available in the [NCBI] repository, https://www.ncbi.nlm.nih.gov/nuccore/OP572246
Rudrappa, M. et al. Plumeria albaMediated green synthesis of silver nanoparticles exhibits antimicrobial effect and anti-oncogenic activity against glioblastoma U118 MG cancer cell line. Nanomaterials 12 (3), 493. https://doi.org/10.3390/nano12030493 (2021).
Article CAS Google Scholar
Happy, A., Venkat, K. S. & Rajesh, K. S. A review of the eco-friendly approach to the green synthesis of zinc oxide nanoparticles. Resour. Effic. Technol. 3 , 406–413. https://doi.org/10.1016/j.reffit.2017.03.002 (2017).
Article Google Scholar
Barabadi, H. et al. Penicillium family as an emerging nanofactory for the biosynthesis of green nanomaterials: a journey into the world of microorganisms. J. Clust. Sci. https://doi.org/10.1007/s10876-019-01554-3 (2019).
Rajivgandhi, G. et al. Biosynthesized zinc oxide nanoparticles (ZnO NPs) using actinomycetes enhance the anti-bacterial efficacy against K. pneumoniae. J. King Saud Univ. Sci. 34 (1), 101731. https://doi.org/10.1016/j.jksus.2021.101731 (2021).
Daraei, H. et al. Biotoxicity evaluation of zinc oxide nanoparticles on the bacterial performance of activated sludge at COD, nitrogen, and phosphorus reduction. Front. Environ. Sci. Eng. 16 , 19. https://doi.org/10.1007/s11783-021-1453-z (2022).
Faisal, S. et al. Green synthesis of zinc oxide (ZnO) nanoparticles using aqueous fruit extracts of Myristica fragrans : Their characterizations and biological and environmental applications. ACS Omega 6 (14), 9709–9722. https://doi.org/10.1021/acsomega.1c00310 (2021).
Article PubMed PubMed Central CAS Google Scholar
Tilahun, E., Adimasu, Y. & Dessie, Y. Biosynthesis and optimization of ZnO nanoparticles using Ocimum lamifolium leaf extract for electrochemical sensor and antibacterial activity. ACS Omega 8 (30), 27344–27354. https://doi.org/10.1021/acsomega.3c02709 (2023).
Izzi, M., Sportelli, M. C., Torsi, L., Picca, R. A. & Cioffi, N. Synthesis and antimicrobial applications of ZnO nanostructures: A review. ACS Appl. Nano Mater. 6 (13), 10881–10902. https://doi.org/10.1021/acsanm.3c01432 (2023).
Difco Manual Dehydrated culture media and reagents for microbiology. 10th Ed. Difco Laboratories, Detroit, M. (ed.), (1984). p. 1027.
Ammoneh, H., Harba, M., Akeed, Y., Al-Halabi, M. & Bakri, Y. Isolation and identification of local Bacillus isolates for xylanase biosynthesis. Iran. J. Microbiol. 6 (2), 127–132 (2014).
PubMed PubMed Central Google Scholar
Kasana, R. C., Panwar, N. R. & Kaul, R. K. Biosynthesis and effects of copper nanoparticles on plants. Environ. Chem. Lett. 15 , 233–240. https://doi.org/10.1007/s10311-017-0615-5 (2017).
Abd-Elhalim, B. T., Gamal, R. F., Abou-Taleb, K. A. & Haroun, A. A. Biosynthesis of copper nanoparticles using bacterial supernatant optimized with certain agro-industrial byproducts. Novel Res. Microbiol. J. 6 , 558–578 (2019).
Google Scholar
Chakraborty, B. et al. Biosynthesis and characterization of polysaccharide-capped silver nanoparticles from Acalypha indica L. and evaluation of their biological activities. Environ. Res. 225 , 115614. https://doi.org/10.1016/j.envres.2023.115614 (2023).
Article PubMed CAS Google Scholar
Abudoleh, S. M., Abdallah Abualassal, Q. I., Abudayeh, Z., Aldalahmah, Y. & Hussein, M. Z. Preparation and characterization of ciprofloxacin-loaded silver nanoparticles for drug delivery. IET Nanobiotechnol. 16 (3), 92–101. https://doi.org/10.1049/nbt2.12081 (2022).
Article PubMed PubMed Central Google Scholar
Wang, C. et al. Zinc oxide nanoparticles as a substitute for zinc oxide or colistin sulfate: Effects on growth, serum enzymes, zinc deposition, intestinal morphology, and epithelial barrier in weaned piglets. PLoS One https://doi.org/10.1371/journal.pone.0181136 (2017).
FDA (Food and Drug Administration), Washington, DC, USA, 2015. Select Committee on GRAS Substances (SCOGS) Opinion: Zinc Salts 2015. https://www.accessdata.fda.gov/scripts/cdrh/cfdocs/cfcfr/CFRSearch.cfm?fr=182.8991 . Accessed Nov. 5, 2018.
Mohd Yusof, H. et al. Microbial synthesis of zinc oxide nanoparticles and their potential application as an antimicrobial agent and a feed supplement in the animal industry: A review. J. Anim. Sci. Biotechnol. 10 , 57. https://doi.org/10.1186/s40104-019-0368-z (2019).
Giller, K. E., Witter, E. & McGrath, S. P. Heavy metals and soil microbes. Soil Biol. Biochem. 41 , 2031–2037. https://doi.org/10.1016/j.soilbio.2009.04.026 (2009).
Sarkar, J., Ghosh, M., Mukherjee, A., Chattopadhyay, D. & Acharya, K. Biosynthesis and safety evaluation of ZnO nanoparticles. Bioprocess. Biosyst. Eng. 37 , 165–171. https://doi.org/10.1007/s00449-013-0982-7 (2014).
Velusamy, P., Kumar, G. V., Jeyanthi, V., Das, J. & Pachaiappan, R. Bio-inspired green nanoparticles: Synthesis, mechanism, and antibacterial application. Toxicol. Res. 32 , 95–102. https://doi.org/10.5487/TR.2016.32.2.095 (2016).
Salvadori, M. R., Ando, R. A., Do Nascimento, O. C. A. & Corrêa, B. Intracellular biosynthesis and removal of copper nanoparticles by dead biomass of yeast isolated from the wastewater of a mine in the Brazilian Amazonia. PLoS One https://doi.org/10.1371/journal.pone.0087968 (2014).
Saravanan, M. et al. Green synthesis of anisotropic zinc oxide nanoparticles with antibacterial and cytofriendly properties. Microb. Pathog. 115 , 57–63. https://doi.org/10.1016/j.micpath.2017.12.039 (2018).
Tripathi, R. M. et al. ZnO nanoflowers: Novel biogenic synthesis and enhanced photocatalytic activity. J. Photochem. Photobiol. B Biol. 141 , 288–295. https://doi.org/10.1016/j.jphotobiol.2014.10.001 (2014).
Król, A., Railean-Plugaru, V., Pomastowski, P., Złoch, M. & Buszewski, B. Mechanism study of intracellular zinc oxide nanocomposites formation. Colloids Surf. A Physicochem. Eng. Asp. 553 , 349–358. https://doi.org/10.1016/j.colsurfa.2018.05.069 (2018).
Prasad, K. & Jha, A. K. ZnO nanoparticles: Synthesis and adsorption study. Nat. Sci. 01 , 129–135. https://doi.org/10.4236/ns.2009.12016 (2009).
Chauhan, R., Reddy, A. & Abraham, J. Biosynthesis of silver and zinc oxide nanoparticles using Pichia fermentans JA2 and their antimicrobial properties. Appl. Nanosci. 5 , 63–71. https://doi.org/10.1007/s13204-014-0292-7 (2015).
Article ADS CAS Google Scholar
Moghaddam, A. B. et al. Biosynthesis of ZnO nanoparticles by a new Pichia kudriavzevii yeast strain and evaluation of their antimicrobial and antioxidant activities. Molecules 22 , 1–18. https://doi.org/10.3390/molecules22060872 (2017).
Markus, J. et al. Intracellular synthesis of gold nanoparticles with antioxidant activity by probiotic Lactobacillus kimchicus DCY51T isolated from Korean kimchi. Enzym. Microb. Technol. 95 , 85–93. https://doi.org/10.1016/j.enzmictec.2016.08.018 (2016).
Selvarajan, E. & Mohanasrinivasan, V. Biosynthesis and characterization of ZnO nanoparticles using Lactobacillus plantarum VITES07. Mater. Lett. 112 , 180–182. https://doi.org/10.1016/j.matlet.2013.09.020 (2013).
Taran, M., Rad, M. & Alavi, M. Biosynthesis of TiO2 and ZnO nanoparticles by Halomonas elongata IBRC-M 10214 in different conditions of medium. BioImpacts. 8 , 81–89 (2018).
Mohamed, E. A. Green synthesis of copper and copper oxide nanoparticles using the extract of seedless dates. Heliyon 6 , e03123. https://doi.org/10.1016/j.heliyon.2019.e03123 (2020).
Kitching, M., Ramani, M. & Marsili, E. Fungal biosynthesis of gold nanoparticles: Mechanism and scale-up. Microb. Biotechnol. 8 , 904–917. https://doi.org/10.1111/1751-7915.12151 (2015).
Velmurugan, P. et al. Removal of zinc by live, dead, and dried biomass of Fusarium spp. isolated from the abandoned metal mine in South Korea and its perspective on producing nanocrystals. J. Hazard. Mater. 182 , 317–324. https://doi.org/10.1016/j.jhazmat.2010.06.032 (2010).
Bao, H., Hao, N., Yang, Y. & Zhao, D. Biosynthesis of biocompatible cadmium telluride quantum dots using yeast cells. Nano Res. 3 , 481–489. https://doi.org/10.1007/s12274-010-0008-6 (2010).
Mendes, C. R. et al. Antibacterial action and target mechanisms of zinc oxide nanoparticles against bacterial pathogens. Sci. Rep. 12 (1), 1–10. https://doi.org/10.1038/s41598-022-06657-y (2022).
Anil Kumar, S. et al. Nitrate reductase-mediated synthesis of silver nanoparticles from AgNO3. Biotechnol. Lett. 29 , 439–445. https://doi.org/10.1007/s10529-006-9256-7 (2007).
Kalimuthu, K., Suresh Babu, R., Venkataraman, D., Bilal, M. & Gurunathan, S. Biosynthesis of silver nanocrystals by Bacillus licheniformis . Colloids Surf. B Biointerfaces. 65 , 150–153. https://doi.org/10.1016/j.colsurfb.2008.02.018 (2008).
Kundu, D., Hazra, C., Chatterjee, A., Chaudhari, A. & Mishra, S. Extracellular biosynthesis of zinc oxide nanoparticles using Rhodococcus pyridinivorans NT2: Multifunctional textile finishing, biosafety evaluation, and in vitro drug delivery in colon carcinoma. J. Photochem. Photobiol. B Biol. 140 , 194–204. https://doi.org/10.1016/j.jphotobiol.2014.08.001 (2014).
Hulkoti, N. I. & Taranath, T. C. Biosynthesis of nanoparticles using microbes: A review. Colloids Surf. B Biointerfaces. 121 , 474–483. https://doi.org/10.1016/j.colsurfb.2014.05.027 (2014).
Jain, D. et al. Microbial fabrication of zinc oxide nanoparticles and evaluation of their antimicrobial and photocatalytic properties. Front. Chem. 8 , 559994. https://doi.org/10.3389/fchem.2020.00778 (2020).
Singh, B. N., Rawat, A. K., Khan, W., Naqvi, A. H. & Singh, B. R. Biosynthesis of stable antioxidant ZnO nanoparticles by Pseudomonas aeruginosa rhamnolipids. PLoS ONE 9 , e106937. https://doi.org/10.1371/journal.pone.0106937 (2014).
Article ADS PubMed PubMed Central CAS Google Scholar
Deng, X. Y. et al. Nanosized zinc oxide particles induce neural stem cell apoptosis. Nanotechnology https://doi.org/10.1088/0957-4484/20/11/115101 (2009).
Article PubMed Google Scholar
Pujalte, I. et al. Cytotoxicity and oxidative stress induced by different metallic nanoparticles on human kidney cells. Part Fibre Toxicol. 8 , 10. https://doi.org/10.1186/1743-8977-8-10 (2011).
Guo, D. et al. Synergistic cytotoxic effect of different-sized ZnO nanoparticles and daunorubicin against leukemia cancer cells under UV irradiation. J. Photochem. Photobiol. B Biol. 93 , 119–126. https://doi.org/10.1016/j.jphotobiol.2008.07.009 (2008).
Burns, A. A. et al. Fluorescent silica nanoparticles with efficient urinary excretion are ideal for nanomedicine. Nano Lett. 9 , 442–448. https://doi.org/10.1021/nl803405h (2009).
Kumar, R. et al. In vivo biodistribution and clearance studies using multimodal organically modified silica nanoparticles. ACS Nano. 4 , 699–708. https://doi.org/10.1016/j.jconrel.2012.05.046 (2010).
Rajput, V. D. et al. Effects of zinc-oxide nanoparticles on soil, plants, animals, and soil organisms: A review. Environ. Nanotechnol. Monit. Manag. 9 , 76–84. https://doi.org/10.3390/nano11071722 (2018).
Wahab, R. et al. Self-styled ZnO nanostructures promote cancer cell damage and suppress the epithelial phenotype of glioblastoma. Sci. Rep. https://doi.org/10.1038/srep19950 (2016).
Swain, P. S., Rao, S. B. N., Rajendran, D., Dominic, G. & Selvaraju, S. Nanozinc, an alternative to conventional zinc as an animal feed supplement: A review. Anim. Nutr. 2 , 134–141. https://doi.org/10.1016/j.aninu.2016.06.003 (2016).
Król, A., Pomastowski, P., Rafińska, K., Railean-Plugaru, V. & Buszewski, B. Zinc oxide nanoparticles: Synthesis, antiseptic activity, and toxicity mechanism. Adv. Colloid Interface Sci. 249 , 37–52. https://doi.org/10.1016/j.cis.2017.07.033 (2017).
Najafzadeh, H. et al. Serum biochemical and histopathological changes in liver and kidney in lambs after zinc oxide nanoparticle administration. Vet World. https://doi.org/10.5455/vetworld.2013.534-537 (2013).
Tang, H. Q. et al. The effect of ZnO nanoparticles on liver function in rats. Int. J. Nanomed. https://doi.org/10.2147/IJN.S109031 (2016).
Wang, C. et al. Effects of long-term exposure to zinc oxide nanoparticles on development, zinc metabolism, and biodistribution of minerals (Zn, Fe, Cu, and Mn) in mice. PLoS One https://doi.org/10.1371/journal.pone.0164434 (2016).
Saman, S., Moradhaseli, S., Shokouhian, A. & Ghorbani, M. Histopathological effects of ZnO nanoparticles on liver and heart tissues in Wistar rats. Adv. Biores. 4 (2), 83–88 (2013).
CAS Google Scholar
Luo, M. et al. Reducing ZnO nanoparticle cytotoxicity by surface modification. Nanoscale https://doi.org/10.1039/c4nr00458b (2014).
Chia, S. L. & Leong, D. T. Reducing ZnO nanoparticle toxicity through silica coating. Heliyon https://doi.org/10.1016/j.heliyon.2016.e00177 (2016).
Deylam, M., Alizadeh, E., Sarikhani, M., Hejazy, M. & Firouzamandi, M. Zinc oxide nanoparticles promote the aging process in a size-dependent manner. J. Mater. Sci. Mater. Med. https://doi.org/10.1007/s10856-021-06602-x (2021).
Wang, M. et al. A scaffold with zinc-whitlockite nanoparticles accelerates bone reconstruction by promoting bone differentiation and angiogenesis. Nano Res. 16 , 757–770. https://doi.org/10.1007/s12274-022-4644-4 (2023).
Download references
Acknowledgements
The authors would like to thank the Agricultural Microbiology Department, Faculty of Agriculture, Ain Shams University, for providing all lab facilities.
Open access funding provided by The Science, Technology & Innovation Funding Authority (STDF) in cooperation with The Egyptian Knowledge Bank (EKB).
Author information
Authors and affiliations.
Department of Agricultural Microbiology, Faculty of Agriculture, Ain Shams University, Shubra El-Khaimah, Cairo, 11241, Egypt
Mona A. Ashour & Basma T. Abd-Elhalim
You can also search for this author in PubMed Google Scholar
Contributions
The research was conceived of and planned by B.A. and M.A. B.A. and M.A. performed tests and gathered information. All data were processed and interpreted by B.A. and M.A., and the article was edited by B.A. and M.A. The manuscript & draft was written by all contributors. The manuscript was read and modified by B.A. and M.A. The article was reviewed and approved by all authors.
Corresponding author
Correspondence to Basma T. Abd-Elhalim .
Ethics declarations
Competing interests.
The authors declare no competing interests.
Additional information
Publisher's note.
Springer Nature remains neutral with regard to jurisdictional claims in published maps and institutional affiliations.
Rights and permissions
Open Access This article is licensed under a Creative Commons Attribution 4.0 International License, which permits use, sharing, adaptation, distribution and reproduction in any medium or format, as long as you give appropriate credit to the original author(s) and the source, provide a link to the Creative Commons licence, and indicate if changes were made. The images or other third party material in this article are included in the article's Creative Commons licence, unless indicated otherwise in a credit line to the material. If material is not included in the article's Creative Commons licence and your intended use is not permitted by statutory regulation or exceeds the permitted use, you will need to obtain permission directly from the copyright holder. To view a copy of this licence, visit http://creativecommons.org/licenses/by/4.0/ .
Reprints and permissions
About this article
Cite this article.
Ashour, M.A., Abd-Elhalim, B.T. Biosynthesis and biocompatibility evaluation of zinc oxide nanoparticles prepared using Priestia megaterium bacteria. Sci Rep 14 , 4147 (2024). https://doi.org/10.1038/s41598-024-54460-8
Download citation
Received : 27 October 2023
Accepted : 13 February 2024
Published : 20 February 2024
DOI : https://doi.org/10.1038/s41598-024-54460-8
Share this article
Anyone you share the following link with will be able to read this content:
Sorry, a shareable link is not currently available for this article.
Provided by the Springer Nature SharedIt content-sharing initiative
- Cytotoxicity
- Half-maximal inhibitory concentration (IC 50 )
- Human bone marrow cell line
- Human skin melanoma
- Priestia megaterium
- Zinc oxide nanoparticles
By submitting a comment you agree to abide by our Terms and Community Guidelines . If you find something abusive or that does not comply with our terms or guidelines please flag it as inappropriate.
Quick links
- Explore articles by subject
- Guide to authors
- Editorial policies
Sign up for the Nature Briefing: Translational Research newsletter — top stories in biotechnology, drug discovery and pharma.


Antimicrobial Nanocomposite Coatings for Rapid Intervention of Catheter-Associated Urinary Tract Infections
Catheter-associated urinary tract infections (CAUTIs) pose a significant challenge in hospital settings. Current solutions available in the market involve incorporating antimicrobials and antiseptics onto catheters. However, challenges such as the uncontrolled release leading to undesirable toxicity, as well as the prevalence of antimicrobial resistance reduce the effectivity of these solutions. Additionally, conventional antibiotics fail to effectively eradicate entrenched bacteria metabolically suppressed bacteria present in the biofilm, necessitating the exploration of alternative strategies. Here, we introduce a novel polymer-nanocomposite coating that imparts rapid antimicrobial and anti-biofilm properties to coated urinary catheters. We have coated onto silicone-based urinary catheters an organo-soluble antimicrobial polymer nanocomposite (APN), containing hydrophobic quaternized polyethyleneimine and zinc oxide nanoparticles, in a single step coating process. The coated surfaces exhibited rapid eradication of drug-resistant bacteria within 10-15 mins, including E. coli, K. pneumoniae, MRSA, S. epidermidis, as well as drug-resistant fungi C. albicans. APN coated catheters exhibited potent bactericidal activity against uropathogenic strains of E. coli, even when incubated in human urine. Furthermore, the stability of the coating and retention of antimicrobial activity was validated even after multiple washes. More importantly, this coating deterred biofilm formation onto the catheter surface, and displayed rapid inactivation of metabolically repressed stationary phase and persister cells. The ability of coated surfaces to disrupt bacterial membranes and induce the generation of intracellular reactive oxygen species (ROS) was assessed through different techniques, such as electron microscopy imaging, flow cytometry as well as fluorescence spectroscopy and microscopy. The surface coatings were found to be biocompatible in an in-vivo mice model. Our simple one-step coating approach for catheters holds significant potential owing to its ability to tackle multidrug resistant bacteria and fungi, and the challenge of biofilm formation. This work brings us one step closer to enhancing patient care and safety in hospitals.
- This article is part of the themed collection: Celebrating the 65th birthday of Professor Santanu Bhattacharya
Supplementary files
- Supplementary information PDF (758K)
Article information
Download citation, permissions.

D. Patra, S. Ghosh, S. Mukherjee, Y. Acharya, R. Mukherjee and J. Haldar, Nanoscale , 2024, Accepted Manuscript , DOI: 10.1039/D4NR00653D
To request permission to reproduce material from this article, please go to the Copyright Clearance Center request page .
If you are an author contributing to an RSC publication, you do not need to request permission provided correct acknowledgement is given.
If you are the author of this article, you do not need to request permission to reproduce figures and diagrams provided correct acknowledgement is given. If you want to reproduce the whole article in a third-party publication (excluding your thesis/dissertation for which permission is not required) please go to the Copyright Clearance Center request page .
Read more about how to correctly acknowledge RSC content .
Social activity
Search articles by author.
This article has not yet been cited.
Advertisements
Degradation of toxic methylene blue dye with Zinc oxide (ZnO) nanoparticles synthesized by leaf extract of Madagascar periwinkle plant
- Original Article
- Published: 06 May 2024
Cite this article
- Akansha Gupta 1 ,
- Sapna Yadav 2 ,
- Nutan Rani 2 ,
- Kalpna Gupta 3 &
- Kalawati Saini ORCID: orcid.org/0000-0002-0317-1935 2
16 Accesses
Explore all metrics
In this paper, we have synthesized ZnO nanoparticles namely ZnO (L5) and ZnO (L10) nanoparticles. Both ZnO (L5) and ZnO (L10) nanoparticles have been synthesized by biosynthesis methods using Madagascar periwinkle leaf extract. The biosynthesis method is simple, eco-friendly, and cheap. ZnO (L5) and ZnO (10) nanoparticles have been characterized by using X-ray diffraction (XRD), Scanning Electron Microscopy (SEM), Energy Dispersive Spectroscopy (EDS), UV- Visible Spectroscopy, Fourier Transform Infrared Spectroscopy (FTIR), Field Emission Scanning Electron Microscopy (FESEM), Brunauer–Emmett–Teller (BET), and Transmission Electron Microscopy (TEM). The Powder X-ray diffraction (PXRD) revealed that synthesized ZnO (L5) and ZnO (L10) consist of a typical hexagonal wurtzite phase. The size and morphology of synthesized ZnO (L5) and ZnO (L10) nanoparticles were determined using Scanning Electron Microscopy (SEM), Field Emission Scanning Electron Microscopy (FESEM), and Transmission Electron Microscopy (TEM). The FTIR study reveals that phytochemicals from the leaf of the Madagascar periwinkle leaf extract are present on the surface of synthesized ZnO (L5) and ZnO (L10) nanoparticles. The BET analysis shows that the measured surface areas of ZnO (L5) and ZnO (L10) nanoparticles are 10.202 m 2 /g and 38.762 m 2 /g respectively. The photocatalytic activity of both the synthesized nanoparticles was determined against methylene blue (MB) organic dye. It was evident from the results that ZnO (L10) is a better catalyst than ZnO (L5). Herein, MB dye was degraded (94.09%) in 600 min by ZnO (L5) photocatalyst and (97.92%) in 360 min by ZnO (L10) photocatalyst. Therefore synthesized ZnO (L5) and ZnO (L10) nanoparticles could be employed as an efficient photocatalyst for the degradation of toxic organic dyes.
Graphical Abstract
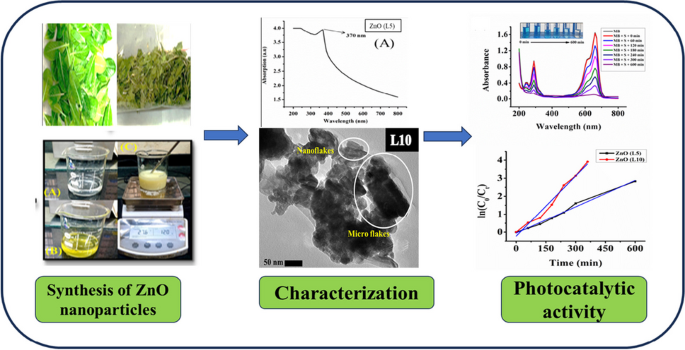
This is a preview of subscription content, log in via an institution to check access.
Access this article
Price includes VAT (Russian Federation)
Instant access to the full article PDF.
Rent this article via DeepDyve
Institutional subscriptions

Similar content being viewed by others
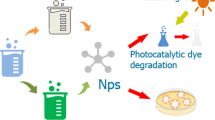
Synthesis of TiO2 nanoparticles by chemical and green synthesis methods and their multifaceted properties
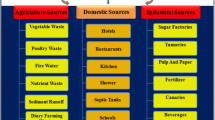
A Review on Photocatalysis Used For Wastewater Treatment: Dye Degradation
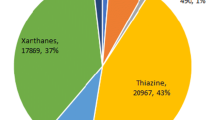
Photocatalysis: an effective tool for photodegradation of dyes—a review
Data availability.
Not applicable.
Abbreviations
X-Ray Diffraction
Scanning Electron Microscopy
Energy Dispersive Spectroscopy
Ultraviolet
Fourier Transform Infrared Spectroscopy
Field Emission Scanning Electron Microscopy
Brunauer-Emmett-Teller
Transmission Electron Microscopy
Powder X-Ray Diffraction
Methylene blue
Leaf extract
Advanced Oxidation Processes
Nanoparticles
Reduced graphene oxide
ZnO nanoparticle synthesized using 5 mL of Madagascar Periwinkle plant’s leaf extract
Subki NS (2017) Environmental contamination by batik wastewater and the potential application of activated carbon from pineapple waste for wastewater treatment. Ph. D. Thesis, University Sains Malaysia, Kubang Kerian, Kelantan, Malaysia
Patel H, Yadav VK, Yadav KK, Choudhary N, Kalasariya H, Alam MM, Gacem A, Amanullah M, Ibrahium HA, Park JW, Park S, Jeon BH (2022) A Recent and Systemic Approach Towards Microbial Biodegradation of Dyes from Textile Industries. Water 14:3163. https://doi.org/10.3390/w14193163
Article Google Scholar
Kaushal S, Kurichh P, Singh PP (2021) Novel 3D fower like ZnO/ MnV 2 O 6 heterojunction as an efficient adsorbent for the removal of imidacloprid and photocatalyst for degradation of organic dyes in waste water. Polyhedron 201:115161. https://doi.org/10.1016/j.poly.2021.115161
Zhang X, Yin Y, Sun Z, Du Y, Ma S, Wu Y (2016) Cr/S/TiO 2 - loaded hollow glass microspheres as an efficient and recyclable catalyst for the photocatalytic degradation of indigo carmine under visible light. Quim. Nova 39:956−961. https://doi.org/10.21577/0100-4042.20160121
Gao Y, Wang T (2021) Preparation of Ag 2 O/TiO 2 nanocomposites by two-step method and study of its degradation of RhB. J Mol Struct 1224:129049. https://doi.org/10.1016/j.molstruc.2020.129049
Mousa HM, Alenezi JF, Mohamed IMA, Yasin AS, Hashem AFM, Abdal-hay A (2021) Synthesis of TiO 2 @ZnO heterojunction for dye photodegradation and wastewater treatment. J Alloys Compd 886:161169. https://doi.org/10.1016/j.jallcom.2021.161169
Wu Y, Pang H, Liu Y, Wang X, Yu S, Fu D, Chen J, Wang X (2019) Environmental remediation of heavy metal ions by novel-nanomaterials: A review. Environ Pollut 246:608–620. https://doi.org/10.1016/j.envpol.2018.12.076
Ghanbari F, Zirrahi F, Lin KYA, Kakavandi B, Hassani A (2020) Enhanced electro-peroxone using ultrasound irradiation for the degradation of organic compounds: a comparative study. J Environ Chem Eng 8:104167. https://doi.org/10.1016/j.jece.2020.104167
Yadav S, Rani N, Saini K (2022) A review on transition metal oxides based nanocomposites, their synthesis techniques, diferent morphologies and potential applications. IOP Conf Ser: Mater Sci Eng. 1225:012004. https://doi.org/10.1088/1757-899X/1225/1/012004
Flilissa A, Laouameur K, HammoudI NE, Tamam N, Yadav KK, Achouri B, Alyami AY, Flilissa O, Algethami JS, Abbasj M, Jeon BH, Benboudiaf S, Benguerba Y (2024) Bentonite SDBS-loaded composite for methylene blue removal from wastewater: An experimental and theoretical investigation. Environ Res 241:117544. https://doi.org/10.1016/j.envres.2023.117544
Utami M, Wang S, Musawwa MM, Purbaningtias TE, Fitri M, Yuspita I, Abd-Elkader OH, Yadav KK, Ramanujam GM, Bang D, Chang SW, Ravindran B (2023) Simultaneous photocatalytic removal of organic dye and heavy metal from textile wastewater over N-doped TiO 2 on reduced graphene oxide. Chemosphere 332:138882. https://doi.org/10.1016/j.chemosphere.2023.138882
Yadav VK, Yadav KK, Gacem A, Gnanamoorthy G, Ali IH, Khan SH, Jeon BH, Kamyab H, Inwati GK, Choudhary N, Islam S, Cabral-Pinto MMS (2022) A novel approach for the synthesis of vaterite and calcite from incense sticks ash waste and their potential for remediation of dyes from aqueous solution. Sustain Chem Pharm 29:100756. https://doi.org/10.1016/j.scp.2022.100756
Naik HS, Sah PM, Dhangade M, Lakkakula J, Raut RW, Roy A, Alghamdi S, Qusty N, Alhindi Z, Kabrah A, Rani A (2023) Synthesis of a silica matrix with ZnO nanoparticles for the fabrication of a recyclable photodegradation system to eliminate methylene blue dye. Green Process Synth 12:20230157. https://doi.org/10.1515/gps-2023-0157
Fetimi A, Merouani S, Khan MS, Asghar MN, Yadav KK, Jeon BH, Hamachi M, Senhadji OK, Benguerba Y (2022) Modeling of Textile Dye Removal from Wastewater Using Innovative Oxidation Technologies (Fe(II)/Chlorine and H 2 O 2 /Periodate Processes): Artificial Neural Network-Particle Swarm Optimization Hybrid Model. ACS Omega 7:13818–13825. https://doi.org/10.1021/acsomega.2c00074
Singhal N, Selvaraj S, Sivalingam Y, Venugopal G (2022) Study of photocatalytic degradation efficiency of rGO/ZnO nano-photocatalyst and their performance analysis using scanning Kelvin probe. J Environ Chem Eng 10:107293. https://doi.org/10.1016/j.jece.2022.107293
Karim AV, Aydin Hassani A, Eghbali P, Nidheesh PV (2022) Nano-structured modified layered double hydroxides (LDHs)-based catalysts: a review on synthesis, characterization, and applications in water remediation by advanced oxidation processes. Curr Opin Solid State Mater Sci 26:100965. https://doi.org/10.1016/j.ossms.2021.100965
Kumari V, Yadav S, Jindal J, Sharma S, Kumari K, Kumar N (2020) Synthesis and characterization of heterogeneous ZnO/CuO hierarchical nanostructures for photocatalytic degradation of organic pollutant. Adv Powder Technol. 31:2658–2668. https://doi.org/10.1016/j.apt.2020.04.033
Giannakis S, Rtimi S, Pulgarin C (2017) Light-assisted advanced oxidation processes for the elimination of chemical and microbiological pollution of wastewaters in developed and developing countries. Molecules 22:1070. https://doi.org/10.3390/molecules22071070
Aminuzzaman M, Chong CY, Goh WS, Phang YK, Hock TL, Chee SY, Akhtaruzzaman M, Ogawa S, Watanabe A (2021) Biosynthesis of NiO Nanoparticles Using Soursop ( Annona muricata L. ) Fruit Peel Green Waste and Their Photocatalytic Performance on Crystal Violet Dye. J Clust Sci 32:949–958. https://doi.org/10.1007/s10876-020-01859-8
Pandit C, Roy A, Ghotekar S, Khusro A, Islam MN, Emran TB, Lam SE, Khandaker MU, Bradley DA (2022) Biological agents for synthesis of nanoparticles and their applications. J King Saud Univ Sci 34:101869. https://doi.org/10.1016/j.jksus.2022.101869
Noor F, Mahmood A, Zafar N, Sarfraz RM, Rehman U, Ijaz H, Hussain Z, Ahmed IA, Imam MT, Abdulmonem WA, Yadav KK, Benguerba Y (2023) Fabrication of pH-responsive hydrogels of perindopril erbumine using black seed extract and β-cyclodextrin co-polymerized with methacrylic acid and methylene bisacrylamide. J Drug Deliv Sci Techno 88:104924. https://doi.org/10.1016/j.jddst.2023.104924
Idris DS, Roy A (2024) Biogenic Synthesis of Ag–CuO Nanoparticles and Its Antibacterial, Antioxidant, and Catalytic Activity. J Inorg Organomet Polym Mater 34:1055–1067. https://doi.org/10.1007/s10904-023-02873-9
Bhusal U, Roy A, Kunwar S (20230 Bio-fabrication of Cu/Fe/Zn nanoparticles and its antioxidant and catalytic activity. Chem Pap. 77 :7099–7111. https://doi.org/10.1007/s11696-023-03001-0
Idris DS, Roy A, Subramanian A, Alghamdi S, Chidamabaram K, Qusty NF (2023) Bio-fabrication of Silver-Zinc Bimetallic Nanoparticles and Its Antibacterial and Dye Degradation Activity. J Inorg Organomet Polym Mater. https://doi.org/10.1007/s10904-023-02936-x
Di T, Xu Q, Ho W, Tang H, Xiang Q, Yu J (2019) Review on Metal Sulphide-based Z-scheme Photocatalysts. Chem Cat Chem 11:1394–1411. https://doi.org/10.1002/cctc.201802024
Mills A, Davies R, H, Worsley D, (1993) Water purification by semiconductor photocatalysis. Chem Soc Rev 22:417–425. https://doi.org/10.1039/CS9932200417
Waghchaure RH, Adole VA, Jagdale BS, Koli PB (2022) Fe3+ modifed zinc oxide nanomaterial as an efficient, multifaceted material for photocatalytic degradation of MB dye and ethanol gas sensor as part of environmental rectifcation. Inorg Chem Commun 140:109450. https://doi.org/10.1016/j.inoche.2022.109450
Golmohammadi M, Honarmand M, Ghanbari S (2020) A green approach to synthesis of ZnO nanoparticles using jujube fruit extract and their application in photocatalytic degradation of organic dyes. Spectrochim Acta A Mol Biomol Spectrosc 229:117961. https://doi.org/10.1016/j.saa.2019.117961
Wetchakun K, Wetchakun N, Sakulsermsuk S (2019) An overview of solar/visible light-driven heterogeneous photocatalysis for water purification: TiO 2 - and ZnO-based photocatalysts used in suspension photoreactors. J Ind Eng Chem 71:19–49. https://doi.org/10.1016/j.jiec.2018.11.025
Gu X, Li C, Yuan S, Ma M, Qiang Y, Zhu J (2016) ZnO based heterojunctions and their application in environmental photocatalysis. Nanotechnology 27:402001. https://doi.org/10.1088/0957-4484/27/40/402001
Modi S, Yadav VK, Gacem A, Ali IH, Dave D, Khan SH, Yadav KK, Rather S, Ahn Y, Son CT, Jeon BH (2022) Recent and Emerging Trends in Remediation of Methylene Blue Dye from Wastewater by Using Zinc Oxide Nanoparticles. Water 14:1749. https://doi.org/10.3390/w14111749
Pham HNT, Vuong QV, Bowyer MC, Scarlett CJ (2020) Phytochemicals Derived from Catharanthus roseus and Their Health Benefits. Technologies 8(4):80. https://doi.org/10.3390/technologies8040080
Yadav S, Rani N, Saini K (2022) Green synthesis of ZnO and CuO NPs using Ficus benghalensis leaf extract and their comparative study for electrode materials for high performance supercapacitor application. Mater Today: Proc. 49:2124–2130. https://doi.org/10.1016/j.matpr.2021.08.323
Rahman QI, Ahmad M, Misra SK, Lohani M (2013) Efective photocatalytic degradation of rhodamine B dye by ZnO nanoparticles. Mater Lett 91:170–174. https://doi.org/10.1016/j.matlet.2012.09.044
Su NR, Lv P, Li M, Zhang X, Li M, Niu J (2014) Fabrication of MgFe 2 O 4 -ZnO heterojunction photocatalysts for application of organic pollutants. Mater Lett 122:201–204. https://doi.org/10.1016/j.matlet.2013.12.106
Li JF, Rupa EJ, Hurh J, Huo Y, Chen L, Han Y, Ahn JC, Park JK, Lee HA, Mathiyalagan R, Yang DC (2019) Cordyceps militaris fungus mediated zinc oxide nanoparticles for the photocatalytic degradation of Methylene blue dye. Optik 183(691):697. https://doi.org/10.1016/j.ijleo.2019.02.081
Mahajan P, Singh A, Arya S (2020) Improved performance of solution processed organic solar cells with an additive layer of sol-gel synthesized ZnO/CuO core/shell nanoparticles. J Alloys Compd 814:152292. https://doi.org/10.1016/j.jallcom.2019.152292
Yadav S, Yadav J, Kumar M, Saini K (2022) Synthesis and characterization of nickel oxide/cobalt oxide nanocomposite for effective degradation of methylene blue and their comparative electrochemical study as electrode material for supercapacitor application. Int J Hydrog Energy 47:41684–41697. https://doi.org/10.1016/j.ijhydene.2022.02.011
Yadav S, Rani N, Saini K (2023) Coupling ZnO with CuO for efcient organic pollutant removal. Environ Sci Pollut Res 30:71984–72008. https://doi.org/10.1007/s11356-022-24139-6
Rani N, Yadav S, Mushtaq A, Rani S, Saini M, Rawat S, Gupta K, Saini K, Maity D (2024) Azadirachta indica peel extract-mediated synthesis of ZnO nanoparticles for antimicrobial, supercapacitor and photocatalytic applications. Chem Pap. https://doi.org/10.1007/s11696-024-03340-6
Miri A, Vahed HOS, Sarani M (2018) Biosynthesis of silver nanoparticles and their role in photocatalytic degradation of methylene blue dye. Res Chem Intermed. 44:6907–6915. https://doi.org/10.1007/s11164-018-3529-3
Ullah R, Dutta J (2008) Photocatalytic degradation of organic dyes with manganese-doped ZnO nanoparticles. J. Hazard. Mater. 156:194–200. https://doi.org/10.1016/j.jhazmat.2007.12.033
Saleh R, Djaja NF (2014) UV light photocatalytic degradation of organic dyes with Fe-doped ZnO nanoparticles. Superlattices Microstruct. 74:217–233. https://doi.org/10.1016/j.spmi.2014.06.013
Devi LV, Sellaiyan S, Selvalakshmi T, Zhang HJ, Uedono A, Sivaji K, Sankar S (2017) Synthesis, defect characterization and photocatalytic degradation efficiency of Tb doped CuO nanoparticles. Adv Powder Technol. 28:3026–3038. https://doi.org/10.1016/j.apt.2017.09.013
Download references
Acknowledgements
The authors heartfully acknowledge Principal, Miranda House, University of Delhi for providing the lab facility. The authors want to thanks to USIC, University of Delhi for providing instrumental facilities.
Sapna Yadav is thankful to CSIR, New Delhi for SRF (CSIR, File No. 08/700(0004)/2019-EMR-I).
Author information
Authors and affiliations.
Department of Chemistry, Gurugram University, Haryana, 122003, Gurugram, India
Akansha Gupta
Department of Chemistry, Miranda House, University of Delhi, Patel Chest Marg, Delhi, 110007, India
Sapna Yadav, Nutan Rani & Kalawati Saini
Department of Chemistry, Raj Rishi Government College, Alwar, 301001, Rajasthan, India
Kalpna Gupta
You can also search for this author in PubMed Google Scholar
Contributions
Conceptualization, methodology, writing-original draft preparation, Akansha Gupta, Sapna Yadav, and Nutan Rani; supervision and editing, Kalpna Gupta and Kalawati Saini.
Corresponding author
Correspondence to Kalawati Saini .
Ethics declarations
Ethics approval, consent to participate.
All the authors consented to participate in the drafting of this research article.
Consent for publication
All of the authors consented to publish this research article.
Conflict of interest
The authors declare no conflict of interest.
Additional information
Publisher's note.
Springer Nature remains neutral with regard to jurisdictional claims in published maps and institutional affiliations.
Rights and permissions
Springer Nature or its licensor (e.g. a society or other partner) holds exclusive rights to this article under a publishing agreement with the author(s) or other rightsholder(s); author self-archiving of the accepted manuscript version of this article is solely governed by the terms of such publishing agreement and applicable law.
Reprints and permissions
About this article
Gupta, A., Yadav, S., Rani, N. et al. Degradation of toxic methylene blue dye with Zinc oxide (ZnO) nanoparticles synthesized by leaf extract of Madagascar periwinkle plant. Biomass Conv. Bioref. (2024). https://doi.org/10.1007/s13399-024-05711-1
Download citation
Received : 22 January 2024
Revised : 11 April 2024
Accepted : 29 April 2024
Published : 06 May 2024
DOI : https://doi.org/10.1007/s13399-024-05711-1
Share this article
Anyone you share the following link with will be able to read this content:
Sorry, a shareable link is not currently available for this article.
Provided by the Springer Nature SharedIt content-sharing initiative
- Biosynthesis
- ZnO (L5) nanoparticles
- ZnO (L10) nanoparticles
- Madagascar periwinkle
- Photocatalytic degradation
- Organic dye
- Find a journal
- Publish with us
- Track your research
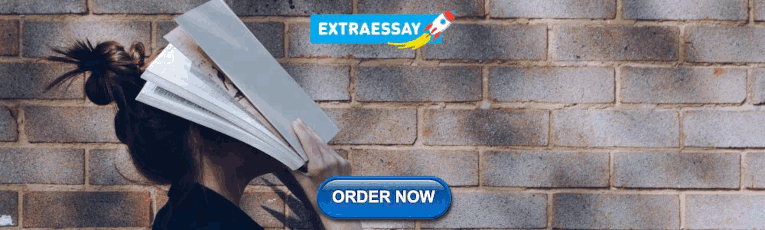
IMAGES
VIDEO
COMMENTS
Zinc oxide nanoparticles are categorized. among the materials that have potential. applications in many a reas of nanotechnology. [29, 30]. Z nO possesses one-, two- and three-. dimensional str uc ...
Zinc oxide nanoparticles (ZnO-NPs) are the most commonly used metal oxide nanoparticles because their distinctive optical and chemical properties can be easily modified by altering the morphology and the wide bandgap (3.37 eV) and high excitation binding energy (60 meV) to simulate the ZnO-NPs to be a potent photocatalytic and photo-oxidizing ...
Fig. 4, Fig. 5 clearly shows that the zinc oxide nanoparticles obtained by refluxing diethylene glycol and triethylene glycol for 2 h and 3 h in presence and in absence of sodium acetate have uniform shape and size with different morphology. Image depicts addition of sodium acetate, use of different polyol and change in reflux time from 2 h to ...
Band gap energy of zinc oxide nanoparticle at 500 rpm was 3.50 eV. ZnO.NPs FTIR spectrum was recorded in the range of 4000-500 cm−1. ... Young M (2016) Synthesis of zinc oxide nanoparticles with different morphology by wet chemistry routes Doctoral Thesis submitted in partial fulfilment of the requirements for the award of doctor of ...
In recent years, zinc oxide nanoparticles (ZnONPs) emerged as an excellent candidate in the field of optical, electrical, food packaging and particularly in biomedical research. ZnONPs show cancer cell specific toxicity via the pH-dependent (low pH) dissolution into Zn 2+ ions, which generate reactive oxygen species and induce cytotoxicity in ...
This is to certify that the thesis entitled, "Synthesis and characterisation of ZnO nanoparticles ... In the present research work, synthesis of zinc nanoparticles and its characterization was done. In this study, zinc nanoparticles were rapidly synthesized from Zn(NO3)2 solution ... Zinc oxide, ZnO is an inorganic compound also known as ...
In this work, two different methods (sol-gel and biosynthesis) were adopted for the synthesis of zinc oxide (ZnO) nanoparticles. The leaf extract of Azadirachta Indica (Neem) was utilized in the biosynthesis scheme. Structural, antibacterial, photocatalytic and optical performances of the two variants were analyzed. Both variants demonstrated a ...
Synthesis of zinc oxide nanoparticles by a green process and the investigation of their physical properties . Takalani Nethavhanani . A thesis submitted in partial fulfilment of the requirements for the degree of Magister Scientiae in the Department of Physics, University of the Western Cape . Supervisor: Prof M Maaza, iThemba LABS
Zinc Oxide is a transition metal oxide semiconductor with wide bandgap and high exciton binding energy. Hence ZnO is one of the most preferable materials in optoelectronic research field [10-18]. It is a low-cost material and easily available in nature . ZnO nanoparticles are transparent to visible part of light and absorbing UV radiations.
Biosynthesis and characterization of ZnO nanoparticles. The synthesis of biosynthesized ZnO NPs was carried out by taking 2:2 (v/v) of leaves extract of and zinc nitrate solution to obtain a ...
Nanoparticle (NP) research has gained distinct interest due to the enhanced electrochemical reactivity, thermal conductivity, and nonlinear optical properties of nanoparticles which offer unique applications [2]. Zinc oxide nanoparticles (ZnO-NPs) are the most commonly used metal oxide nanoparticles because their distinctive optical
Zinc nanomaterials, particularly zinc oxide NPs (ZnONPs), are getting prominence as revolutionary medicinal treatments. Due to their biocompatible and non-toxic nature, zinc oxide nanoparticles are listed as "generally recognized as safe" (GRAS) by the U.S. FDA. ZnO is an n-type semiconductor with a wide band gap of 3.4 eV at room temperature and relatively large exciting binding energy of ...
Rajiv, P., Rajeshwari, S. & Venckatesh, R. Bio-fabrication of zinc oxide nanoparticles using leaf extract of Parthenium hysterophorus L. and its size-dependent antifungal activity against plant ...
Zinc oxide nanoparticle synthesis by using different sources. 2.1. Green synthesis of ZnO NPs using plant extract. Plant parts like leaf, stem, root, fruit, and seed have been used for ZnO NPs synthesis because of the exclusive phytochemicals that they produce. Using natural extracts of plant parts is a very eco-friendly, cheap process and it ...
In recent years, zinc oxide nanoparticles (ZnONPs) emerged as an excellent candidate in the field of optical, electrical, food packaging and particularly in biomedical research. ZnONPs show cancer cell specific toxicity via the pH-dependent (low pH) dissolution into Zn 2+ ions, which generate reactive oxygen species and induce cytotoxicity in ...
Through this study, zinc oxide nanoparticles (ZnO.NPs) have been synthesized based on using the environmentally benign extract of the aerial parts of D. tortuosa as a reducing and capping agent.
2.1. Nanoparticles Characterization Results. The UV-Vis spectrophotometer was used to characterize the structural properties of the NPs by determining the absorbance, confirming the formation of previous findings and verifying that the K. blossfeldiana extract yielded a stable ZnO NP synthesis. Figure 1 shows the UV-Vis absorption spectra of the ZnO NPs observed at 200-600 nm.
Mechanism of formation of zinc oxide nanoparticles via green synthesis. Although many studies indicate the effectiveness of the green synthesis for the production of metal and metal oxides nanoparticles, this process has only been demonstrated at a laboratory scale (Agarwal et al., 2017; Kharissova et al., 2013; Mirzaei and Darroudi, 2016 ...
The undersigned, appointed by the dean of the Graduate School, have examined the thesis entitled . CHARACTERIZATION OF ZINC OXIDE NANOPARTICLES AND THEIR APPLICATIONS IN FOOD SAFETY . Presented by RUOYU LI A candidate for the degree of Master of Science And hereby certify that, in their opinion, it is worthyceptance.of ac .
Solubility of zinc oxide (1.6-5.0 mg/L) in aqueous medium is higher than that of zinc oxide nanoparticles (0.3-3.6 mg/L) in the same medium [] which is toxic to algae and crustaceans.Both nano-zinc oxide and bulk zinc oxide are 40-80-fold less toxic than ZnSO 4 against V. fischeri.The higher antibacterial activity of ZnSO 4 is directly proportional to its solubility releasing Zn 2+ ions ...
The current study aimed to find an effective, simple, ecological, and nontoxic method for bacterial green synthesis of zinc oxide nanoparticles (ZnONPs) using the bacterial strain Priestia ...
We have coated onto silicone-based urinary catheters an organo-soluble antimicrobial polymer nanocomposite (APN), containing hydrophobic quaternized polyethyleneimine and zinc oxide nanoparticles, in a single step coating process.
The elemental composition of zinc oxide nanoparticles was analyzed using inbuilt energy dispersive X-ray spectroscopy (EDS). The optical properties of synthesized nanoparticles were analyzed through UV-visible spectroscopy (Spectramax M2e UV-visible spectrophotometer). ... Thesis, University Sains Malaysia, Kubang Kerian, Kelantan, Malaysia.